- Academic Editor
Background: The interaction between oxidative status markers and
biological rhythms is considered particularly important in the pathogenesis of
many diseases and more effective therapies. We aimed to determine if the salivary
secretion of myeloperoxidase exhibits diurnal variations, and if the potential
daily variability differs seasonally. Methods: The study was performed
in Poznan, Poland (52,25°N, 16,58°E) in 10 healthy male
volunteers (age median 23.5 years). Whole mixed unstimulated saliva was collected
in summer (August) and winter (December) during 36 h at 2-h intervals starting at
6 a.m. on Saturday and ending at 6 p.m. on Sunday, in the domestic setting. The
samples were analysed for myeloperoxidase (MPO) and cortisol by immunoassays. The
presence of the circadian rhythm of cortisol secretion in saliva confirmed the
rhythmicity of the volunteers. Results: For salivary MPO, significantly
higher concentrations compared to midnight and noon were observed for 4 a.m. in
both summer and winter. Using the cosinor analysis, the variations in salivary
MPO levels showed a moderate fit for the 12-h period rhythm (acrophases: in
summer 05:37/17:37, in winter 06:16/18:16), without significant differences in
the rhythm parameters in summer and winter. However, higher self-reported Global
Seasonal Score (which may predispose to seasonal affective disorder) was
associated with significantly stronger relative amplitude (R
Biological rhythms are repetitive natural phenomena depending on the time of day or year. Most human life processes have a circadian rhythm lasting about 24 hours, such as cortisol secretion. Shorter rhythms are called ultradian rhythms, while infradian rhythms are endogenous rhythms with longer than 28-hour periods; the seasonal cycle is the best-known example [1]. The light/dark rotation is the strongest zeitgeber responsible for the synchronisation of rhythms [2]. The light impulses from the retina reach the suprachiasmatic nucleus (SCN) in the hypothalamus region, which is found to be the primary regulator of endogenous rhythms (“body clock”) [3].
Therefore, the human body clock is susceptible to seasonal variations in the natural light-dark cycle, leading to the expanded biological night in winter compared with summer [4]. Seasonal rhythms influenced by environmental changes are commonly described in mammals [5]. In humans, seasonal variations in diurnal rhythms may be affected by various exogenous factors, such as dietary patterns, physical activity, ambient temperature, and natural light exposure [6]. Honma et al. [7] indicated that the circadian rhythms of the individual biological parameters (such as the timing of sleep or the rectal temperature) might also depend on seasons.
In addition, seasonal variations in mood and behaviour refer to depressive
symptoms caused by seasonal changes [8]. Seasonal affective disorder (SAD) is a
recurrent seasonal condition with depressive episodes commonly in autumn or
winter and remissions in spring or summer. The prevalence is estimated to be from
1.5% to 9% worldwide [9]. The potential risk factors include family history,
female sex, living in more northern latitudes, and young adult age (
Myeloperoxidase (MPO) is a heme peroxidase expressed primarily in neutrophils and monocytes. It is one of the most important enzymes responsible for local immunity and host defence [20, 21]. MPO catalyses the formation of reactive species (including hypochlorous acid) involved in microbial killing [22, 23]. Due to the potentially defensive role, MPO is increased in conditions associated with oxidative stress and inflammation [24, 25]. For instance, we found that salivary MPO could predict differential diagnosis and treatment effectiveness in inflammatory bowel disease patients [21, 26], but it may be affected by daily secretion variability. In addition, circadian variations in MPO concentrations might accompany some other time-dependent events, such as atherosclerotic plaque destabilisation, which plays a role in the occurrence of CVDs [27, 28]. MPO is elevated in patients with acute coronary syndrome [29], which is found to be associated with seasonality [30].
However, no study has assessed the direct association of MPO with SAD. Galijasevic et al. [31] presented that MPO could be inhibited by melatonin under physiological-like conditions as well as during inflammation. Interestingly, the melatonin-MPO interactions may be bidirectional, and MPO may act as a chelator for melatonin, limiting its bioavailability. Therefore, these interactions could have biological implications for wake-sleep rhythmicity regulation. The authors speculated that increased inflammation and decreased immunity caused by chronic sleep loss could result in the insufficient inhibitory protective effects of melatonin on MPO during prolonged waking hours.
Moreover, many antioxidative enzymes manifested diurnal rhythmicity in several organisms and tissues, but the detailed rhythm parameters, such as amplitudes or acrophases, were significantly divergent [32]. Understanding the interaction between oxidative status and diurnal, seasonal rhythms might be essential to understand better the pathogenesis of some inflammatory diseases (including coronary artery diseases) and undertake a more effective therapy considering both components [33].
To our knowledge, the daily and seasonal variations of MPO secretion have not been evaluated. In Poland, the seasonal daylight fluctuations are intense, and thus, the Polish population is a good subject for studying seasonality. The salivary cortisol secretion was assessed as a marker of diurnal and seasonal rhythm. Cortisol secretion in the saliva is characterised by a circadian rhythm with higher values in the morning than in the evening [34] and with seasonal changes [35, 36].
Our study aimed to determine if the salivary secretion of MPO exhibits diurnal variations in healthy men, if the daily variability differs seasonally between summer and winter, and whether SAD could affect MPO rhythms.
The study protocol followed the guidelines for biological rhythm research on human beings by Portaluppi et al. [37], was approved by the Bioethics Committee of the Poznan University of Medical Sciences (223/19), and followed the principles of the Declaration of Helsinki [38]. All participants gave written informed consent.
The study group consisted of 10 healthy male volunteers recruited from Poznan University of Medical Sciences. The detailed characteristics of the participants are presented in Table 1.
Parameter | |
Age, years | 23.5 (21.0–26.0) |
Body mass index, kg/m |
20.3 (19.6–21.1) |
Global seasonal score | 10.5 (8.0–14.0) |
Seasonal affective disorder, n (%) | 5 (50) |
Chronotype, n (%): | |
“lark” | 2 (20) |
“owl” | 5 (50) |
“neither” | 3 (30) |
All of them demonstrated normal values of body mass index. The subjects had no history of systemic disease, current or past psychiatric disorders, and long-term use of medications, including steroids. In each session, one week before laboratory admission, participants were asked to abstain from excessive physical activity, alcohol, and caffeine consumption. During this week, they were also enquired to keep regular sleep-wake rhythms (i.e., sleep duration of 8 h at night within a regular bedtime) to ensure proper circadian entrainment between the sleep-wake and light-dark cycle.
We excluded volunteers with sleep disorders (self-assessed according to the Pittsburgh Sleep Quality Index [39]) and habitual sleep duration of less than six or more than nine hours. Further exclusion criteria included shift workers and participants who had transmeridian flights in the three previous months. Also, smokers and those taking antioxidants or other supplements with redox properties were excluded. Based on the dental examination (conducted by K.N.), the presence of overt inflammatory lesions in the oral cavity was excluded.
During the eligibility screening, the participants were asked to fill in the
survey on their habits regarding sleep (retiring and rising time, sleep length,
sleep quality, refreshed after sleep), food intake (number of courses for each
meal) and physical activity (in hours per day: lying, sitting, walking, exercise
and standing) [40], as well as the Seasonal Pattern Assessment Questionnaire
[41]. According to the criteria by Kasper et al. [42], self-assessed
seasonal affective disorder (SAD) was defined by the global seasonal score (GSS)
The study was conducted in two sessions—summer (August) and winter (December)—in Poznań (west-central Poland; 52,25°N, 16,58°E). The meteorological data for these study periods were obtained from the National Institute of Meteorology and Water Management (Table 2).
Parameter | Summer | Winter | ||
1st day | 2nd day | 3rd day | 4th day | |
Sunrise time | 5:20 | 5:22 | 7:42 | 7:44 |
Sunset time | 20:35 | 20:33 | 15:41 | 15:39 |
Photoperiod, h | 15.25 | 15.18 | 7.98 | 7.92 |
Sunshine duration, h* | 5.3 | 10.3 | 0.0 | 0.0 |
Relative humidity, % | 59.0 | 45.0 | 94.0 | 97.0 |
Median temperature, °C | 19.8 | 19.3 | –1.7 | 2.0 |
Minimal temperature, °C | 15.3 (23:00) | 9.1 (5:00) | –3.1 (2:00) | 0.1 (0:00) |
Maximal temperature, °C | 23.1 (18:00) | 24.4 (15:00) | 0.0 (23:00) | 2.7 (13:00) |
*sunshine above 120 W/m
Unstimulated whole mixed saliva was collected using Salivette (Sarstedt,
Nümbrecht, Germany), according to the manufacturer’s instructions. The
absorbent rolls were kept in the mouth for 5 minutes without chewing (if an
extremely small of saliva was produced, the swabs were left longer). During each
session, in the summer and winter seasons, the samples were collected during 36 h
at 2-h intervals starting at 6 a.m. on Saturday and ending at 6 p.m. on Sunday,
in the domestic setting. One hour before sampling, participants were asked not to
eat, drink (except water) or brush their teeth. Sleeping was allowed at night.
The samples were refrigerated and then, after centrifugation (1000
Since the questionnaire data did not follow the normal distribution (as determined by the Shapiro–Wilk test), medians and quartile ranges were used for descriptive statistics. We compared these variables using the non-parametric Mann–Whitney test (SAD vs non-SAD subjects) and Wilcoxon test (summer vs winter). The relationship between variables was analysed with Spearman’s rank correlation. The determined salivary concentrations of MPO and cortisol were analysed using a two-way repeated-measures analysis of variance in the model with the interaction. The variables were compared separately at specific points in time depending on the season using LSD post-hoc test. The statistical analysis was performed using Statistica 13.3 (Statsoft, Cracow, Poland). The significance level for all analyses was set at 0.05.
The daily rhythms were assessed by the cosinor analysis using packages cosinor and cosinor2 in RStudio 1.2.5033 (RStudio Inc., Boston, MA, USA). According to the procedure described by Cornelissen [44], to calculate population-mean cosinor, single cosinors were first performed on each subject, and linearised parameters are averaged, which allows for the calculation of delinearised parameters. After such a procedure was completed, confidence intervals of population-mean cosinor parameters were calculated as described by Bingham et al. [45]. The cosinor parameters, such as MESORs (midline estimating statistic of rhythm - mean of all values across the circadian rhythm), amplitudes (half the predictable extent of change within a day) and acrophases (timepoint of overall peak values recurring each day), were compared for populations as described by Bingham et al. [45]. The rhythm detection test (also called the zero-amplitude test) was performed to assess the overall significance of the cosinor models, as described by Cornelissen [44]. The relative strength of rhythms was evaluated by Percent Rhythm, the coefficient of determination obtained by squaring the correlation between observed and estimated values.
As previously reported in Table 1, half of the volunteers met the criteria for seasonal affective disorder (SAD). In terms of chronotype, 5 participants were “owls”, 2 “larks”, and 3 did not fit into either group.
Participants did not differ significantly in terms of sleep parameters, activity and eating habits between summer and winter (Table 3). Also, the significant differences between SAD and non-SAD subjects in these reported parameters were not found in either summer or winter (data not presented). Only SAD participants declared more courses consumed for dinner in winter than in summer, at borderline significance (p-value = 0.068).
Summer | Winter | Comparison | ||
Sleep parameters | ||||
Retiring time | 00:15 (23:00–00:30) | 00:30 (23:30–00:30) | ns | |
Rising time | 09:30 (8:30–10:00) | 09:15 (7:30–9:30) | ns | |
Sleep length, h | 9.0 (8.5–10.0) | 8.5 (8.5–9.0) | ns | |
Sleep quality | 3.0 (2.5–3.5) | 3.0 (3.0–3.5) | ns | |
Refreshed after sleep | 3.5 (3.0–4.0) | 3.5 (3.0–3.5) | ns | |
Activity, h per day | ||||
Lying | 7.5 (5.0–8.0) | 6.0 (5.0–8.5) | ns | |
Sitting | 7.5 (5.5–9.0) | 7.5 (6.0–8.0) | ns | |
Walking | 2.0 (1.5–2.5) | 2.0 (1.0–2.5) | ns | |
Exercise | 0.5 (0.0–1.0) | 0.5 (0.0–1.0) | ns | |
Standing | 2.0 (1.0–2.5) | 1.5 (1.0–2.0) | ns | |
Meals | ||||
Breakfast | 1.0 (1.0–1.0) | 1.0 (1.0–1.0) | ns | |
Lunch | 0.5 (0.0–0.5) | 1.0 (0.0–1.0) | ns | |
Dinner | 1.0 (1.0–1.5) | 1.5 (1.0–2.0) | ns | |
Tea | 1.0 (0.5–1.0) | 0.5 (0.0–1.0) | ns | |
Supper | 1.0 (1.0–1.0) | 1.0 (1.0–1.0) | ns |
Sleep quality and refreshed after sleep were scored 0–5 (0—the lowest, 5—the highest). Meals were scored as followed: 0—nothing eaten, 1—snack/one-course meal, 2—two-course meal, and 3—three-course meal or more. ns, not significant.
Figs. 1,2 present the daily changes in the salivary concentrations of MPO and cortisol. We observed statistically significant changes in the levels of both markers in saliva during the day (Supplementary Tables 1,2). However, no significant differences were found for individual time points in summer and winter for the salivary concentrations of both MPO and cortisol (Supplementary Table 3).
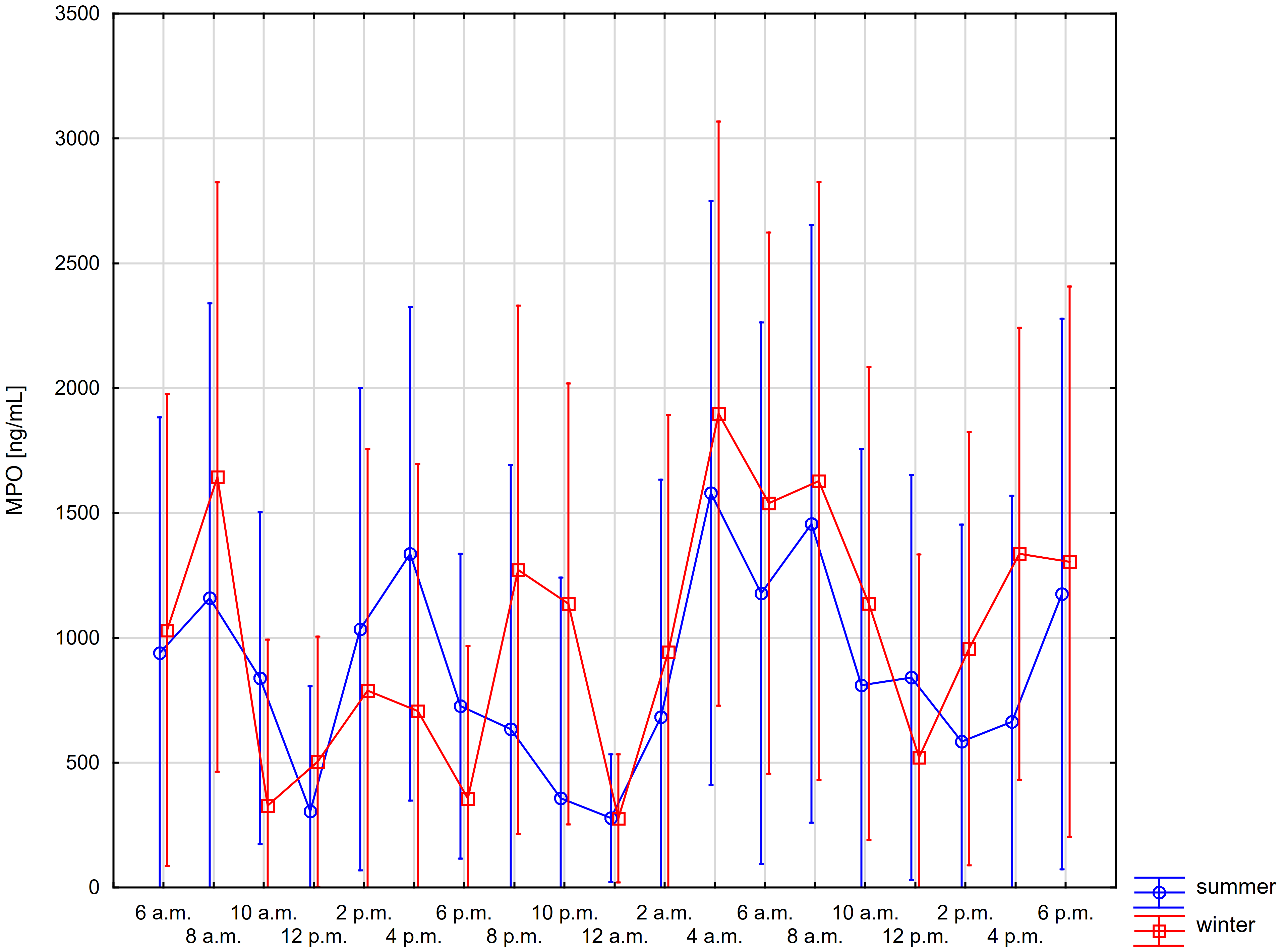
Comparison of daily changes in salivary myeloperoxidase (MPO)
concentration in summer and winter using repeated-measures ANOVA (whiskers show
95% confidence intervals)—time*season: p-value = 0.824, time:
p-value
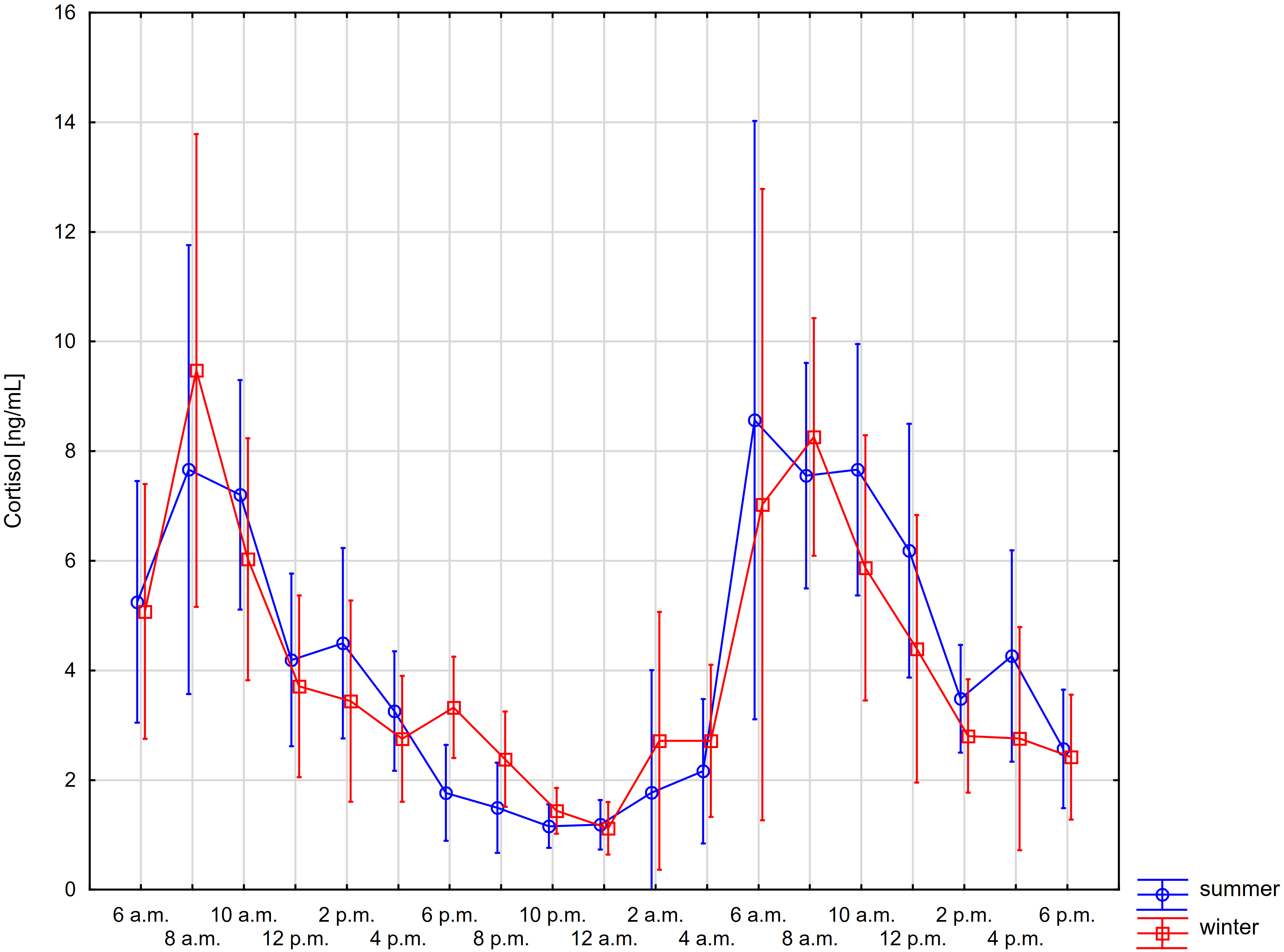
Comparisons of daily changes in salivary cortisol concentration
in summer and winter using repeated-measures ANOVA (whiskers show 95%
confidence intervals)—time*season: p-value = 0.933, time:
p-value
For salivary MPO, significantly higher concentrations compared to midnight and noon were observed for 4 a.m. in both summer and winter. Similar significant differences, although less marked, were recorded for levels at 4 p.m. (Fig. 1).
In contrast, salivary cortisol levels recorded at 8 a.m. were significantly higher than levels between 12 p.m. and 4 a.m. in both summer and winter. Interestingly, the levels observed at 10 a.m. were significantly higher than those between 12 p.m. and 4 a.m. in summer and only between 4 p.m. and 4 a.m. in winter. Also, the levels found at 12 p.m. were significantly increased compared with the levels between 4 p.m. and 4 a.m. only in summer (Fig. 2).
The potential rhythms of MPO and cortisol in saliva were evaluated by the cosinor analysis. Table 4 shows the detailed results.
Period [h] | MESOR (95% CI) | Amplitude (95% CI) | Acrophase (95% CI) | Percent Rhythm | p-value^ | ||
Myeloperoxidase [ng/mL] | summer | 12 | 857.0 (207.5–1506.5) | 306.0 (30.3–581.7) | 05:37 a.m./p.m. (04:09–08:11) | 36.51 | 0.089 |
winter | 12 | 997.8 (340.6–1654.9) | 356.6 (168.4–544.8) | 06:16 a.m./p.m. (04:32–7:35) | 29.92 | 0.008 | |
p-value | 0.734 | 0.909 | 0.284 | ||||
Cortisol [ng/mL] | summer | 24 | 3.8 (3.1–4.6) | 3.2 (2.2–4.2) | 09:18 (08:09–10:30) | 78.82 | |
winter | 24 | 3.9 (3.5–4.3) | 2.7 (1.8–3.6) | 08:16 (06:38–10:00) | 71.00 | ||
p-value | 0.938 | 0.811 | 0.005* |
*significant difference between seasons; ^for the zero-amplitude test. MESOR, midline estimating statistic of rhythm; CI, confidence interval.
The fluctuations in salivary MPO levels showed a moderate fit for function with 12-hour periods for rhythms. However, no significant differences in the rhythm parameters were observed in summer and winter. In contrast, for salivary cortisol concentrations, significant circadian rhythms were found both in summer and winter. In summer, acrophase was significantly delayed by about 1 hour compared to winter, despite the absence of differences in MESOR and amplitudes. Both models concerning salivary cortisol were characterised by high quality.
Since half of the participants met the criteria for SAD, the additional cosinor analysis was performed separately for subjects without and with SAD (Table 5).
Participants without SAD | Participants with SAD | Comparison between participants | |||||||||||||
Period [h] | MESOR (95% CI) | Amplitude (95% CI) | Acrophase (95% CI) | Percent Rhythm | p-value^ | MESOR (95% CI) | Amplitude (95% CI) | Acrophase (95% CI) | Percent Rhythm | p-value^ | MESOR | Amplitude | Acrophase | ||
MPO [ng/mL] | summer | 12 | 953.2 (589.3–1317.1) | 362.7 (46.0–679.3) | 06:30 a.m./p.m. (03:50–08:32) | 17.27 | 0.132 | 760.8 (–880.0–2410.4) | 329.3 (–356.9–1015.6) | 04:39 a.m./p.m. (n/a) | 58.81 | 0.344 | - | - | - |
winter | 12 | 1161.0 (354.2–1967.7) | 581.0 (183.6–978.4) | 07:17 a.m./p.m. (05:22–08:18) | 31.34 | 0.056 | 834.6 (–639.9–2309.0) | 361.5 (–8.8–731.7) | 04:28 a.m./p.m. (n/a) | 25.93 | 0.181 | - | - | - | |
p-value | - | - | - | - | - | - | |||||||||
Cortisol [ng/mL] | summer | 24 | 4.1 (2.6–5.7) | 3.5 (1.5–5.5) | 10:19 (09:09–12:29) | 78.71 | 0.035 | 3.5 (2.6–4.4) | 3.1 (1.5–4.6) | 08:08 (06:42–09:57) | 61.49 | 0.037 | 0.374 | 0.821 | 0.014 |
winter | 24 | 3.7 (3.0–4.3) | 2.9 (1.8–4.0) | 09:53 (09:16–11:07) | 48.86 | 4.1 (3.4–4.7) | 3.0 (1.0–5.0) | 06:43 (04:09–09:43) | 68.50 | 0.075 | 0.257 | 0.954 | 0.009 | ||
p-value | 0.454 | 0.743 | 0.011* | 0.212 | 0.986 | 0.011* |
*significant difference between seasons;
For the salivary MPO, in SAD subjects, no significant rhythm was detected, while for non-SAD subjects, the rhythm in winter was borderline significant. If these rhythms were statistically significant, SAD participants would be characterised by earlier acrophases.
Again, for the salivary cortisol, significant differences were observed only in acrophases. Both participants without and with SAD demonstrated earlier cortisol peaks in saliva in winter compared to summer - for the former, the difference was about 30 minutes, and for the latter already 90 minutes. Therefore, there were significant differences between acrophases for different seasons in subjects without and with self-assessed SAD. The individual characteristics of seasonal cortisol rhythms were reported in Supplementary Table 1.
Moreover, Table 6 shows Spearman’s coefficients for the correlation between GSS and the relative amplitudes (the amplitude/MESOR ratios) for the salivary MPO and cortisol. Only a significant strong positive monotonic correlation was observed for MPO in winter (Supplementary Fig. 1).
R |
p-value | ||
Myeloperoxidase [ng/mL] | summer | 0.189 | 0.601 |
winter | 0.811 | 0.004* | |
Cortisol [ng/mL] | summer | 0.384 | 0.273 |
winter | 0.390 | 0.265 |
*statistically significant correlation.
In the present study, we wished to determine whether the secretion of MPO in saliva demonstrated diurnal and seasonal variations using cosinor analysis. The findings suggest that salivary MPO may have shorter 12-hour ultradian rhythms, although the power of these models was relatively moderate. The MPO acrophases were observed in the early morning and the early evening, with no noticeable variability depending on the seasons. Also, the presence of self-assessed SAD showed no significant variances. MPO daily variations do not perfectly follow a sinusoidal waveform, so we consider alternatives to the cosinor method, such as non-parametric approaches to investigate MPO patterns. We found that increased self-assessed SAD (higher GSS values) was associated with stronger relative amplitude in winter season only.
Moreover, the presence of the circadian rhythm of cortisol secretion in saliva confirmed the rhythmicity of the volunteers. They demonstrated the cortisol peaks in the morning hours, with the observed delay in acrophase in summer compared to winter. The participants with self-assessed SAD demonstrated a stronger shift of peak to earlier morning hours, especially in winter.
To our best knowledge, this is the first study to address the potential rhythmicity of MPO secretion in healthy volunteers, using saliva as a diagnostic medium helpful in chronobiology. Saliva samples are relatively easy to collect in a non-invasive way, unlike blood [46]. Due to its dual nature, MPO exhibits prooxidative and antioxidant properties, especially in saliva [47, 48]. Thus, salivary MPO levels reflect the oxidative status of the human organism - both systemic elements associated, for example, with increased metabolic states, as well as local ones caused even by microinflammation in the oral cavity [49]. In the literature, there are previous reports about daily changes in antioxidant enzymes, but mainly in the serum of patients with systemic diseases or animals [33, 50]. Apart from the frequency of measurements, the undoubted advantage of our study is the homogeneity of the study group consisting of 10 healthy men, mainly in the third decade of life. Moreover, their sleep parameters, activity and eating habits did not differ significantly between summer and winter.
Only a few studies have discussed the daily MPO variations, not the rhythms. Dominguez-Rodriguez et al. [51] observed significant differences in MPO levels comparing the dark and light periods (2 a.m. vs 9 a.m.) in STEMI patients with higher levels in the dark phase, suggesting the existence of diurnal differences in these patients. In the healthy subjects, MPO concentrations were lower in both dark and light periods, without day/night variations. Also, in the study by Uluoglu et al. [52], the activity of MPO in the rat liver was significantly higher in the diabetics than in the controls, however, without a circadian variation.
Surprisingly, our analysis suggests the 12-hour ultradian rhythm for MPO; however, it is known that circadian rhythms are fundamental regulators of neutrophil receptor expression, counts, and tissue homing. Although inflammatory processes can also reprogram rhythms, it seems that rhythmicity-related treatment should be more effective in therapeutic response [53].
Light is an essential factor influencing circadian and seasonal rhythms [54]. A physiological link between the light/dark cycle and MPO variability may be related to diurnal rhythmicity in neutrophils and endothelial activity, which is known to occur in the morning hours [55]. The involvement of these parameters and MPO in atheromatous lesions, resulting in CVD events, is well established [56]. In the large population study, the highest incidence of acute myocardial infarction occurred at 9 a.m., with a second lower incidence peak at 8 p.m., and the lowest incidence during the night [57]. Thus, diurnal rhythm in MPO can modulate susceptibility to CVD events.
In turn, Tenovuo and Valtakoski [58] suggested that the circadian variation in peroxidase activity and redox potential positively correlated with the variations in the salivary flow rate during the day. Decreased salivation around noon and late evening was associated with decreased peroxidase activity. This tendency is similar to our ultradian rhythmicity. The higher peroxidase activity should result in the lowered redox potentials of the oral environment by decomposing hydrogen peroxide. Also, the differences found in peroxidase activity and salivary flow rate did not correlate with the clinical conditions of the oral cavity.
In the literature, previous studies have been concerned on diurnal variations of antioxidants, mainly in plasma. Budkowska et al. [59] suggest that increased levels of antioxidants at night may be associated with the intensification of processes responsible for removing oxygen free radicals.
In particular, more studies have focused on the daily rhythms of plasma antioxidants in selected diseases. Kushwaha et al. [60] determined that the renal stone formers demonstrated significantly lower MESOR of SOD, GPx, GR and CAT. Also, the patients differed from the healthy subjects in terms of the circadian amplitudes and acrophases for almost all determined enzymes (except amplitude for GPx and acrophase for SOD). In cirrhotic patients, circadian amplitudes of plasma CAT, GPx and GR, as well as MESOR for plasma SOD were lowered, indicating a decrease in antioxidant defensive mechanisms [61]. Similar findings were observed for patients with gynaecological malignancies and pulmonary tuberculosis [62, 63]. In the recent study, Singh et al. [64] found lower circadian amplitudes and MESOR for plasma SOD, GPx and GR in patients suffering from peptic ulcer disease. The reduced activity of these primary defence enzymes could be caused by the greater involvement of reactive oxygen species in the pathogenesis of gastric ulcers, interfering with the pro- vs antioxidant ratio.
Interestingly, Goncharova et al. [65] observed a tight correlation between the diurnal changes in the SOD activity and the cortisol levels in the monkeys’ blood plasma. Despite the flattening of circadian rhythms with ageing, the correlation between these parameters remained. It suggests that corticosteroids may play a role in regulating SOD activity in non-human primates, which decreases with ageing.
In contrast to the rhythms of antioxidants, the daily cortisol rhythm is much better known, but there is no agreement in the literature as to seasonal rhythmicity. Based on the CIRCORT dataset, Miller et al. [35] observed elevated levels of salivary cortisol in winter and spring, with accompanying delayed acrophases. In the same study, the authors found that men between 20 and 30 years of age had significantly earlier demonstrated a daily increase in cortisol levels in saliva, compared to the age-matched women. Also, these analyses described the expected circadian variations of salivary cortisol levels with an immediate increase about an hour after awakening and a gradual decrease during the day, leading to a nadir around bedtime.
Previously, Hadlow et al. [66] stated that the sunrise time might be the most crucial zeitgeber for the seasonal variations in the cortisol acrophase. Similarly, Vondrasová et al. [67] investigated that exposure to a natural bright light photoperiod in summer advanced the morning salivary cortisol rise compared with exposure to a combined artificial and natural light photoperiod in winter. However, in the study by Persson et al. [68], the diurnal concentration pattern for salivary cortisol was similar across months, with higher levels in February, March and April, and lower ones in July and August.
In contrast, Kanikowska et al. [36] presented a significantly higher MESOR of salivary cortisol without the differed amplitudes for the volunteer women in summer compared with winter. The acrophase occurred about 4 hours delayed in summer than in winter. The participants with higher scores on the seasonal affective disorder scale demonstrated a tendency toward lower relative cortisol amplitude in summer. Moreover, Thorn et al. [69] concluded that only the salivary cortisol response to awakening was attenuated in participants with self-assessed SAD during the shortened photoperiod in winter. In summer, no differences in psychological and cortisol profiles were found.
Despite the undoubted advantages of the study, which include the mentioned homogeneity of the group and the frequency of measurements, it is important to be aware of the study limitations. The preliminary study was conducted on a relatively small study group in the domestic setting and could have a longer period of time. Studies on circadian rhythms are more often carried out under controlled chamber conditions with adjustable light intensity and strictness of the same diet with a defined fixed meal frequency. Although the participants recorded the parameters of sleep, physical activity and meals consumed, this survey reflected their daily functioning and was not unified between them. Also, it must be stressed that all the questionnaires used were self-reported, thus their findings were subjective in some way. In our study, SAD was not considered as a condition diagnosed by medical professionals, but only as a potential confounder determined via a self-assessment form. The volunteers did not suffer from any systemic inflammatory diseases that could affect their antioxidant status, so our findings cannot be directly reflected in a forward-looking approach to optimising drug delivery times based on circadian rhythms. Further studies should also include chronotype confounding and measuring cyclic internal factors such as body temperature or a sleep-activity rhythm.
Our observations suggest the possible occurrence of the ultradian rhythm for MPO in saliva, with two peaks during the day, regardless of the season. On the other hand, our male volunteers presented the salivary cortisol diurnal rhythmicity and the potential variability of this rhythm depending on the season. Considering individual factors, such as seasonal affective disorder, is also important. As mentioned, the potential daily variations of the oxidative status markers seem to be promising factors in planning more effective treatment models for systemic diseases. However, further research is needed to verify these findings.
Data are available on request from the corresponding author.
KN is responsible for conceptualisation, methodology, formal analysis, investigation and resources, writing the original draft, review and editing, visualisation. RR is responsible for investigation and resources. DG is responsible for investigation and resources. JW is responsible for conceptualisation and methodology. AS is responsible for conceptualisation and supervision. DK is responsible for conceptualisation, methodology, writing review and editing, and supervision. All authors contributed to editorial changes in the manuscript. All authors read and approved the final manuscript. All authors have participated sufficiently in the work and agreed to be accountable for all aspects of the work.
The study was conducted according to the guidelines of the Declaration of Helsinki, and approved by the Bioethics Committee of Poznan University of Medical Sciences (223/19). Informed consent was obtained from all subjects involved in the study.
Not applicable.
This research received no external funding.
The authors declare no conflict of interest. Given his role as Guest Editor, Kacper Nijakowski had no involvement in the peer-review of this article and has no access to information regarding its peer-review. Full responsibility for the editorial process for this article was delegated to Graham Pawelec.
Publisher’s Note: IMR Press stays neutral with regard to jurisdictional claims in published maps and institutional affiliations.