- Academic Editor
Background: Trachyspermum ammi is a frequently utilized
traditional medicinal plant renowned for its pharmacological attributes,
particularly in the realm of treating infectious diseases. This current study
aims to comprehensively assess the in vitro properties of freshly
prepared nanosuspensions derived from Trachyspermum ammi extracts, with
a focus on their cost-effective potential in various areas, including
antioxidant, antibacterial, cytotoxic, and antidiabetic activities.
Methods: Biochemical characterization of T. ammi
nanosuspensions by high-performance liquid chromatography (HPLC) and
Fourier-transform infrared spectroscopy (FTIR) analyses. Results: HPLC
analysis revealed the presence of kaempferol and sinapic acid in various amounts
at 11.5 ppm and 12.3 ppm, respectively. FTIR analysis of T. ammi powder
revealed the presence of alcohols and amines. The assessment of antioxidant
activity was conducted using a DPPH scavenging assay, indicating that the
nanosuspensions exhibited their highest free radical scavenging activity,
reaching 14.9%. Nanosuspensions showed 3.75
Various drugs have been used in the treatment of infectious diseases, yet most of them cause adverse effects on the living tissues in the human body [1]. In the modern era, a variety of chemical drugs, aspirin, clopidogrel, diclofenac, enoxaparin, ibuprofen, naproxen, and warfarin are used for therapeutic purposes. However, excessive use of these drugs increases the risk of cardiac failure, hepatic dysfunction, hemorrhage, respiratory failure, etc. [2]. Thus, due to their adverse side effects, these drugs are strongly prohibited due to hypersensitivity and autoimmunity [3]. Therefore, there is an urgent need for alternatives to chemical drugs to treat infectious and inflammatory diseases. Medicinal plants are a rich source of bioactive compounds and can be an alternative option due to their high bioavailability and minimal side effects [4]. The efficacy or safety of nanosuspensions from many synthetic plants has not previously been evaluated and remains a challenge in green chemistry [5]. Medicinal plants can be used to treat cardiovascular diseases, prostate cancer, myocardial infarction, and liver cirrhosis. Furthermore, they also activate the natural killer cells in the human body and provide long-term immunity for boosting the immune system [6]. Herbal medications are safe and reliable treatment options that are more effective in treating specific ailments than other medications. They are known to be fully natural unless blended with additional chemical components [7].
Trachyspermum ammi L. (Apiaceae) is the most valuable aromatic medicinal plant, which exhibits pharmacological potential for the upcoming exploration of novel herbal medicine [8]. It is naturally produced in countries in southwest Asia, the Mediterranean region, Afghanistan, Iran, Egypt, Iraq, India, and Pakistan [9]. It is used in the treatment of different diseases, abdominal illnesses, poisoning, nausea, appendicitis, hypertension, cardiovascular health issues, constipation, and mosquito bites, while it is used for constipation in traditional Asian and African communities [10]. Its roots, seeds, and leaves are used in the treatment of gastrointestinal disorders, stomach pain, tumors, and piles. Antihelmintic qualities are found in the leaves of ajwain plants, thereby making them valuable for treating helminth infections in animals. Its seeds have anti-vomiting, diuretic, anti-asthmatic, and anti-dyspnea properties [11]. Trachyspermum ammi L. is a rich source of phenolics, antioxidants, flavonoids, polyphenols, and volatile aromatic compounds. These compounds have inhibitory action against infectious diseases and are commonly used for formulating various nutraceuticals [12]. It has been reported that T. ammi oil showed antimalarial, antimicrobial, antibacterial, anti-inflammatory, antioxidant, cytotoxic, nematicidal, and anthelmintic activities [13]. Different studies revealed that green nanoparticle synthesis of T. ammi was performed through different noble metals, such as gold (Au), selenium (Se), magnesium (Mg), and zinc (Zn) [14]. These plant-based noble metallic nanoparticles have shown different biological activities. In comparison to the ethanolic extract of T. ammi, green-synthesized nanoparticles showed a decreasing trend in the above-stated activities. Ethanolic extract, crude extract, and aqueous extract of T. ammi showed higher potential for different biological activities, compared to its nanoparticles and nanosuspension [15].
The nanotechnology approach is applied to formulations of herbal medicines for therapeutic purposes [16, 17]. These nanomedicines have gained special attention in targeting tumors and cancerous cells [18]. Nanosuspensions are defined as nanoparticles, which are made of pure drug, with no matrix material, and an average diameter below 1 µm (typically in the range of 200–500 nm). Nanotherapeutics have improved absorption, minimal toxicity, healing effects, and created better pharmacokinetics [19]. Improving and increasing the bioavailability and solubility of drugs is a major challenge in medicine [14]. However, nanosuspensions have been formulated and designed for medical applications through advancements in nanotechnology, especially in targeting the delivery of nanosuspensions of natural products [20]. The major advantages of nanosuspensions are in the pharmaceutical technicalities of natural product delivery, including increasing dissolution rate and saturated solubility, thereby improving bioavailability for oral administration and enhancing skin surface penetration or cell membrane adhesion for transdermal administration [21]. Techniques such as media milling and high-pressure homogenization have been used commercially for producing nanosuspensions. The unique features of nanosuspensions have enabled their use in various dosage forms, including specialized delivery systems, such as mucoadhesive hydrogels [22].
We hypothesize that nanosuspensions of T. ammi extract showed enhanced bioactivities and bioavailability in therapeutic compounds, compared to its ethanolic extract. The main objectives of this study included the synthesis and the in vitro evaluation of the antioxidant, antibacterial, cytotoxic, and antidiabetic activities of T. ammi extract nanosuspensions. Structural characterization and identification of functional groups in bioactive compounds were accomplished using high-performance liquid chromatography (HPLC) and fourier-transform infrared spectroscopy (FTIR) analyses.
The following chemicals and reagents were procured from the specified sources: Ethanol, acetone, and acetonitrile from Sigma Aldrich (Schnelldorf, Germany); Lot No. S240697, DPPH (2,2-Diphenyl-1-picrylhydrazyl), and alpha-amylase from Sigma Aldrich, PVA (polyvinyl alcohol) from Appli. Chem (Boca Raton, Florida, USA); Lot No. P28C013, bovine serum albumin from Merck (Germany), and Folin–Ciocalteu reagent from Sigma Aldrich; Lot No. 11-0700SAJ.
Trachyspermum ammi (Ajwain) seeds were randomly collected from the local market in Faisalabad and verified by the Department of Botany, University of Agriculture, Faisalabad, Pakistan.
T. ammi seeds were initially grounded into fine powder form, and extraction was carried out using the Soxhlet apparatus. Petroleum ether was used to remove the fatty substances and 95% ethanol was used as the solvent for further extraction. The extract was separated using standard protocols of filtration. Finally, the extract was kept in the desiccator for further use in subsequent experiments [23].
Nanosuspensions of T. ammi were prepared using the nanoprecipitation method. By following this method, 11.25 mL of ethanol and acetone (1:3) were used to dissolve 1.5 g of the plant extract. The resultant solution was injected into 15 milliliters of water using polyvinyl alcohol (1.5% by volume). The resultant emulsion was diluted in 30 mL of an aqueous solution of PVA (0.2% W/V), and it was continuously stirred at 500 rpm for 3 hours. Then, following the evaporation, the solvent and nanosuspensions were produced. Finally, the freshly prepared nanosuspension was stored at –18 °C to keep it frozen and in dry form [23].
We assessed the antioxidant profile of T. ammi using the methods described below. Primarily, we employed colorimetric and scavenging techniques to estimate the antioxidant levels.
Quantification of the total flavonoid content in the sample was carried out using the colorimetric method. Specifically, 38 µL of both the extract and nanosuspension were combined and incubated at ambient room temperature for 10 minutes. Subsequently, 19 µL of a 10% AlCl3 solution was introduced to the mixture and further incubated for 5 minutes. Absorbance measurements were conducted at 510 nm by a microtiter plate reader (Synergy HTX, BioTek, Santa Clara, CA, USA), and the obtained results were compared to a standard catechin calibration curve [24].
The Folin-Ciocalteu assay was employed to quantify the phenolic content in the sample. Briefly, a mixture of diluted reagent (10%; 25 µL) and test samples (125 µL) was prepared and incubated at room temperature for two hours. Subsequently, absorbance was measured using a microtiter plate reader (BioTek, U.S.A.) at a wavelength of 765 nm, and the obtained results were compared with a standard gallic acid calibration curve [25].
The assessment of the antioxidant activity of the T. ammi nanosuspensions and extract was conducted using the 2,2-diphenyl-1-picrylhydrazyl (DPPH) assay. Specifically, a mixture of 250 µL of DPPH solution and 2.5 µL of the extract and nanosuspension solution was prepared and covered with aluminum foil for approximately 35 minutes. All measurements were performed in triplicate [26]. The free radical scavenging capacity was expressed as a percentage of inhibition and calculated using the following formula.
% DPPH scavenging = [A (control) – A (sample) / A (control)]
The biofilm inhibition assay was conducted using a microtiter plate reader. Nutrient broth, plant extracts, and 100 µL of both Escherichia coli and Staphylococcus aureus bacteria were incubated aerobically in a 96-well plate at 37 °C for 12 hours. Subsequently, the plates were rinsed three times with sterile phosphate-buffered saline to remove the adhering bacteria. A 100 µL solution of crystal violet stain (50%) was added to the wells of the microtiter plate, and any excess stain was removed by washing with tap water. Following the drying of the plates, the dye was solubilized with 100 µL of glacial acetic acid (33% v/v) in each well. Absorbance at 630 nm was measured using a plate reader [26]. A positive control was established by using nutrient broth containing ciprofloxacin, while nutrient broth with bacterial strains served as the negative control. The fractions/extracts were examined under a microscope to assess their potential to inhibit the formation of microbial biofilms [26].
The following formula calculated percentage inhibition:
% inhibition = 100
Hemolytic activity was assessed to evaluate the cytotoxicity of the T.
ammi nanosuspensions. The procedure began with the centrifugation of 3 mL of
blood samples to separate the cellular components from the plasma. The separated
red blood cells (RBCs) were washed three times with 5 mL of sterile chilled
isotonic phosphate buffer saline (PBS; pH 7.4), and the plasma was discarded.
Then, the washed cells were subjected to another round of centrifugation at 8000
rpm to obtain a normal saline suspension. The concentration of RBCs was
determined, resulting in a count of 7.068
% hemolysis = A (sample) – A (negative control) / A (positive control) – A
(negative control)
Alpha-amylase inhibition of T. ammi nanosuspensions was evaluated. In a 96-well plate, 30 µL samples were mixed with acarbose for 10 minutes, then treated with 10 µL amylase solution prepared in 0.02 M sodium phosphate buffer (pH 6.9; 0.5 mg/mL). Next, 40 µL of 1% starch solution was added and incubated for 30 minutes. Then, 75 µL of iodine solution and 40 µL (1 M) HCl solution were added to each well. A microtiter plate reader was used to measure absorbance at 630 nm against a blank [28]. The following formula was used to calculate % inhibition:
% inhibition = 1 – A (control) / A (sample)
A spectrophotometer was used to assess the antiglycation potential of the nanosuspension. Firstly, 450 mg bovine serum albumin and 4.5 g D-glucose were mixed together, and the mixture was kept at 37 °C for 24 hours. Absorbance was measured by spectrophotometer at 440 nm for the emission wavelength and 370 nm for the excitation wavelength [29]. The following formula was used to calculate % inhibition:
% inhibition = [A
FTIR spectroscopy was performed to analyze the structure of the T. ammi powder. In chloroauric solution, the substance was centrifuged at 10,000 rpm for 15 minutes. Pellets were washed out thrice with 20 mL of unionized water. The FTIR analysis was carried out by the FTIR system (Agilent Cary 630, Agilent Technologies, Santa Clara, CA, USA) in diffuse reflect array mode with a resolution of 4 cm [30].
High-performance liquid chromatography was performed using a Flexar HPLC system (200, Shelton, WA, USA) to identify different compounds. T. ammi seeds were dried and hydrolyzed. Then, 0.5 g of dried contents were mixed with 20 mL of ethyl alcohol and 1 g/L of BHT (butylated hydroxytoluene). Subsequently, the obtained mixture was combined with 10 mL of 1 M HCl. Saponification was conducted for 15 minutes, and the resulting mixture was employed for HPLC (high-performance liquid chromatography) analysis. A test sample of 20 µL was injected, and the analysis was conducted at 280 nm. Various compounds were identified based on their respective retention times [31].
ANOVA was used to evaluate the data in order to compare the means of two
populations at once and determine whether certain formulation parameters were
significant. p
This study aimed to investigate the cost-effective synthesis and bioactivities
of T. ammi nanosuspensions and its ethanolic extract. The results
demonstrated that T. ammi nanosuspensions exhibited higher total
phenolic contents (TPCs) at 253.04 GAE/100 g compared to the extract, which had
TPCs of 153.06 GAE/100 g. Nanosuspensions exhibited significant biofilm
inhibition against Escherichia coli, with a maximum inhibition of 3.75
Table 1 shows the antioxidant potential of T. ammi
nanosuspensions. The results revealed that nanosuspension showed high total
phenolics, compared to the ethanolic extract of T. ammi, at
253.04 and 103.96 mg GAE/100 g, respectively. The nanosuspension showed a total flavonoid content (TFC) of 157.06, and the extract contained 168.28 mg CE/100
g TFCs, respectively. The nanosuspension showed a maximum free radical activity
of 14.9%, and the extract showed 43% accordingly. Moreover, according to
statistical analysis, the TPC, TFC, and DPPH tests indicated extremely
significant differences (p
Treatments | Antioxidant profile | Antidiabetic profile (%) | Biofilm inhibition (%) | Hemolysis (%) | ||||
TPC | TFC | DPPH | Glycation inhibition | EC | SA | |||
NS | 253.04 | 157.06 | 14.9 | 25.35 |
34.6 |
13.75 |
No Activity | 22.73 |
E | 103.96 | 168.28 | 43 | 30.91 |
45.67 |
36.91 |
No Activity | 22.69 |
Control | 750.87 | 244.44 | 90 | 56.91333 |
82.53 |
0.121 |
0.119 |
90 |
* Results are represented as a percentage or as the mean and standard deviation of measurements taken in triplicate. NS, T. ammi nanosuspension; E, T. ammi extract; EC, Escherichia coli; SA, Staphylococcus aureus; TPC, total phenolic contents; TFC, total flavonoid content; DPPH, 2,2-diphenyl l-picrylhydrazyl. Ciprofloxacin (antimicrobial assay). Metformin (antiglycation assay). BHT (butylated hydroxytoluene).
Recently, two bacterial strains, i.e., E. coli and S. aureus,
were tested against the nanosuspension and ethanolic extracts of T. ammi
seeds, and the ethanolic extract and nanosuspension exhibited amazing biofilm
inhibition, either by removing the biofilm or lowering biofilm formation against
E. coli. The ethanolic extract exhibited an inhibition of 36.91
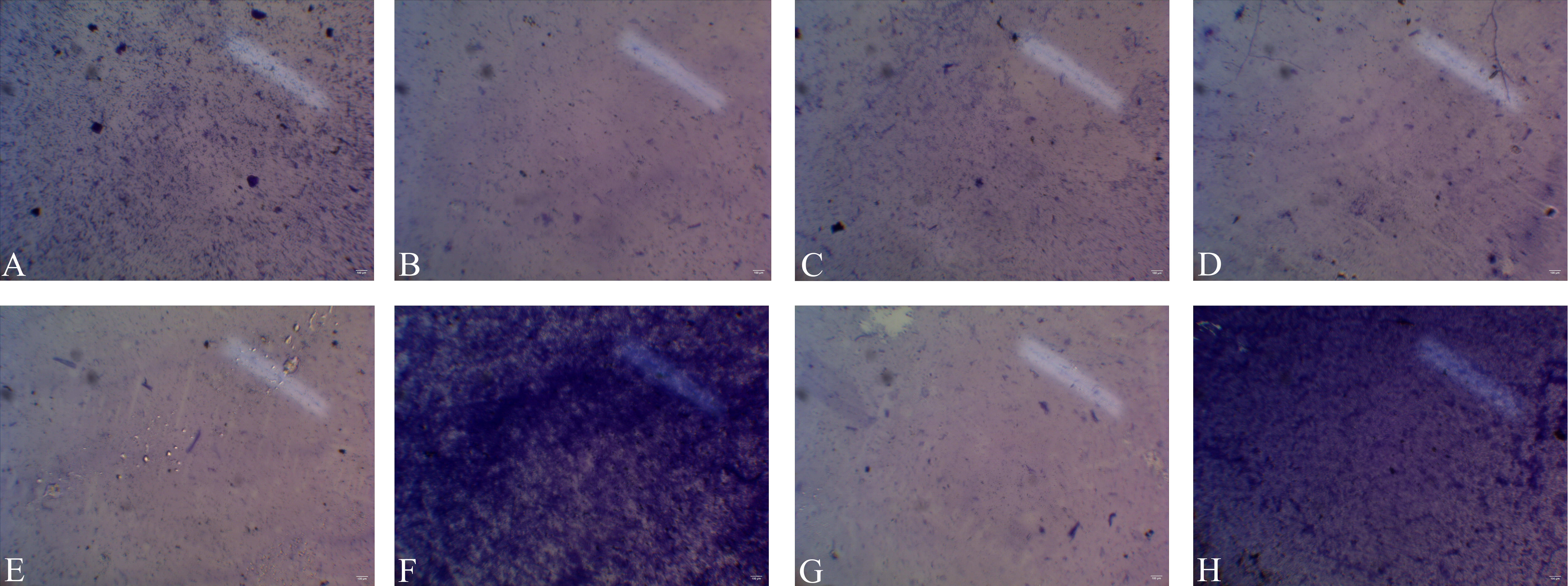
Qualitative biofilm inhibition. (A) inhibition of E. coli by extract fraction (min). (B) Inhibition of E. coli by nanosuspension fraction (max). (C) Inhibition of S. aureus by extract fraction (min). (D) Inhibition of S. aureus by nanosuspension fraction (max). (E) Inhibition of E. coli by positive control. (F) Inhibition of E. coli by negative control. (G) Inhibition of S. aureus by positive control. (H) Inhibition of S. aureus by negative control.
Table 1 shows that the T. ammi nanosuspension exhibited 22.73
The high flavonoid content and significant antioxidant capabilities of the seeds
contribute to the antiglycation effect of the T. ammi nanosuspension.
The nanosuspension showed an antiglycation activity of 25.35
The results showed that the nanosuspension only inhibited 34.6
The structural characterization of the T. ammi seed extracts, as well as the identification of the bioactive functional groups present in the sample, were performed by HPLC and FTIR.
Fig. 2 shows the infrared spectroscopy analysis of the chemical compounds, such
as acids, polyphenols, and a variety of other known and unknown moieties
identified in the test sample. Table 2 shows the absorption values of different
functional groups predicted to be present in the powder of the T. ammi
seeds by FTIR. Alcohols were detected because of a prominent peak at 3281
cm
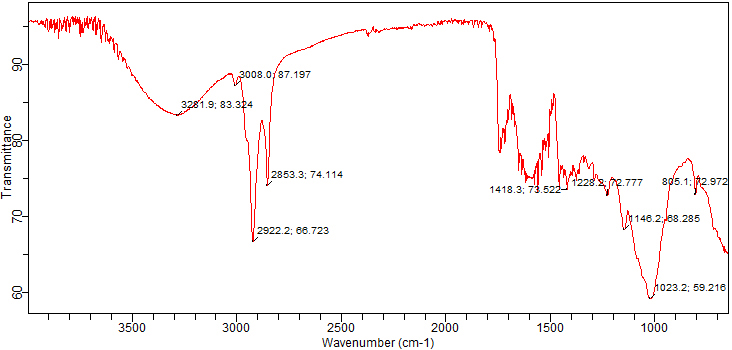
Fourier-transform infrared spectroscopy (FTIR) chromatogram of powdered T. ammi seeds.
Sr. No. | Peak Indemnification | Characteristic Absorption | Identified Functional Groups | Compounds Class |
1 | High | 3281 | O-H stretching | Alcohol |
2 | High | 3008 | O-H stretching | Alcohol |
3 | High | 2922 | N-H stretching | Amines |
5 | Medium | 1418 | O-H stretching | Alcohol |
6 | Medium | 1228 | C-O stretching | Alkyl aryl ether |
7 | Medium | 1146 | C-O stretching | Aliphatic ether |
8 | Low | 1023 | C-N stretching | Amine |
Sr. No., Serial Number.
Fig. 3 shows the bioactive compounds that were identified through HPLC analysis. Kaempferol and sinapic acid were found in T. ammi ethanolic seed extracts in various amounts at 11.5 ppm and 12.3 ppm, respectively.
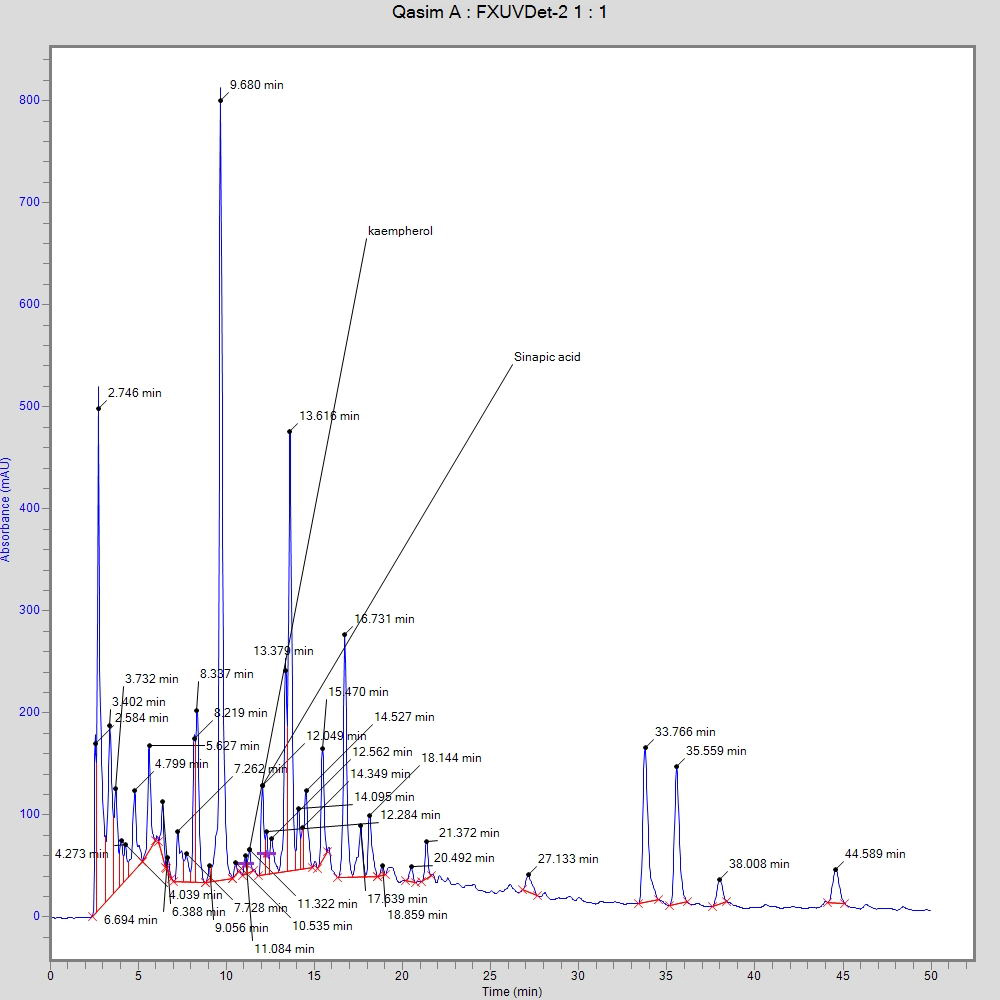
High-performance liquid chromatography (HPLC) chromatogram of T. ammi demonstrating the bioactive compounds.
T. ammi nanosuspension extracts contain different proportions of
phenolic and flavonoid compounds [32]. Our findings agreed with the previous
study, which reported that T. ammi extracts contain more flavonoids than
nanosuspensions, whereas T. ammi nanosuspensions possess higher phenolic
contents [33]. Another study investigated the ethanolic extract and
nanosuspension of T. ammi at higher concentrations (50 g/mL); the
acetone extract of T. ammi had 99% free radical scavenging, which is quite close to normal rutin (99.12%). Previous
studies revealed that T. ammi extracts with different
proportions of DPPH free radical scavenging potentials followed as such: acetone
Different studies revealed the presence of antioxidants in T. ammi
nanosuspensions, which is consistent with our findings, whereby a study reported
that the antioxidants compounds in the ethanolic extract of T. ammi
showed maximum free radical scavenging activity of 64.40%, compared to the
scavenging activity identified in leaves [35]. The current study also agreed with
another study, which revealed that nanosuspensions of T. ammi have a
maximum radical scavenging activity of 43% [36]. Previous studies reported that
the extract of T. ammi nanosuspensions contained antioxidants like
p-pinene, p-cymene, and
Previous studies reported similar findings to our study, revealing nanosuspensions of T. ammi, biogenic selenium nanoparticles (SeNPs), and testing for their toxicological and therapeutic potential in collagen-induced arthritic animals. Another study reported that the tested doses of the SeNPs had no notable deleterious effects on the liver, kidney, spleen, or serum biochemical markers in healthy mice and that less toxicity was induced by the SeNPs, which lessened the severity of the condition [15]. Another study reported that selenium-based bioengineered nanosuspensions of T. ammi combined with TAE extract significantly improved their therapeutic efficacy by up to 90% [35]. Another study reported that the administration of biogenic SeNPs of T. ammi into the liver tissues boosted the catalase activity to some amount [14].
Our research aligns with other prior studies, which also observed the inhibitory effects of T. ammi seed nanosuspensions on biofilm formation and the susceptibility of three distinct bacterial strains to foodborne pathogens. This reinforces the effectiveness of T. ammi seeds in preventing biofilm formation [36]. Another study reported on the efficacy of gold nanoparticles made from T. ammi extracts (TA-AuNPs) against drug-resistant biofilms and found that NPs inhibited the formation of biofilms and the growth of bacterial pathogens, such as Enterococcus faecalis, Staphylococcus aureus, Staphylococcus epidermidis, Streptococcus viridans, E. coli, Klebsiella pneumoniae, Proteus mirabilis, and Pseudomonas aeruginosa [38]. This study revealed that nanosuspensions acted as environmentally friendly and have strong antibiofilm potential, which can be used in a variety of biomedical applications [35]. A prior study highlighted T. ammi oils as a valuable reservoir of bioactive compounds with potential efficacy against multidrug-resistant and oxidative stress-related ailments. This finding suggests that the application of T. ammi in the food industry holds promise as a natural antioxidant and also in the pharmaceutical sector for the development of innovative antibiotics [39].
Previous studies reported that the antidiabetic activity of T. ammi
extracts containing free amino acids (IC
Another study reported that T. ammi seeds inhibited up to 50% of alpha-amylase activity. However, alpha-amylase activity in the T. ammi seeds was increased with the passage of time and the nature of alpha-polypeptide amylase, as well as the polyphenol concentration of the T. ammi seeds [44] Another study revealed that the T. ammi seeds and combinations of medicinal substances inhibited the alpha-amylase activity by 70%, meaning it could be used as a viable alternative to synthetic diabetic medicines [45]. These studies suggested that T. ammi seeds are a rich source of polyphenols and antioxidants that could be used for diabetes. Further, in vivo studies are needed to investigate the action of novel medicines.
Our findings agreed with a previous study, which reported that T. ammi
nanosuspensions showed a hemolytic potential of 30
Infrared spectroscopy is used to determine the structural properties of sample
materials by identifying their distinct functional groups. Previous studies
investigated the infrared spectra of T. ammi oil and found that the
stretching vibration of the amine group was allocated to a medium-intensity band
at 3429 cm
High-performance liquid chromatography is used to identify and quantify various biologically active functional groups, which are present in the test material [42]. Other studies have reported that the chromatogram created by the HPLC shows the peaks of several bioactive chemicals that are contained in the sample based on their column retention time, such as kaempferol in the seed extracts of T. ammi, yet they do not disclose a quantitative analysis of the phenolic and flavonoid chemicals found in the leaves [49, 50]. Another study reported that the phenolic and flavonoid contents were high in the T. ammi extract [51]. These studies suggested that T. ammi nanosuspensions have a diverse nature of phenolic and flavonoids, which could be used in pharmaceutical industries.
This study reflects the cost-effective synthesis and enhanced bioactivities of
freshly prepared T. ammi nanosuspensions. Biochemical characterization
analysis was performed by HPLC and FTIR and revealed the presence of bioactive
compounds: chlorogenic acid, gallic acid, kaempferol, alcohols, and amines. The
nanosuspension showed a higher quantity of TPCs, compared to the ethanolic
extract of T. ammi, at 253.04 and 103.96 mg GAE/100 g, respectively. At
the same time, the nanosuspension contains TFCs at 157.06, while the extract
contains 168.28 mg CE/100 g TFCs. Nanosuspensions demonstrated a maximum free
radical scavenging activity of 14.9% and an antibacterial activity
against E. coli of 3.75
The datasets used/or analyzed during the current study are available from the corresponding author upon reasonable request.
RJ and TA—designed the research study. RJ and TA—performed the research. FH—provided help and advice on experimentation. RJ, MSS, SD, MJA, TAA, MN and FH—visualization, acquiring, analyzing, or interpreting data from the reviewed literature. MJA, TA, MN and FH—wrote the manuscript. SD, TAA, MSS and MJA review and finalize the manuscript. All authors contributed to editorial changes in the manuscript. All authors read and approved the final manuscript. All authors have participated sufficiently in the work and agreed to be accountable for all aspects of the work.
Trachyspermum ammi (Ajwain) seeds were used in this study. Verified by Dr. Farooq Ahmad University of Agriculture Faisalabad Pakistan.
We thanked the administration of the University of Agriculture Faisalabad for their continuous support. This project was supported by Researchers Supporting Project number (RSP2023R230) King Saud University, Riyadh, Saudi Arabia.
This project was supported by Researchers Supporting Project number (RSP2023R230) King Saud University, Riyadh, Saudi Arabia.
The authors declare no conflict of interest.
Publisher’s Note: IMR Press stays neutral with regard to jurisdictional claims in published maps and institutional affiliations.