- Academic Editor
Nonalcoholic fatty liver disease (NAFLD) is a pathological syndrome characterized by excessive fat deposition in hepatocytes. A sedentary lifestyle is a major risk factor for NAFLD, and regular exercise is considered a cornerstone of NAFLD treatment independent of weight loss. Even low-intensity activity could have beneficial effects on NAFLD. Fibroblast growth factor 21 (FGF21), a cytokine mainly secreted by the liver, improves glucolipid metabolism, reduces inflammation and oxidative stress, increases insulin sensitivity, and acts on multiple organs through autocrine, paracrine, and endocrine actions. Both clinical trials and animal experiments have shown a high correlation between liver fat content and circulating blood FGF21 levels, and abnormal FGF21 signaling appears to be an important mechanism for the development of NAFLD. FGF21 is an exerkine that responds to exercise; therefore, it may be a key target in exercise to improve NAFLD. This review provides an overview of NAFLD and its pathogenesis, and summarizes the effects of exercise intervention on NAFLD, as well as the role of FGF21 in NAFLD. Emphasis is placed on possible mechanisms for improving NAFLD by targeting FGF21 during exercise.
Non-alcoholic fatty liver disease (NAFLD) is the most prevalent chronic liver disease globally, affecting about 25.24% of the population worldwide [1, 2]. It causes liver inflammation, fibrosis, cirrhosis and even liver cancer, and lead to cardiovascular and metabolic diseases, making it a major public health burden [3]. Obesity, lack of exercise and unhealthy dietary patterns are important risk factors for NAFLD [4]. Although the dangers of NAFLD are well known, no drugs have been approved specifically for the treatment of this disease [5]. Several promising targets for the treatment of NAFLD such as peroxisome proliferator-activated receptor (PPAR), sodium-glucose transport protein 2, glucagon-like peptide 1, farnesoid X receptor, fibroblast growth factor 19 (FGF19), and FGF21 are being actively explored, and related drugs such as pioglitazone, canagliflozin, liraglutid, obeticholic acid, NGM282, and pegbelfermin (BMS-986036) are undergoing clinical studies [6]. Lifestyle and dietary interventions, as well as possible bariatric surgery, are generally recognized as the cornerstones of NAFLD treatment [7].
Long-term sedentary lifestyle and inactivity are important health hazards, and the risk of multiple chronic diseases is directly related to a lack of physical activity [8]. Only recently has the biomedical scientific community clearly acknowledged the significant benefits of regular exercise on the prevention and improvement of several illnesses, even showing exercise to be more effective than some low-performing medicines [9]. Regular exercise reduces liver fat content, increases insulin sensitivity and improves lipid metabolism, thereby relieving NAFLD and other metabolic diseases, independent of weight loss [10]. During exercise, organs such as skeletal muscle, liver, and adipose tissue secrete a series of cytokines such as irisin, interleukin 6 (IL-6), brain-derived neurotrophic factor and FGF21, called exerkines, which have important health benefits [9].
FGF21 is an atypical member of the FGF family with multiple biological roles [11]. It is mainly derived from the liver, although it can also be secreted from other organs such as skeletal muscle, adipose tissue and heart [12]. FGF21 acts via autocrine, paracrine, and endocrine functions, or is released into the circulatory system to exert metabolic regulation on multiple target organs such as the hypothalamus, adipose tissue and pancreas [13]. FGF21 has various important biological roles such as ameliorating oxidative stress [14], exerting anti-inflammatory [15] and anti-apoptotic effects [16], and alleviating endoplasmic reticulum (ER) stress [17], and is a potential drug target for the treatment of various metabolic diseases such as obesity [18, 19], type 2 diabetes mellitus (T2DM) [20], and NAFLD [21].
This review provides an overview of NAFLD and its pathogenesis, and summarizes the effects of exercise intervention on NAFLD, as well as the role of FGF21 in NAFLD and its response to exercise. Emphasis is placed on the effects of FGF21 on exercise intervention in NAFLD and its possible mechanisms.
NAFLD is the accumulation of triglycerides (TGs) in hepatocytes exceeding at least 5% of the liver weight of individuals with little or no alcohol consumption and no other liver disease [22]. The progression of liver disease usually includes the following stages: NAFL (without hepatocellular injury), non-alcoholic steatohepatitis (NASH, inflammation and fibrosis), cirrhosis and Hepatocellular carcinoma (HCC) (Fig. 1) [23, 24]. Liver biopsy is one way to histologically diagnose NAFLD despite its invasive nature. Transient elastography based on ultrasound techniques [25] and magnetic resonance elastography [6] are important noninvasive methods for the histologic diagnosis of NAFLD. In addition, some predictive models of NAFLD, such as fatty liver index [26], hepatic steatosis index [27], fibrosis 4 score [28], Framingham steatosis index [29], percentage of hepatic fat [30], and NAFLD Screening Score [31]; and blood biochemical markers such as aspartate aminotransferase (AST) and alanine aminotransferase (ALT) are also diagnostic for NAFLD.
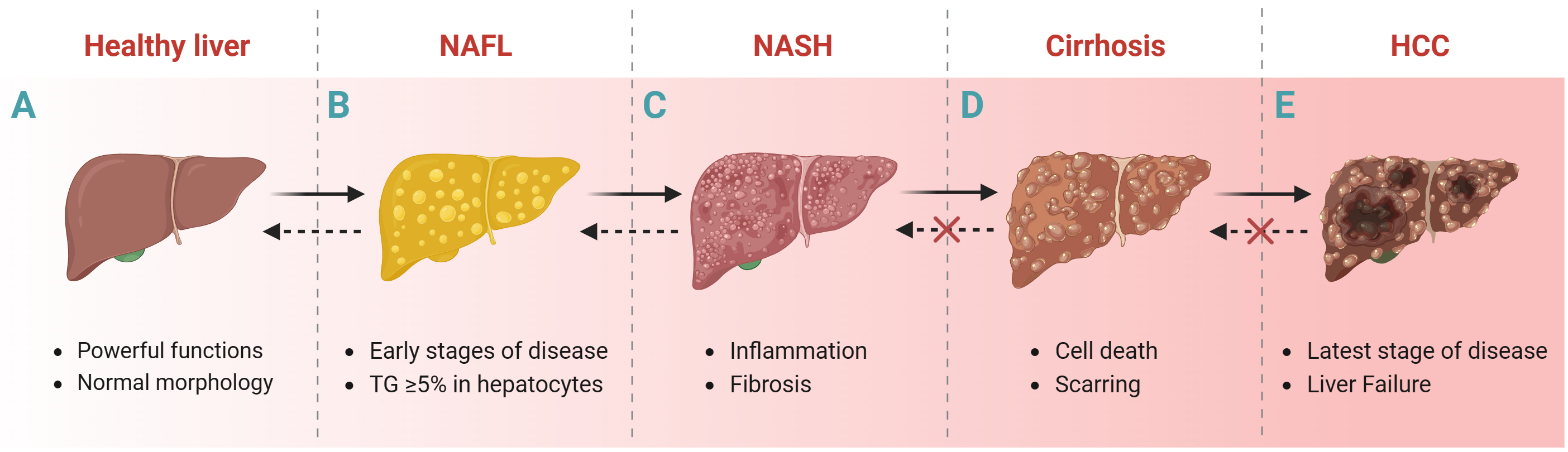
Liver disease progression. Healthy liver to NAFL, NASH, cirrhosis and HCC. Abbreviations: NAFL, non-alcoholic fatty liver; NASH, nonalcoholic steatohepatitis; HCC, hepatocellular carcinoma; TGs, triglycerides. Fig. 1 created with BioRender.com.
As scientific research continues, the possible pathogenesis of NAFLD is gradually being refined (Fig. 2). The “two-hit hypothesis” was originally proposed as one of the hypotheses to explain the pathogenesis of NAFLD [32]. The hypothesis suggested that the “first-hit” was due to a metabolic disturbance caused by insulin resistance (IR), which allowed excess free fatty acids (FFAs) to flow into the liver, promoting the production and accumulation of TGs and causing hepatic steatosis. According to the “second-hit” hypothesis, an increase in pro-inflammatory cytokines and oxidative stress due to hepatic steatosis caused by TG accumulation, results in NASH and fibrosis [33]. However, as the influencing factors of NAFLD were revealed, it was gradually found that the “two-hit hypothesis” was insufficient to explain the process of NAFLD. Therefore, the “multiple-hit hypothesis” was proposed [34]. This hypothesis considers that the pathogenesis of NAFLD may be due to a combination of factors including increased pro-inflammatory cytokines, increased oxidative stress, IR, nutritional factors, alterations in gut microbes, and genetic limitations [34]. Although the cellular and molecular mechanisms of NAFLD have been extensively studied in recent years, the exact mechanism of action of disease development is still not fully understood.
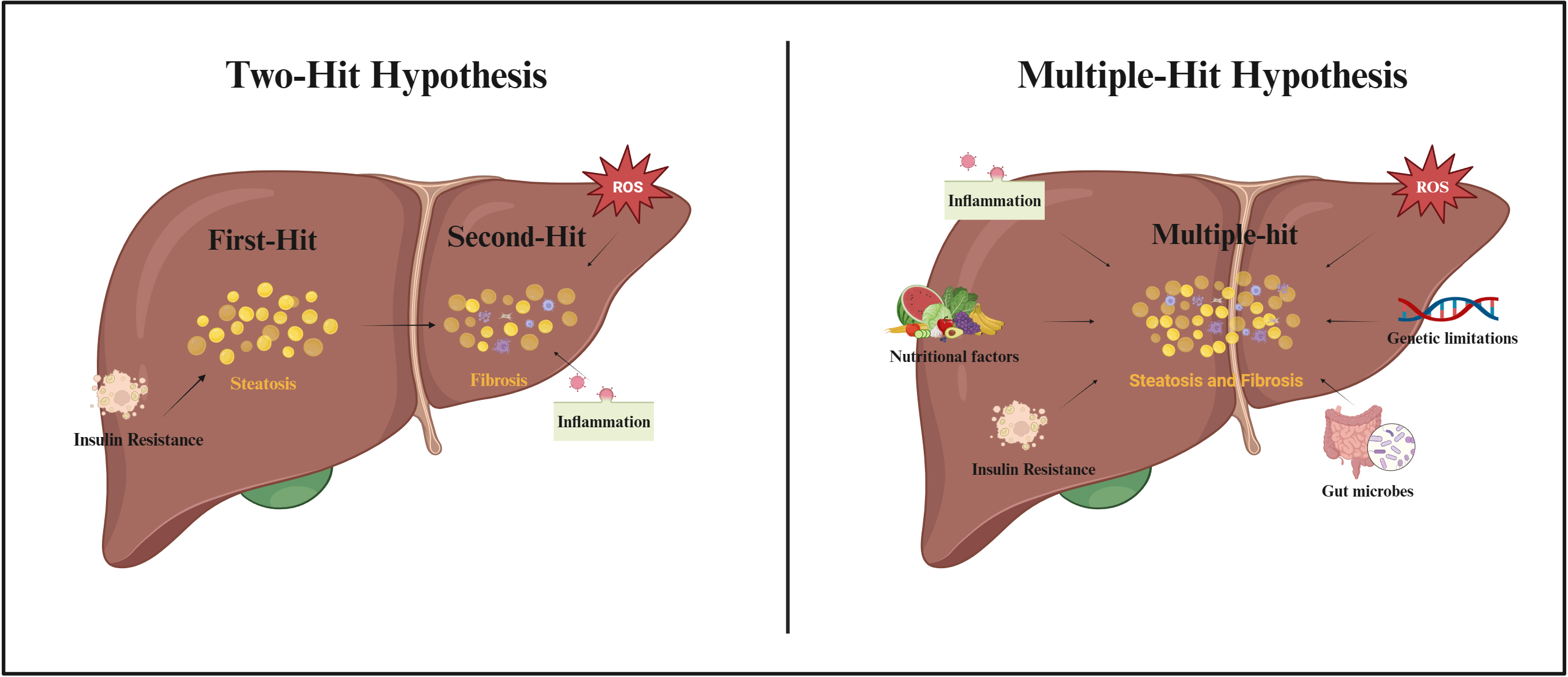
Two-Hit Hypothesis and Multiple-Hit Hypothesis of NAFLD pathogenesis. Abbreviations: ROS, reactive oxygen species. Fig. 2 created with BioRender.com.
Exercise not only improves NAFLD but also serves as an effective preventive tool for several metabolic diseases, such as diabetes [35] and hypertension [36]. The effects of exercise on improving NAFLD include, but are not limited to, reducing intrahepatic lipid content, improving insulin sensitivity, increasing caloric consumption, and promoting weight loss [37]. A variety of regular exercise modalities including aerobic exercise, resistance exercise, and flexibility training could improve NAFLD independent of weight loss [10].
Aerobic exercise is one of the most commonly used forms of exercise to improve NAFLD, as it reduces blood levels of AST and ALT, which are biomarkers of liver damage [38, 39]. Meanwhile, low-intensity aerobic exercises such as brisk walking [40] and cycling [41] are able to reduce liver fat content in patients with NAFLD or obesity, despite no significant change in body weight. In addition, studies have shown that high-intensity aerobic exercise may improve liver fat content and stiffness in patients with NAFLD [42].
Resistance exercise, also known as strength training, is effective in improving muscle strength and mass and is one of the most commonly used exercise methods for NAFLD patients [43]. It has been shown that 8 weeks of resistance exercise is able to reduce liver fat content and increase insulin sensitivity independent of weight loss [44]. In addition, other modalities including high-intensity interval training (HIIT) [45] and concurrent exercise [46] are also exercise methods to improve NAFLD.
Exercise improves IR, and improvement of IR is one of the prominent mechanisms
by which exercise alleviates NAFLD [47]. IR in peripheral tissues such as
skeletal muscle and adipose tissue increases lipolysis and the release of FFAs,
which is subsequently taken up by the liver, resulting in disruption of hepatic
fatty acid metabolism [48]. Exercise improves IR in peripheral tissues, increases
insulin sensitivity, and may reduce excess FFAs flow to the liver [49]. Increased
insulin sensitivity increases glycogen synthesis in the liver and glucose uptake
capacity in skeletal muscle [50]. In addition, exercise also increases the
expression of AMP-activated protein kinase (AMPK) [51] and PPAR
Exercise improves oxidative stress and inflammatory responses. Oxidative stress reflects an imbalance between oxidative and antioxidant systems, leading to the excessive production of reactive oxygen species (ROS), which severely impairs cell function and leads to cell death [53]. The overproduction of ROS and the occurrence of oxidative stress are key mechanisms in the development of NAFLD, leading to impaired antioxidant systems as well as lipid peroxidation and possibly to the activation of inflammation to the point where liver fibrosis and hepatocyte cell death occur [54]. Regular exercise is considered an effective antioxidant [55]. As early as 1983, it was shown that long-term exercise training in rodents could increase the level of antioxidant enzymes, such as glutathione peroxidase (GPX) and superoxide dismutase (SOD), to prevent liver damage caused by oxidative stress [56]. Until recently, studies related to the antioxidant effects of exercise were still being reported. Zhang et al. [57] showed that exercise training alleviated hepatic lipid accumulation and ameliorated lipotoxicity-induced apoptosis in db/db mice via the AMPK/nuclear factor erythroid 2-related factor 2 (Nrf2)/heme oxygenase 1 (HO-1) signaling pathway. Zou et al. [58] reported that swimming intervention attenuated hepatic pathological changes in NAFLD zebrafish through silent information regulator 1 (SIRT1)/AMPK/Nrf2 signaling.
The hepatic inflammatory response is a key mechanism for the progression of
NAFLD to more severe stages, including NASH and fibrosis [59]. During NAFLD,
hepatic Kupffer cells are activated, leading to the massive release of
pro-inflammatory mediators such as tumor necrosis factor alpha (TNF-
Exercise promotes organ crosstalk (Fig. 3). Skeletal muscle is one of the main effector organs in response to movement and is also recognized as an endocrine organ [64]. During exercise, skeletal muscle is able to release exercise-induced proteins, peptides and cytokines that exert autocrine, paracrine, and endocrine effects, collectively known as myokines, to enable crosstalk with other organs. Interestingly, other tissues such as the liver and adipose also respond to exercise by releasing hepatokines and adipokines; myokines, hepatokines and adipokines released during exercise are collectively known as exerkines [65].
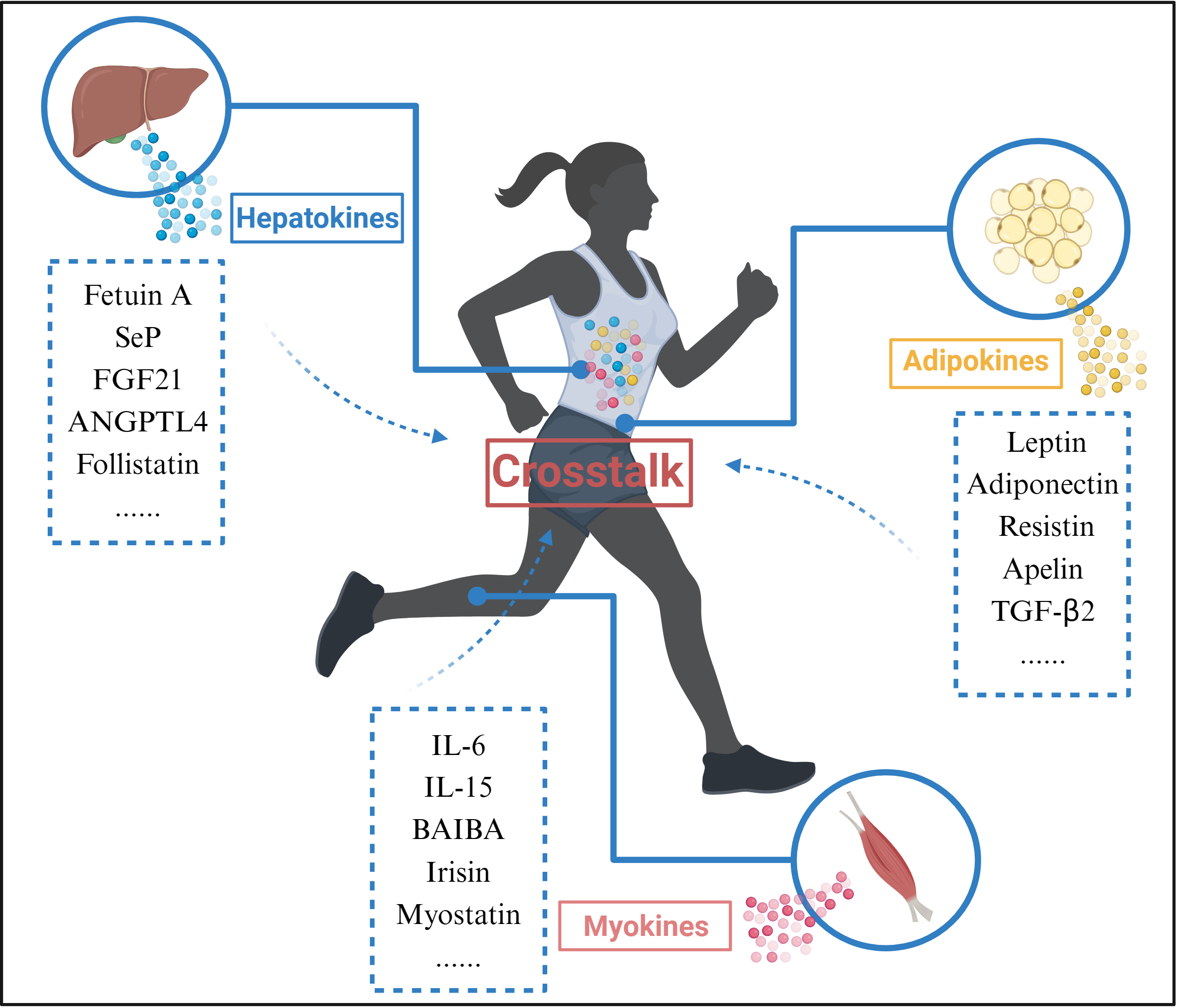
Organ Crosstalk during exercise. During exercise, Myokines,
Hepatokines, and Adipokines released from skeletal muscle, liver, and adipose
tissue interact through blood circulation. Abbreviations: SeP, selenoprotein P;
FGF21, fibroblast growth factor 21; ANGPTL4, angiopoietin-like 4; IL-6,
interleukin 6; IL-15, interleukin 15; BAIBA, beta-aminoisobutyric acid;
TGF-
The exerkines released during exercise may be an important pathway to organ crosstalk. For example, FGF21 is thought to be an exerkine that improves glucolipid metabolism, reduces hepatic steatosis, and improves insulin sensitivity [66]. Although FGF21 is less expressed in skeletal muscle under normal conditions, during exercise, FGF21 may be released by skeletal muscle as a myokine and enter the circulation to interact with other organs such as the liver and adipose tissue [64]. Therefore, organ crosstalk may be one of the mechanisms by which exercise improves NAFLD.
The FGF family is a group of growth factors closely related to cell growth and differentiation, proliferation, wound healing and repair, angiogenesis, embryonic development and other processes. Currently, 23 members of the FGF family have been identified, mainly including endocrine and paracrine types. The FGF family has seven subfamilies. The paracrine FGF1 subfamily, comprising FGF1 and FGF2, play important roles in tissue and organ formation, neural cell regeneration and angiogenesis [67]. The paracrine FGF4 subfamily, comprising FGF4, FGF5, and FGF6, regulates cell proliferation and differentiation, the hair follicle growth cycle, and muscle repair and myocardial function [68]. The paracrine FGF7 subfamily, comprising FGF3, FGF7, FGF10, and FGF22, promotes bone formation and restores trauma repair processes [69, 70]. The paracrine FGF8 subfamily, comprising FGF8, FGF17, and FGF18, is involved in tissue and organ formation, embryonic development, and skeletal developmental processes [71, 72]. The paracrine FGF9 subfamily, comprising FGF9, FGF16, and FGF20, regulates white fat browning and cardiac function [73, 74]. The intracellular FGF11 subfamily, comprising FGF11, FGF12, FGF13, and FGF14, regulates bone resorption and synaptic plasticity [75, 76]. The endocrine FGF15/19 subfamily, comprising FGF15/19, FGF21, FGF23, regulates bile acid homeostasis and glucolipid metabolism, and maintenance of homeostasis [77].
FGF21 is an effective regulator of glucose and lipid metabolism, exerting its
biological effects through FGF receptors (FGFRs) and
Adipose tissue, skeletal muscle, pancreas, and the heart are also capable of
secreting FGF21 [13]. Nutritional excess [85] and cold exposure [86] lead to the
increased expression of FGF21 in adipose tissue, which acts in association with
PPAR
FGF21 functions in complex with FGFRs and
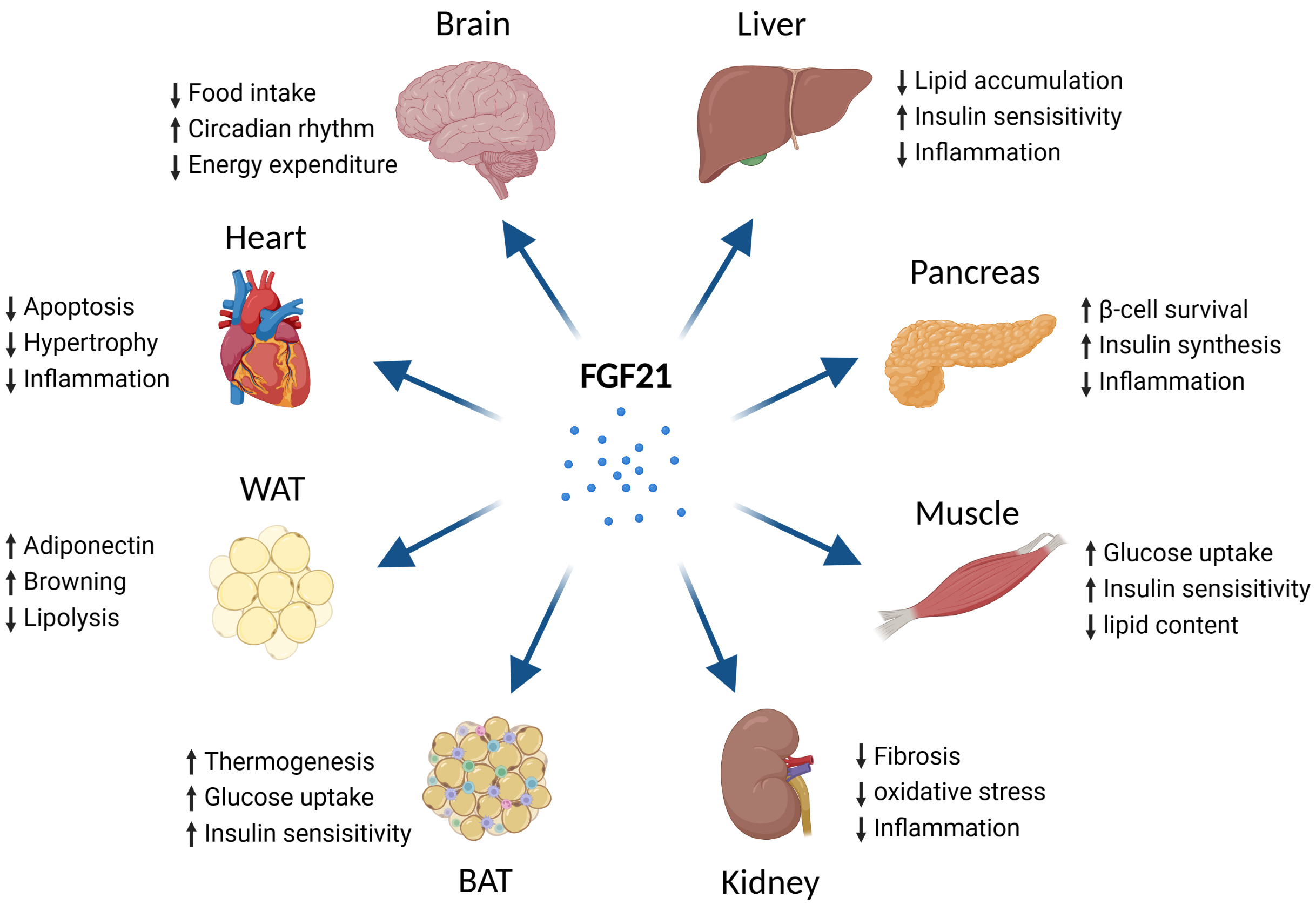
Metabolic effects of FGF21 on different types of tissues. Abbreviations: FGF21, fibroblast growth factor 21; BAT, brown adipose tissue; WAT, white adipose tissue. Fig. 4 created with BioRender.com.
FGF21 levels are elevated in the circulation of NAFLD patients [103], and the same phenomenon has been observed in rodents [104]. Therefore, the notion of “FGF21 resistance” [105] was proposed to explain this change, similar to IR [106], as a compensatory change in organisms. High serum FGF21 are highly correlated with the development of NAFLD [107]. Increased serum FGF21 levels are positively correlated with the degree of hepatic steatosis, as well as serum TG and insulin levels in humans [108], and are negatively associated with high density lipoprotein and the body mass index [109]. In a prospective cohort study of 808 subjects over a 3-year period, elevated serum FGF21 levels were found to predict the development of hepatic steatosis [110]. Another study showed that serum FGF21 level was closely related to hepatic lipid content in a dose-dependent manner in NAFLD patients with central obesity [111]. In addition, it is thought that a reduction in serum FGF21 levels due to gastric bypass surgery may lead to an improvement in NAFLD [112]. In addition, some analogues of FGF21 such as BFKB8488A [113] and efruxifermin [114] have emerged as drug candidates for NAFLD and NASH and entered clinical trial studies. Therefore, FGF21 may serve as a biological marker of NAFLD.
FGF21 reduces steatosis in the development of NAFLD. Hepatic steatosis is the accumulation of excess TGs in hepatocytes, which is one of the main characteristics of NAFLD [115]. Although the excessive accumulation of TGs in hepatocytes has no direct side effects, its continuous release of intermediates, such as fatty acids and ceramides, has toxic effects on the liver [116, 117]. In addition, these intermediates are able to destabilize lysosomes and enhance the inflammatory response and ER stress, thus causing tissue damage [118, 119]. Therefore, hepatic steatosis is an important factor in the development of NAFLD. The role of FGF21 in improving hepatic steatosis in NAFLD has been reported in several studies: First, FGF21 inhibits lipolysis and prevents the flow of excess FFAs into the liver, thus reducing fat accumulation in the liver [120, 121]. Second, mammalian target of rapamycin (mTOR) plays an important role in lipid metabolism and FGF21 is able to inhibit mTOR expression in the liver thereby exerting hypolipidemic effects [122]. In addition, FGF21 inhibits SREBP1c transcription [123] and increases insulin sensitivity [124] to exert lipid regulation.
FGF21 ameliorates oxidative stress in the development of NAFLD. Oxidative stress is a state of imbalance between oxidation and antioxidation in the body, leading to the high production of ROS, which in turn have harmful effects on the organism [53]. Oxidative stress is related to the pathophysiology of metabolic diseases such as NAFLD. For example, excess ROS leads to disorders of hepatic lipid metabolism and IR, triggering liver fibrosis and hepatitis [53, 54]. Elevated ROS levels disrupt ER homeostasis and activate the unfolding protein response signal transduction pathway, which increases FGF21 expression through various pathways including inositol-requiring enzyme 1 alpha/X box binding protein 1 [125], ATF4 [126] and CCAAT enhancer binding protein homologous protein [127]. FGF21 is an important protective factor against oxidative stress in vivo, and is capable of activating a variety of signals such as Nrf2 [128] and AMPK [129]. Exogenous FGF21 reduces ROS production in HepG2 HCC cells [130], and FGF21 analogs alleviate hepatic steatosis, hepatitis, and liver fibrosis in patients with NASH [18]. Therefore, FGF21 is considered an effective regulator of NAFLD [11].
FGF21 ameliorates inflammatory responses in the development of NAFLD.
Inflammation is an immune protective reaction of the organism in response to
various endogenous or exogenous stimuli; moderate inflammation is beneficial to
the organism, while excessive inflammation is harmful [131]. The pathogenesis of
NAFLD is associated with a chronic inflammatory state. Sustained upregulation of
signals including Toll-like receptor 4 (TLR4) [132], nuclear factor kappa-B
(NF-
Studies have shown that different exercise intensities have different effects on serum levels of FGF21. Experiments in 10 healthy men with moderate-intensity exercise (55% maximal oxygen uptake) and high-intensity exercise (75% maximal oxygen uptake) found that both exercise intensities increased their serum FGF21 levels, and that exercise-induced changes in FGF21 were exercise-intensity dependent, with high-intensity exercise providing greater metabolic benefits than low-intensity exercise [141]. FGF21 was also found to be regulated by glucagon. High-intensity exercise has a greater effect on glucagon compared to moderate intensity; therefore FGF21 changes more significantly during high-intensity exercise [141]. In addition, there are significant individualized features of the effect of exercise on serum FGF21. He et al. [142] showed that either HIIT or resistance training increased serum FGF21 levels in untrained male subjects. However, in subjects with moderate levels of training, serum FGF21 levels did not significantly increase even with greater intensity training [143]. Different views have also been reported. Takahashi et al. [144] reported that 12 weeks of resistance exercise reduced serum FGF21 levels in NAFLD patients. Motahari et al. [145] found no significant change in serum FGF21 levels in male T2DM patients with concurrent exercise.
It was also found that long-term metabolic dysregulation such as obesity can
cause a decrease in expression of the FGF21/FGFRs/
As an important cytokine in vivo, FGF21 is secreted by multiple organs and is able to enter the circulation to exert multi-organ interactions [13]. At the same time, FGF21 is an exerkine that responds to exercise [9]. Therefore, it is highly likely that one mechanism of action by which exercise improves NAFLD is by stimulating the secretion of FGF21 from multiple organs, acting on the liver in an autocrine, endocrine, and paracrine manners.
During exercise, organs such as the liver and skeletal muscle are able to
secrete FGF21 and enter the bloodstream for action. In skeletal muscle, exercise
induces the sustained activation of AMPK [92] and AKT [93] signaling,
significantly upregulates FGF21 expression in skeletal muscle, and induces the
secretion of myogenic FGF21. In the liver, exercise stimulates a large increase
in FFAs and depletion of hepatic glycogen, which induces the large secretion of
hepatogenic FGF21 via PPAR
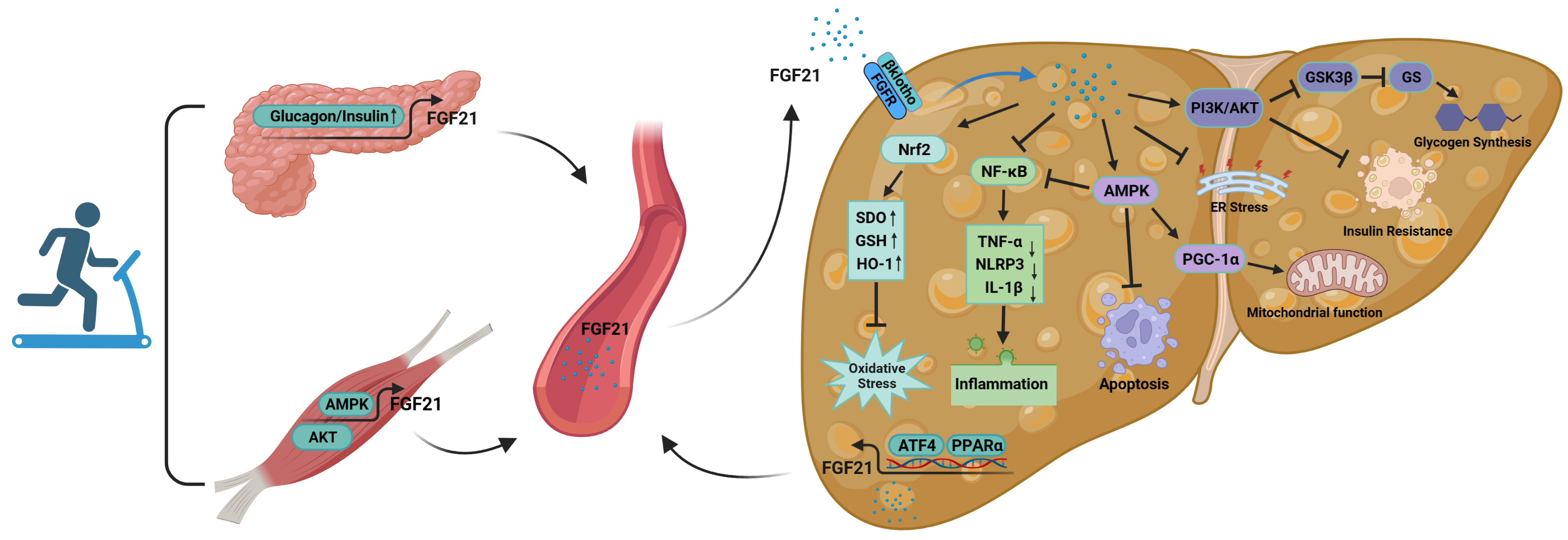
The mechanism of action of FGF21 in the improvement of NAFLD by
exercise. FGF21 is secreted by organs such as skeletal muscle and pancreas
during exercise and acts on the liver through the blood circulation. At the same
time, the liver itself is able to secrete FGF21 during exercise, acting in an
autocrine, paracrine or endocrine manner. Abbreviations: FGFR, fibroblast growth
factor receptor; ER, endoplasmic reticulum; AMPK, AMP-activated protein kinase;
PKB/AKT, protein kinase B; Nrf2, nuclear factor erythroid 2-related factor 2;
SOD, superoxide dismutase; GSH, glutathione; HO-1, heme oxygenase 1;
NF-
FGF21 secreted during exercise is able to act on the liver through blood
circulation. First, FGF21 entering the circulation can activate Nrf2; upregulate
the levels of antioxidant factors such as SOD, glutathione (GSH) and HO-1; and reduce the
excessive accumulation of ROS, thus decreasing the level of oxidative stress in
the liver [152]. Second, these cytokines suppress over-activation of
NF
Exercise interventions are increasingly being recognized as an important non-pharmacological treatment for the prevention and improvement of NAFLD. FGF21, one of the important indicators for improving NAFLD, serves as an exerkine in response to exercise, and multiple exercise modalities have beneficial effects on FGF21. FGF21 is increasingly becoming a crucial target in the development of exercise-improved NAFLD.
FGF21 is an important metabolic regulator that plays an important role in improving glucolipid metabolism, IR, inflammation, and oxidative stress. Changes in serum FGF21 are positively correlated with the development of several metabolic diseases and have become a predictor and biological marker for the early diagnosis of diseases such as NAFLD, T2DM and obesity. Various FGF21 analogs such as LY2405319 [159] and PF-05231023 [160] have entered phase I clinical trials. Although these FGF21 analogs have not achieved great results in glucose metabolism, they have had beneficial effects on improving lipid metabolism in patients with NAFLD and T2DM. Meanwhile, FGF21 is an exerkine that responds to exercise and enables crosstalk between multiple organs through autocrine, endocrine and paracrine approaches. Exercise is also able to improve FGF21 resistance status under pathological conditions. The combination of FGF21 analogs with exercise intervention may bring new hope for the improvement of metabolic diseases such as NAFLD and T2DM. It is worth noting that the short half-life, poor stability and solubility, as well as some potential side effects such as promotion of bone loss in mice [161] and effects on female reproduction [162], have led to concerns about the clinical application of FGF21-based therapies. Meanwhile, a number of drugs that have been approved for the treatment of T2DM have similarly shown benefit in ameliorating NAFLD and may pose a challenge to FGF21 as a therapeutic target for NAFLD. Despite these concerns, FGF21 still has significant potential as a powerful target for improving NAFLD. Thus, more in-depth studies are needed to elucidate the physiological and pharmacological roles of FGF21 and to provide new insights into the development of FGF21 as a therapeutic agent for NAFLD.
Last but not least, prevention rather than cure of NAFLD should be the common goal. How to encourage more patients to participate in exercise should be a key consideration for policy makers. Future research should focus on the precise molecular mechanisms by which FGF21 plays a multi-organ role during exercise, rather than just studying the actions of a single organ. A comprehensive understanding of the role of FGF21 in NAFLD and its changes during exercise are needed to provide novel targets for the establishment of exercise intervention strategies for patients with NAFLD.
NAFLD, Non-alcoholic fatty liver disease; FGF21, Fibroblast growth factor 21;
FGF, fibroblast growth factor; T2DM, type 2 diabetes mellitus; NASH,
non-alcoholic steatohepatitis; AST, aspartate aminotransferase; ALT, alanine
aminotransferase; FFA, free fatty acids; TGs, triglycerides; HIIT, High-intensity
Interval Training; FGFR, fibroblast growth factor receptor; ER, endoplasmic
reticulum; AMPK, AMP-activated protein kinase; PKB/AKT, protein kinase B; Nrf2,
nuclear factor erythroid 2-related factor 2; SOD, superoxide dismutase; GSH,
glutathione; HO-1, heme oxygenase 1; NF-
ZW designed the research study. ZW, TS, JY, and SL performed the research. ZW, LG and YZ provided help and advice on conceptualization, data curation, methodology and formal analysis. All authors contributed to editorial changes in the manuscript. All authors read and approved the final manuscript.
Not applicable.
We thank the authors of the studies included in this review, and thanks to the biorender for the resources, all figures were created with https://www.biorender.com.
This research was funded by Special Fund for Basic Scientific Research of the Central Universities (grant number 20221016) and the Independent research project of Beijing Sport University (grant number 2021TD012).
The authors declare no conflict of interest.
Publisher’s Note: IMR Press stays neutral with regard to jurisdictional claims in published maps and institutional affiliations.