- Academic Editor
Background: Diabetes mellitus type 2 is a risk factor for developing
heart failure and myocardial fibrosis, but there is no specific therapy for
diabetic heart disease. 1-[2-(4-methoxyphenyl)]-2-[3-(4-methoxyphenyl)
propoxy]ethyl-1H-imidazole (SKF96365) is regarded as an inhibitor of
receptor-mediated calcium ion (Ca
Diabetes mellitus (DM) is a general term for a group of metabolic diseases characterised mainly by hyperglycaemia and caused by abnormal insulin secretion or action defects. DM has now become one of the major diseases that threaten human longevity. According to International Diabetes Federation statistics, the number of deaths due to diabetes and its related complications is more than 4.6 million globally each year, and the annual medical expenditure for patients with diabetes is more than 450 billion US dollars [1]. Diabetic cardiomyopathy (DCM) is one of the most serious complications of DM, with high mortality and morbidity [2, 3]. Most patients with DCM are in the subclinical stage, during which the main pathological changes are cardiomyocyte hypertrophy and myocardial fibrosis, and gradually develop abnormal cardiac systolic function with the progression of the disease [4]. The pathogenesis of DCM is complex, and its specific mechanism is not yet fully understood.
Calcium ion (Ca
SKF96365 has been widely used to study the function of TRPC channels [10, 11, 12, 13, 14]. Originally identified as a blocker of receptor-mediated calcium entry, SKF96365 is widely used diagnostically as a blocker of TRPC channels. It has been reported that SKF96365 can inhibit AngII-induced cardiomyocyte hypertrophy [10], but its therapeutic effect and mechanism in DCM are unclear. In this study, a rat model of type-II diabetes was established and SKF96365 was administered. The relevant indicators and pathological changes in myocardial fibrosis were observed and compared to explore the effect of SKF96365 on diabetic myocardial fibrosis. The findings will provide a theoretical basis for the early intervention of patients with diabetes to improve the degree of DCM and provide new ideas for the prevention and treatment of cardiomyopathy in patients with diabetes.
Thirty healthy four-week-old specific pathogen-free (SPF) Wistar male rats (purchased from Beijing Weitong Lihua Laboratory Animal Technology Co., Ltd., SYXK [JING] 2022-0052, China), weighing 180–200 g, were selected for the experiment. The rats were housed at a constant temperature (21 °C–23 °C) and humidity (45%–65%) in a rearing room that maintained a 12-hour light–dark cycle, with free access to food and water. All animal experiments were performed according to the Helsinki Declaration, and this experimental protocol was approved by the Experimental Animal Ethics Committee (approval number: CMU2013070).
The main reagents included the following: streptozotocin (STZ) (Sigma, St. Louis, Missouri, USA), TRIzol reagent (Ambion, Carlsbad, California, USA), SKF96365 (ABCAM, UK), Murine leukaemia virus (MuLV) reverse transcriptase kit (Applied Biosystems, Foster City, CA, USA), Tris (Invitrogen, Carlsbad, USA), ethylene diamine tetra acetic acid (Invitrogen), Agarose (Invitrogen), Paraffin (Leica, Solms, Germany), Radioimmunoprecipitation (RIPA) lysis buffer (Applygen Technologies Inc, Beijing, China), protease inhibitor (Sigma), phosphatase inhibitor (Sigma), chemiluminescence Western Blot Detection Reagent kit (GE Healthcare, Parramatta, Australia) and a Takara reverse transcription–polymerase chain reaction (RT-PCR) Kit (Bao Bioengineering Dalian Co., Ltd., Dalian, China).
The main instruments were as follows: an analytical balance (Sartorius, Gottingen, Germany), a blood glucose meter (Jiangsu Yuyue Medical Equipment Co., Ltd., Danyang, China), a pH meter (Thermo Scientific, Massachusetts, USA), a 4 °C low-temperature refrigerator (Electrolux, Stockholm, Sweden), an incubator (Shanghai Boxun Industrial Co., Ltd.Shanghai, China), a paraffin embedding machine (Leica, Solms,Germany), a paraffin microtome (Leica, Germany), an optical microscope (Olympus, Tokyo, Japan), a low-temperature high-speed centrifuge (Eppendorf, Hamburg, Germany), an ultraviolet spectrophotometer (Shimadzu, Kyoto, Japan), a micropipette (Eppendorf, Hamburg, Germany), a PCR amplifier (Biometra, Jena, Germany), Odyssey image scanning software v3.0 (LiCOR Biosciences, Lincoln, NE, USA), polyvinylidene difluoride (PVDF) membranes (Millipore, Bedford, MA, USA) and an electrophoresis device (Beijing Liuyi Instrument Factory, Beijing, China).
The preparation of the rat model of type-II diabetes was performed in accordance
with the method of Reed et al. [15]. Four-week-old SPF male Wistar rats
were given a high-sugar and high-fat diet (60% fat, 22% carbohydrate [sucrose]
and 18% protein). After 1 week of acclimatisation to the environment, total
calories of about 5.5 kcal/g were given for 8 weeks. After 24 hours of fasting,
an intraperitoneal injection of 1% STZ 35 at mg/kg was administered, and the
indicators were measured after 24 and 48 h. The following were considered to
indicate a successful model: fasting blood glucose
Thirty healthy male Wistar rats were randomly divided into three groups: group A (the blank control group), group B (the diabetes control group) and group C (the diabetes + SKF96365 intervention group), each containing 10 rats. The rats in group B and group C were modelled normally, and the rats in group C were given a 0.74-µmol/kg SKF96365 intraperitoneal injection after successful modelling, once a day for 12 weeks. During this period, the rats in group A were given a normal diet, while those in groups B and C continued to eat a high-sugar and high-fat diet, and their body weight and blood sugar were monitored daily.
After 12 weeks, the rats were injected with 3% pentobarbital sodium (40 mg/kg) intraperitoneally to euthanize them. The weight of the whole of the heart was recorded, and the left ventricle was isolated and weighed on its own. The heart-to-body (whole heart weight/body weight) and left-ventricle-to-body (left ventricular weight/body weight) ratios were calculated.
The tibia was removed, as was the surrounding soft tissue, and the length of the tibia was measured using a vernier calliper (accuracy 0.02 mm), with the main ruler parallel to the tibia length and the two ends of the tibia fully clamped. The shortest distance measured was recorded.
Haematoxylin and eosin (HE) staining was performed in accordance with the method of Yang et al. [16]. Cardiac tissue was fixed in 4% paraformaldehyde for 24 h and then dehydrated and embedded in paraffin. The paraffin block was cut into 4-µm-thick sections using a rotary microtome. The sections were dewaxed with xylene, dehydrated with different concentrations of ethanol, nuclear stained with haematoxylin and differentiated using a differentiation medium. Then, they underwent eosin cytoplasmic staining, were dehydrated with different concentrations of ethanol, cleaned with xylene and sealed with neutral resin; subsequently, a microscopic examination was performed, and the resulting images were analysed.
Masson staining was performed with reference to Li C et al. [17]. Cardiac tissue was fixed in 4% paraformaldehyde for 48 h at 4 °C, dehydrated and embedded in paraffin. The paraffin block was cut into 4-µm-thick sections using a rotary microtome. Staining was performed using Masson’s trichrome, followed by imaging under a microscope (Olympus™, Japan). ImageJ software (V1.8.0, National Institutes of Health, Bethesda, MD, USA) was used to quantify areas of fibrosis and reflect the percentage of blue positive-stained areas in the total tissue area to calculate the myocardial collagen volume fraction (CVF).
Total ribonucleic acid (RNA) extraction from myocardial tissue was performed
using the reagent TRIzol, and complementary deoxyribonucleic acid was synthesised
using the MuLV reverse transcriptase kit. Semi-quantitative reverse RT-PCR was
performed using the polymerase in the Takara RT-PCR kit. After the PCR products
were verified using gel electrophoresis, they were photographed and stored in a
gel imaging system, and the grey values were calculated. Densitometric analysis
was performed to quantify the messenger RNA (mRNA) levels of SKF96365 target
genes relative to the expression of the endogenous reference gene
Gene name | Explain | Primer sequence |
TRPC3 | Forward | 5 |
Reverse | 5 | |
TRPC6 | Forward | 5 |
Reverse | 5 | |
STIM1 | Forward | 5 |
Reverse | 5 | |
Orai1 | Forward | 5 |
Reverse | 5 | |
Homer1 | Forward | 5 |
Reverse | 5 | |
Forward | 5 | |
Reverse | 5 |
Tissues were lysed by adding RIPA lysis buffer containing protease inhibitor and phosphatase inhibitor in concentrations specified by the respective manufacturers. The lysate was centrifuged at 12,000 g for 10 min, and the supernatant was collected. Protein concentration was determined using a bicinchoninic acid assay. Then, the samples were subjected to sodium dodecyl sulphate–polyacrylamide gel electrophoresis, and the proteins were then transferred onto PVDF membranes. The PVDF membranes were blocked with 5% skimmed milk dissolved in Tris-buffered saline containing 0.1% Tween 20 (TBS-T); then, they were incubated overnight at 4 ℃ with specific antibodies against STIM1, Orai1, Homer1 and glyceraldehyde 3-phosphate dehydrogenase (Cambridge, MA, USA). The membranes were washed in TBS-T and incubated with the appropriate horseradish peroxidase-conjugated secondary antibody for 1 h at 25 ℃. Finally, the membranes were washed with TBS-T and developed using an enhanced chemiluminescence Western blot detection reagent kit. Odyssey image scanning software v3.0 (Lincoln, Nebraska, USA) was used to obtain images of the membranes.
Statistical analysis was performed using SPSS 19.0 software (IBM Corp., Chicago,
IL, USA). All data are presented as mean
The body weight and postprandial blood glucose indexes of the rats in all three
groups were detected, and the results are shown in Fig. 1. Compared with the
weight of the rats in group A, the weights in groups B and C decreased, and the
difference was statistically significant (p
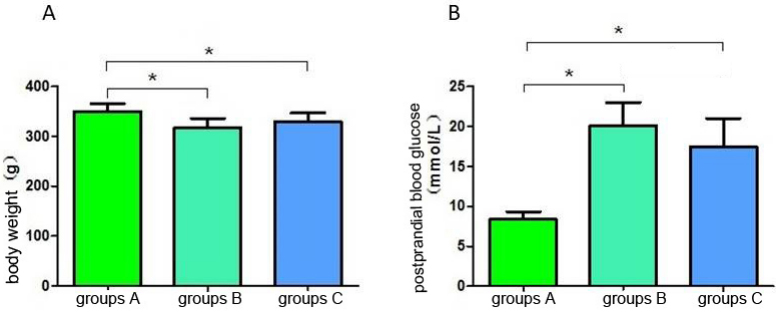
SKF96365 promote postprandial glucose decline. (A) Comparison
of body weight of rats in each group. (B) The postprandial blood glucose of rats
in each group. *p
Compared with the whole heart weight of the rats in group A, that of the rats in
group B increased; the difference was statistically significant (p
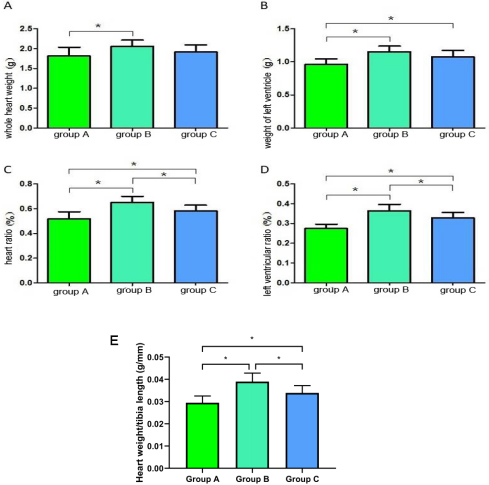
Comparison of cardiac physiological indexes of rats in each
group. (A) Whole heart weight comparison. (B) Left ventricular weight
comparison. (C) Heart weight ratio. (D) Left ventricular weight ratio. (E) The
ratio of heart weight to tibial length was used to assess myocardial hypertrophy.
*p
Haematoxylin and eosin staining was used to observe the pathological changes in the rats’ myocardia. The HE staining results showed that the myocardia of the rats in group A were arranged regularly and neatly; the intercellular space was small, and the myocardial texture was visible.
Compared with the rat cardiomyocytes in group A, those in group B were hypertrophied, the arrangement of cardiomyocytes was disordered, the myocardial texture was blurred (Fig. 3A), the intercellular space was enlarged, and the average width of the cardiomyocytes was increased (Fig. 3B). The degree of myocardial cell hypertrophy in group C was lower than that in group B. Compared with the myocardial arrangement in group B, the myocardial arrangement was better in group C, and the texture of myocardial fibres was slightly blurred. The average width of the cardiomyocytes in group C was significantly lower than that in group B (Fig. 3).

Haematoxylin–eosin (HE) staining of rat heart tissue in each group (200
Masson staining was performed on rat myocardial tissues to observe the degree of
fibrosis. The staining results showed that myocardial fibres were red, and
myocardial collagen fibres were blue–purple. The collagen fibres in group A were
evenly distributed; compared with the collagen fibres in group A, those in group
B were increased and their distribution was uneven, whereas the collagen fibres
in group C were reduced compared with those in group B (Fig. 4A). The myocardial
CVF of the groups showed that group B
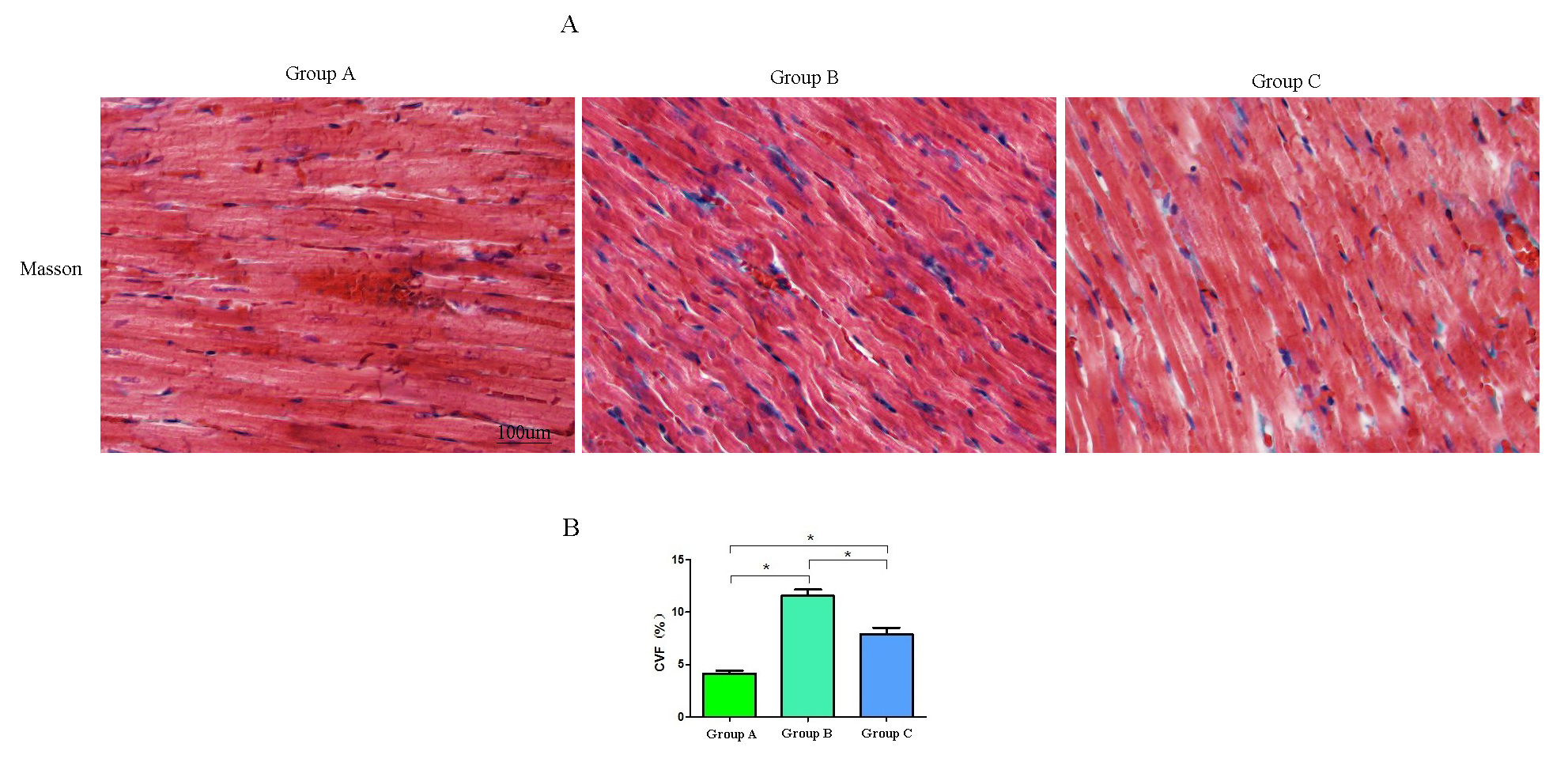
Masson staining. (A) Images of rat heart tissue in each group
(200
The semi-quantitative results showed that the myocardial TRPC3 mRNA and TRPC6
mRNA expressions in groups B and C were significantly higher than those in group
A (p
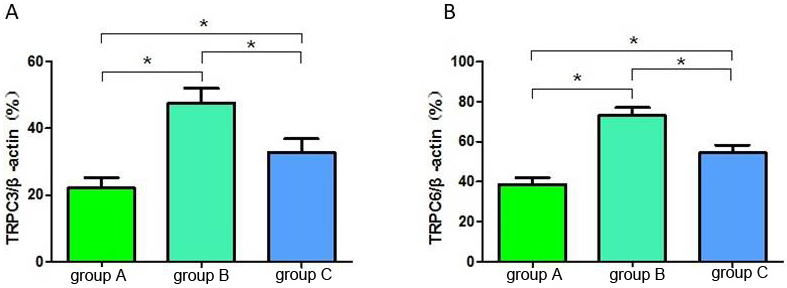
The relative gray value ratio of TRPC3 (A) and TRPC6 (B) in each
group of rats. *p
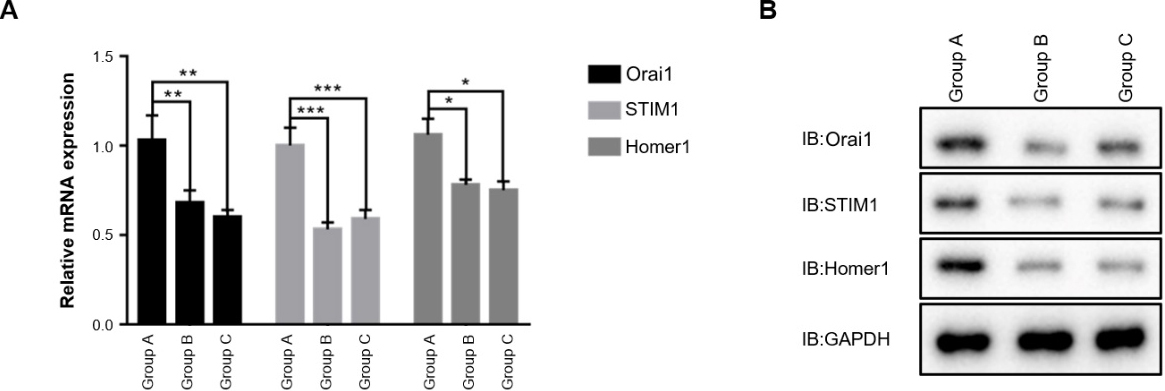
The mRNA (A) and protein (B) expression of other targets of
SKF96365 in each group of rats. *p
Cardiomyocyte fibrosis is the main obvious pathological feature of DCM, which increases myocardial stiffness, causing diastolic dysfunction [18]. Luo et al.’s [19] study of diabetic rats showed eccentric myocardial hypertrophy, and they found that the ratio of heart to body weight was extremely large, the ultrastructure of the left ventricle was severely damaged, and the myocardial interstitial fibrosis was aggravated. The present study showed that the heart-to-body-weight ratio and the left-ventricle-to-body-weight ratio of the diabetic model rats were significantly higher than those of the normal rats, which suggested that the diabetic rats had developed cardiac lesions. Further pathological HE staining showed myocardial hypertrophy, disordered myocardial cells, enlarged intercellular spaces and blurred myocardial texture. In addition, Masson staining revealed severe myocardial fibrosis in the diabetic rats’ myocardia.
Short-term hyperinsulinemia in DM may regulate glucose uptake through Phosphoinositide-3 kinase (PI3K)/protein kinase B or AKT serine/threonine kinase (Akt), thereby stimulating cell growth and causing cardiac hypertrophy [20]. Hyperglycaemia activates signalling molecules (such as c-Jun N-terminal kinase 1) in cardiomyocytes, and oxidative stress also mediates both cardiomyocyte hypertrophy and fibrosis [21, 22]. In addition, abnormal vascular growth factors and changes in tiny blood vessels, especially arteries and capillaries, are prone to phenomena such as fatty infiltration, intimal and subintimal hyperplasia and basement membrane thickening. It further blocks the discharge of harmful substances and aggravates myocardial damage [23]. The complexity of the pathogenesis of DCM implies that more time and energy should be invested in its understanding.
Transient receptor potential canonical channels are a group of ion channels with
many physiological functions [24]. After the expression products of the
TRPC gene combine with each other to form homo- or hetero-polymers
in vivo, they can form non-selective ion channels through which
Ca
In this study, compared with normal rats, diabetic rats showed morphological changes, including increased heart mass, myocardial hypertrophy and upregulated expression of TRPC3 and TRPC6 genes in heart tissue, suggesting that TRPC3 and TRPC6 may be involved in the physiological process of the development of DCM. During DCM development, TRPC3/Nox2-induced oxidative stress processes have been shown to be involved in cardiac fibrosis [28]. However, studies by Oda et al. [29] showed that the deletion of TRPC6 exacerbated heart failure in diabetic mice and oxidative stress, while the deletion of TRPC6 had no significant effect on myocardial fibrosis in DCM mice. Although TRPC3 and TRPC6 have a high degree of functional similarity, TRPC3 and TRPC6 differ substantially in their basal channel activities. Oda S et al. [29] reported that TRPC6 counteracts the TRPC3–Nox2 protein complex, leading to the attenuation of hyperglycaemia-induced heart failure in mice, which may not be related to TRPC6 channel activity. The specific function of TRPC6 in DCM still needs further exploration.
The protective effects of SKF96365 on the nervous system, renal system and
vascular system in diabetic animal models have been preliminarily confirmed
[28, 30]. In the present study, the administration of SKF96365 to type-II diabetes
model rats significantly improved the degree of diabetic myocardial fibrosis. In
the process of ventricular remodelling in DCM, the intracellular calcium signal
transduction pathway plays an important role in mediating myocardial hypertrophy
and myocardial fibrosis. In the intracellular Ca
There are certain limitations to this study. First, due to the constraints of available resources and the timeframe of the research project, the study used a relatively primitive type-II diabetic rat model, wherein the rats were treated with a high-sugar and high-fat diet in combination with a low-dose STZ injection to induce insulin-resistance symptoms. However, although this method is relatively stable, reliable and easy to implement, it lacks a unified standard, and there is no consensus on the modelling standard of type-II diabetic rats. According to published studies [34], this diabetic rat model is lighter in weight and has a significant increase in heart and liver coefficients compared with normal rats. Therefore, in this study, the rats in the model-prepared groups (groups B and C) lost weight. Although there are related experimental studies that used this method to simulate type-II diabetic rats, this modelling method is not used in most higher-level experiments, and spontaneous diabetes models, such as ob/ob mice (Leptin Deficiency Mouse Model), db/db mice (derived from autosomal recessive inheritance of C57BL/KsJ inbred strain, belong to the type 2 diabetes model), obese Zucker rats, Wistar–Kyoto rats and SHR/N-cp rats, are more commonly used. The pathophysiological changes in these models are closer to those of clinical patients with type-II diabetes, so the results may have greater validation. Second, SKF96365 is not a specific TRPC inhibitor but more widely a SOCE inhibitor that acts against TRPC but also other actors, such as voltage-gated calcium channels [35]. There are significant overlapping physiological and pathophysiological associations between TRPC channels and low-pressure activated T-type calcium channels. In future studies, the authors will use gene editing to construct TRPC-deficient rats and specifically block TRPC3/6 to further study the beneficial effect of TRPC on diabetic myocardial fibrosis.
This study found that in diabetic rats, the myocardial tissue was damaged, and the degree of myocardial fibrosis was high. SKF96365 significantly reduced both myocardial CVF and the cardiac and left ventricular ratios and significantly improved myocardial fibrosis in diabetic rats.
TRPC, transient receptor potential channel; TRP, transient receptor potential; IDF, International Diabetes Federation; DM, diabetes mellitus; DCM, diabetes cardiomyopathy; STZ, streptozocin; UV, Ultraviolet; EDTA, Ethylene Diamine Tetraacetic Acid; HE, hematoxylin-eosin; PBS, phosphate buffered saline; CVF, collagen volume fraction; RT-PCR, reverse transcription-polymerise chain reaction; OD, optical density; Tris, Tris (Hydroxymethyl) aminomethane; EDTA, ethylene diamine tetraacetic acid; EB, ethidium bromide; PI3K, phosphatidylinositide 3-kinase; PKB, protein kinase B; JNK1, c-Jun N-terminal kinases-1; NFATs, nuclear factor of activated T cells; ANF, atrial natriuretic factor; BNP, brain natriuretic peptide; NFATs, nuclear factor of activated T cells.
Not applicable.
The data that support the findings of this study are available from the corresponding author upon reasonable request.
HL and WW conceived of the study, and participated in its design and coordination and helped to draft the manuscript. Both authors contributed to editorial changes in the manuscript. Both authors read and approved the final manuscript. Both authors have participated sufficiently in the work and agreed to be accountable for all aspects of the work).
This study was conducted in accordance with the Declaration of Helsinki and approved by the Ethics Committee of the First Affiliated Hospital of China Medical University (CMU2013070).
We would like to express our gratitude to all those who helped us during the writing of this manuscript.
This research received no external funding.
The authors declare no conflict of interest.
Publisher’s Note: IMR Press stays neutral with regard to jurisdictional claims in published maps and institutional affiliations.