- Academic Editor
Background: The brain is one of the most vulnerable metastasis sites in
lung cancer; approximately 40–50% of lung cancer patients develop brain
metastasis during the disease course, contributing to the poor prognosis and high
mortality of lung cancer patients. Therefore, it is important to clarify the
molecular mechanism underlying brain metastasis of lung cancer for improving the
overall survival of lung cancer patients. The present study aimed to investigate
the potential role of blood-brain barrier (BBB) permeability in the development
of brain metastasis of lung cancer and explore the effect of aspirin in an
in-vitro BBB model. Methods: An in-vitro BBB model was
established. The expression of heat shock protein 70 (HSP 70), zonula occludens-1
(ZO-1), and occludin in rat brain microvascular endothelial cells was detected
using Western blot at different time points following the administration of
aspirin. Results: HSP70, ZO-1, and occludin expressions did not show
significant changes before aspirin administration, but increased noticeably after
aspirin administration. Tumor necrosis factor-
Brain metastasis is accepted as the most common fatal complication of lung cancer. Approximately 10–25% of lung cancer cases develop brain metastasis at the initial diagnosis, and 40–50% of lung cancer cases may experience brain metastasis during the disease course [1]; this leads to the dismal prognosis and high mortality of lung cancer patients. Therefore, it is important to determine the molecular mechanism underlying brain metastasis of lung cancer, thus contributing to improving the overall survival of lung cancer patients [2, 3, 4].
Aspirin is a nonsteroidal anti-inflammatory drug, which is commonly used to relieve pain, fever, and peripheral inflammation in clinics [5]. Emerging evidence has also indicated the potent role of aspirin in tumor prevention and treatment [6]. The combination of aspirin and antineoplastic agents has been reported to exhibit a potent anti-tumor activity [7], suggesting an anti-tumor effect of aspirin at the cell level.
The association between aspirin and lung cancer has been documented since 1989 [8]. Accumulating studies have demonstrated the anti-tumor effects of aspirin. For example, aspirin inhibits the proliferation of lung cancer cells by inhibiting cyclooxygenase activity [9]. Specific doses of aspirin can inhibit the proliferation and induce the apoptosis of human lung adenocarcinoma cells and small cell lung cancer cells; aspirin, in combination with radiotherapy, can exhibit a synergistic action. In addition to the anti-tumor effects, aspirin also enhances the therapeutic effect of chemotherapeutic drugs in lung cancer [10]. A previous study has shown that low-dose aspirin is associated with small tumor extents and few metastatic complications in lung cancer patients [11]. Therefore, aspirin is a promising agent for lung cancer. However, the underlying action mechanism of aspirin on lung cancer has not been thoroughly elucidated.
NCI-H209 lung cancer cells (from FuHeng Cell Center, Shanghai, China) were
incubated in Dulbecco’s modified Eagle’s medium (DMEM;
Gibco; Thermo Fisher Scientific, Inc., Waltham, MA, USA)
supplemented with 15% heat-inactivated fetal bovine serum
(FBS; 10270-106, HyClone; Thermo Fisher),
100 U/mL penicillin, and 100 µg/mL streptomycin under standard culture
conditions. When the cells reached confluence, the medium was aspirated and fresh
serum-free medium was added for further incubation (12 h). Subsequently, the
cells were washed once with sterile phosphate-buffered saline (PBS) and then
cultured with a fresh serum-free medium. Next, the cells were treated with
aspirin (Sigma-Aldrich, St. Louis, MO, USA; Merck KGaA) for 10, 30, 60, 120, 180,
and 240 min. All cell lines were validated by STR profiling and tested negative for mycoplasma. Cells were all cultured in a humidified incubator at 37 °C and 5% CO
TNF-
Primary rat brain microvascular endothelial cells (rBMECs) were isolated and
cultured as previously described [12]. The in-vitro BBB model was
established as described by Hurst and Fritz [13]. Initially,
astrocytes (Cell Resource Center, IBMS,
CAMS/PUMC) were seeded (1
The status of BBB permeability was quantitatively evaluated by determining HRP
extravasation.
Lung
cancer cell suspensions
(5
NCI-H209 cells were resuspended in DMEM containing 15% FBS to obtain a
concentration of 1
The effects of aspirin on the level of HSP70, zonula occludens-1 (ZO-1), and occludin proteins in rBMECs were analyzed using the Western blot assay. The confluent rBMECs were collected at different time points and washed with Dulbecco’s PBS containing 0.1 mM ethylenediamine tetraacetic acid (EDTA) in the absence of calcium and magnesium.
Next, rBMECs were homogenized (4 °C) in 1
mL lysis buffer (2 mM EDTA; 10 mM EGTA; 0.4% NaF; 20 mM Tris-HCl) containing
protease inhibitor (pH = 7.5) to obtain the cell membrane fraction.
The samples were then centrifuged (17,000
The measurement data in normal distribution were described as mean
To examine the effect of aspirin on TNF-
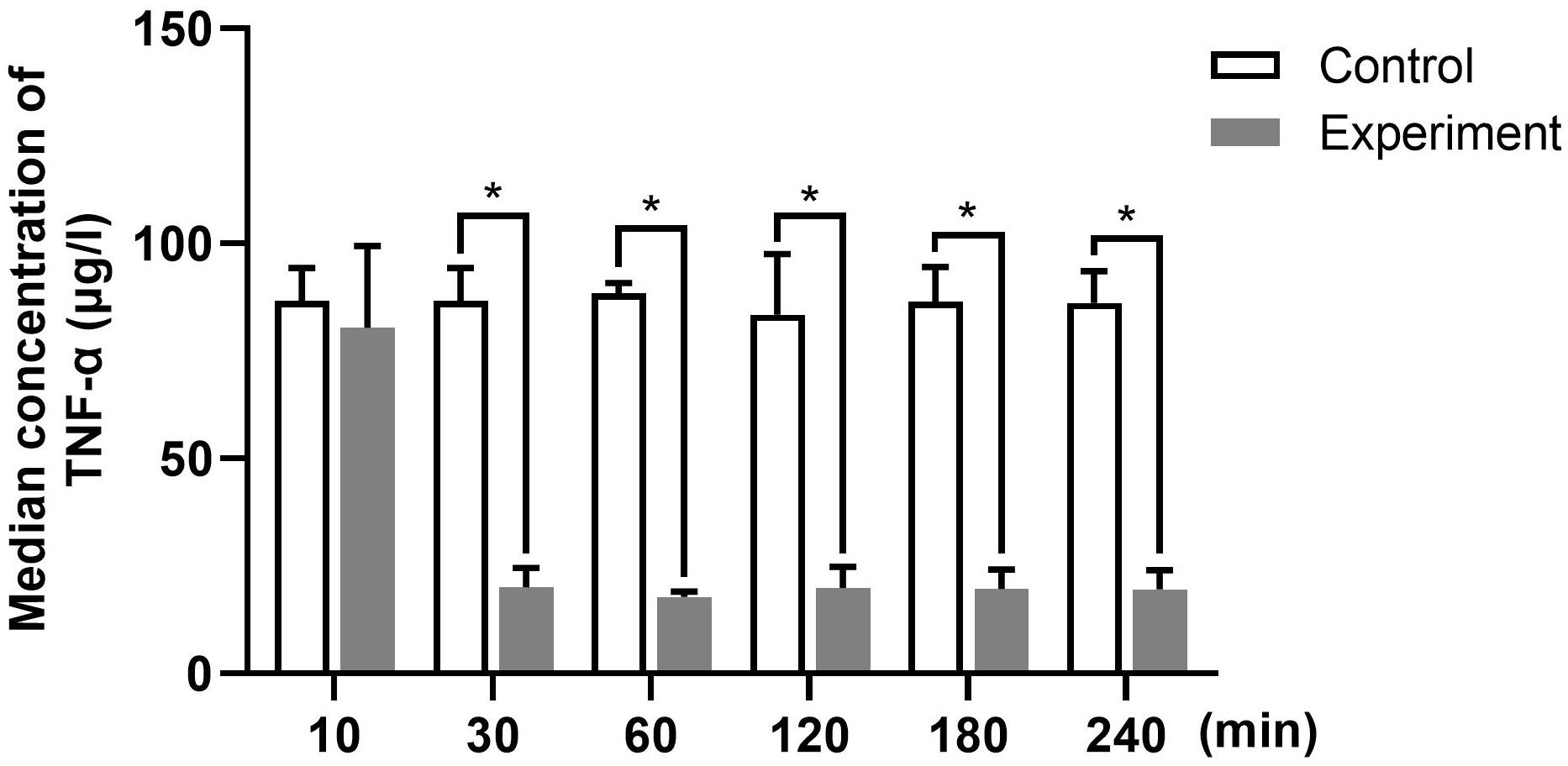
The average concentrations of TNF-
In the present study, the permeability of the in-vitro BBB model was
analyzed by measuring the HRP flux. The average concentrations
of HRP flux at different time points are shown in Fig. 2. We
found that aspirin administration to the rBMEC monolayer on Transwell filters
could decrease HRP leakage. The HRP flux was significantly lower at 60 min after
aspirin administration than that at 10 min (p
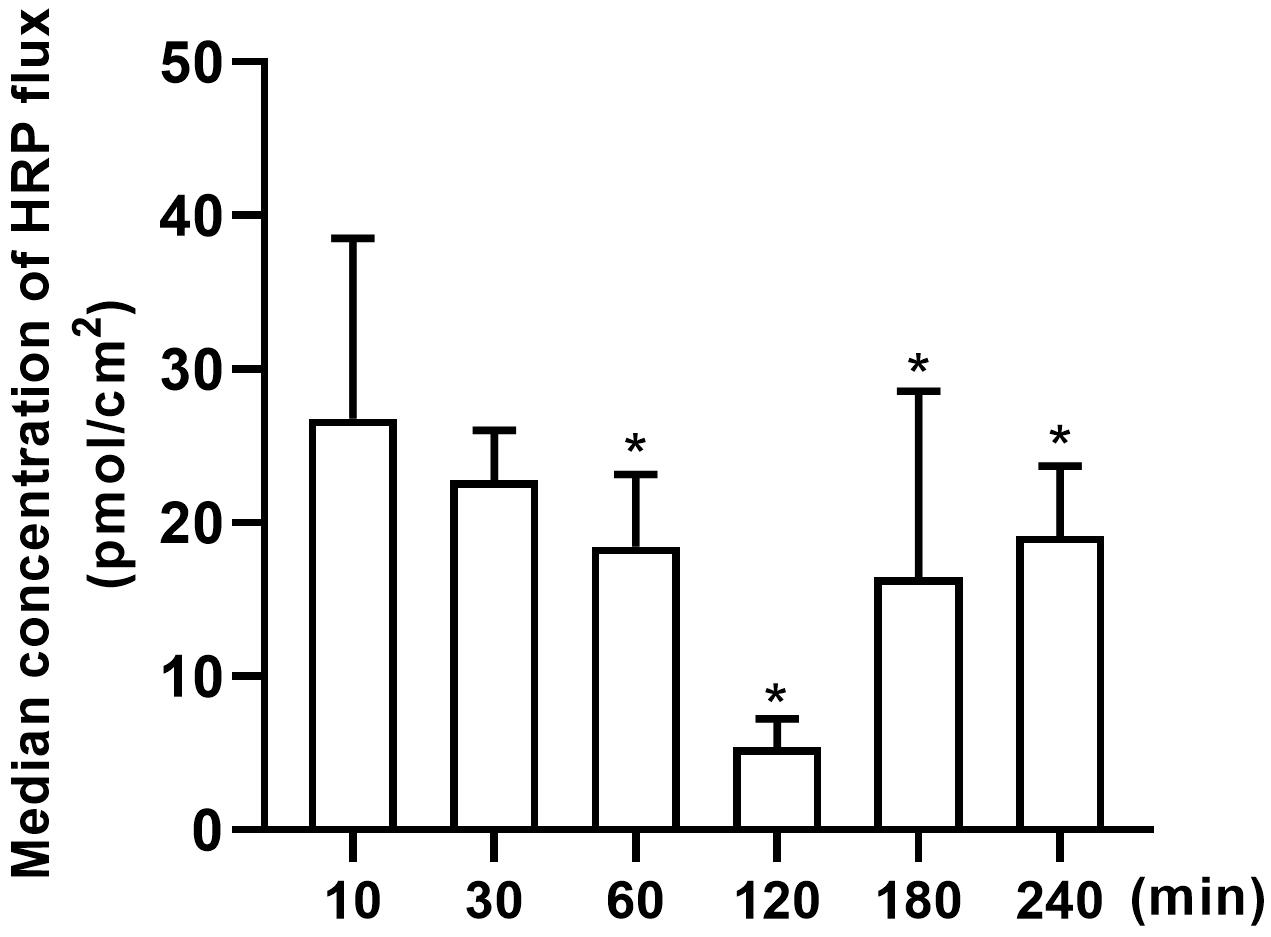
The average concentrations of HRP flux at
different time points were detected. Time course of
aspirin-induced changes in HRP flux of the in-vitro BBB model. At
different time points, rat brain microvascular endothelial cells were treated
with aspirin (8 mM/L) for 10, 30, 60, 120, 180, and 240 min. n = 12. Data are
shown as mean
To examine the effect of aspirin on migration of Lung Cancer Cells,We examined
the migration in each group. The migration of NCI-H209 lung cancer cells treated
with aspirin at different time points was detected.
The
percentage of migrated
cells with aspirin treatment varied at different time points (Fig. 3). Relative
to the 10-min group, other groups showed a 1.8–4.4 times lower percentage of
migrated cells. Further quantitative analysis indicated that the mean value of
migrated cells in the experimental groups was significantly reduced at each time
point (from 10 min to 240 min) compared with the control group (all p
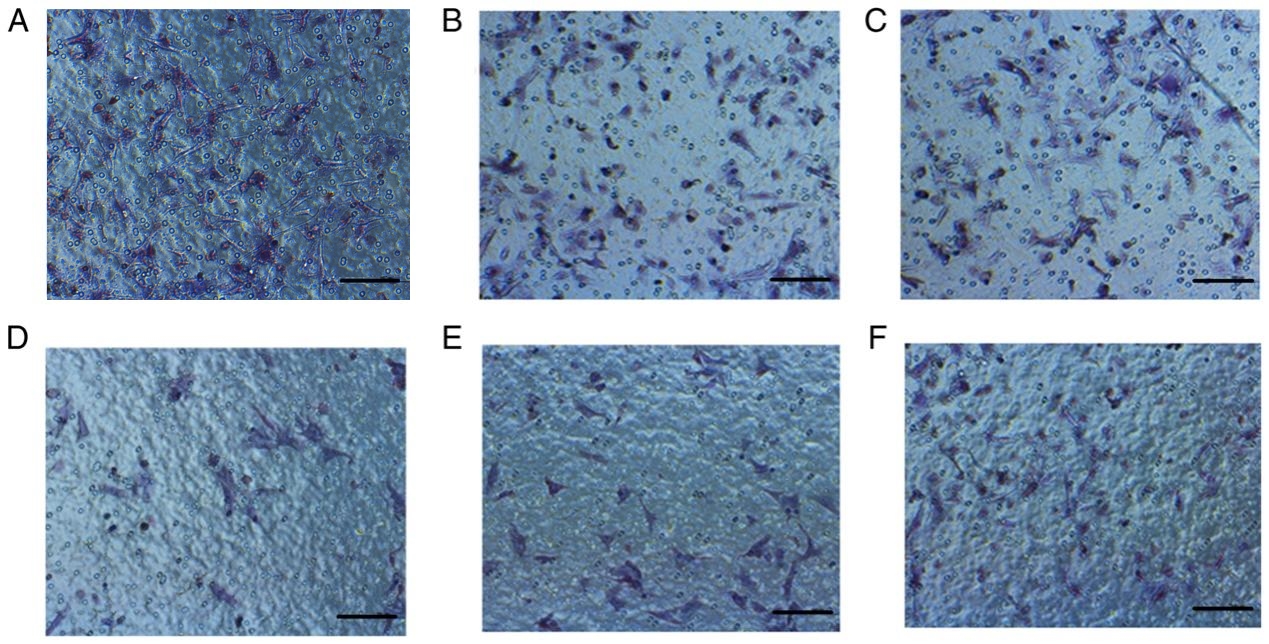
The migration of NCI-H209 lung cancer cells treated with aspirin at different time points was detected. Migration of cells in the (A) 10, (B) 30, (C) 60, (D) 120, (E) 180, and (F) 240 min-groups. n = 12. Scale bars = 100 µm.
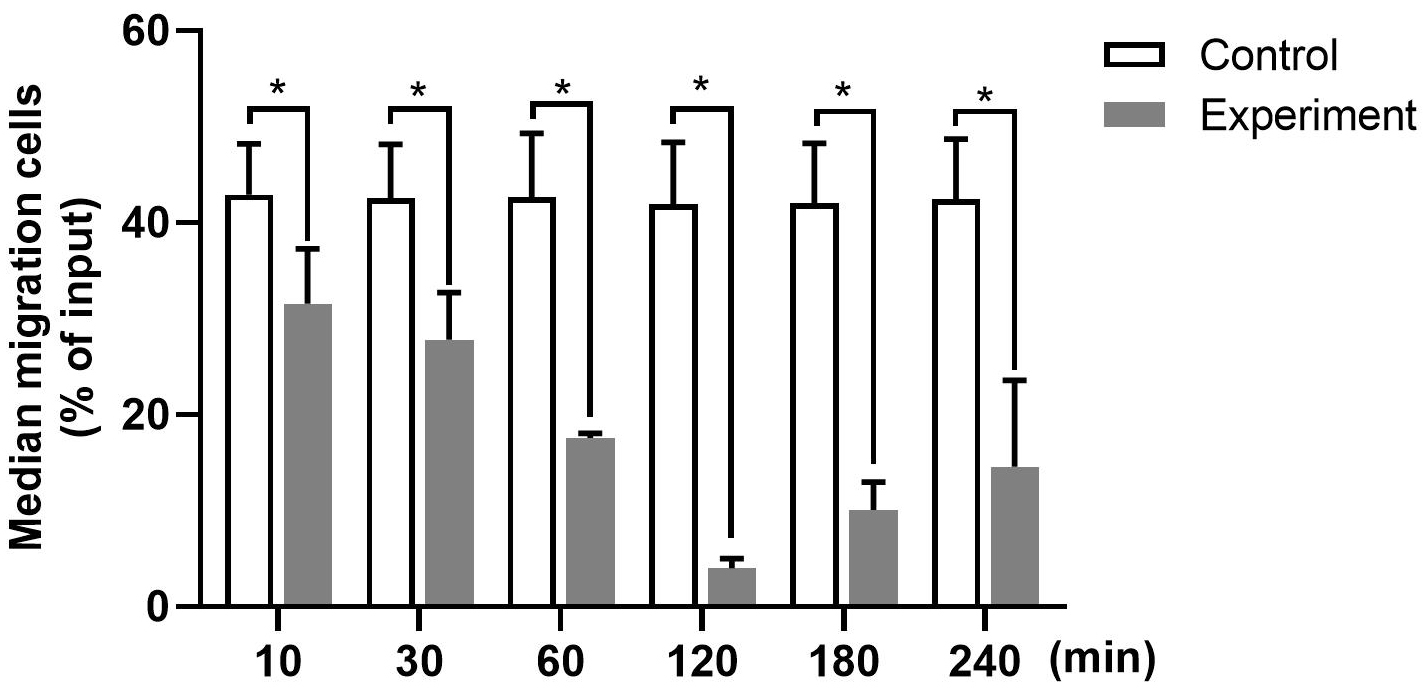
The number of migrated NCI-H209 cells at different time points
was detected. n = 12. Data are shown as mean
The IDV of HSP70 in rBMECs of different groups was detected, and was found to
vary in different lanes (Fig. 5). IDV is the whole optical density value obtained
after computer software measurement. According to Western blot analysis results,
a higher IDV value was associated with a higher protein expression. Compared with
that in the control group, the mean IDV of HSP70 began to
increase significantly 30 min after aspirin administration and reached its peak
at 60 min after aspirin administration (p
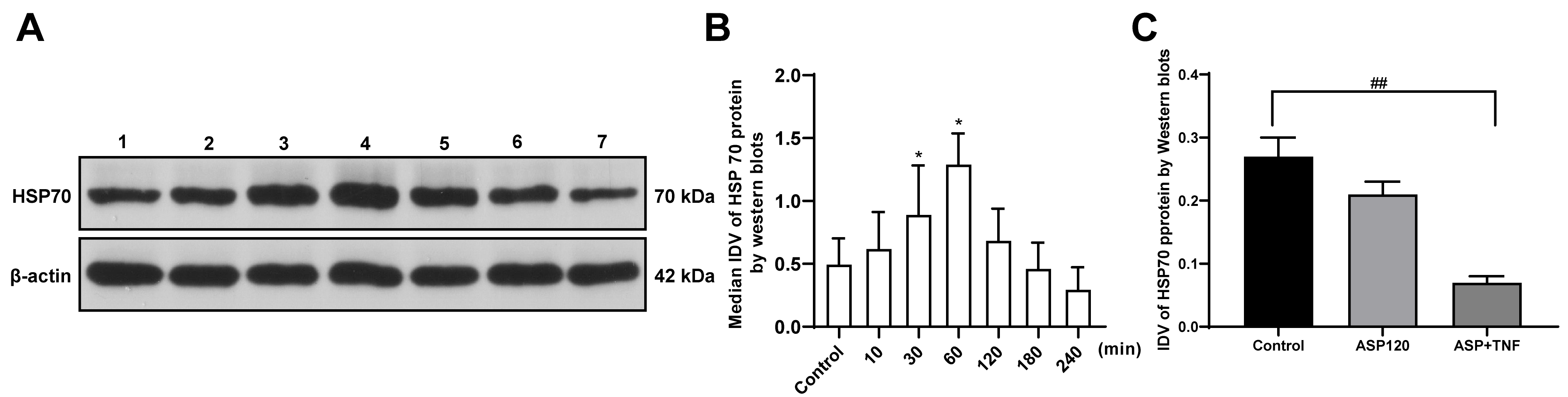
The effects of aspirin on HSP70 protein level in rat brain
microvascular endothelial cells at different time points after treatment were
detected. (A) Lane 1: Control group; lane 2: ASP, 10 min group; lane 3: ASP, 30
min group; lane 4: ASP, 60 min group; lane 5: ASP, 120 min group; lane 6: ASP,
180 min group; lane 7: ASP, 240 min group. Representative Western blots showed
differences in the bands of HSP70. Changes in the relative IDV of HSP70 (B) over
240 min and (C) with ASP and TNF-
The IDV ratios of tight junction proteins ZO-1 and occludin were analyzed using
Western blot (Figs. 6,7). According to the results, the ZO-1 protein level in
rBMECs was significantly upregulated 30 min after aspirin administration,
reaching the highest at 120 min after aspirin administration. The upregulation
trend of ZO-1 in rBMECs continued until 240 min after aspirin treatment (Fig. 6).
The protein level of occludin was noticeably upregulated at 60 min after aspirin
administration, and its highest level was observed at 120 min and lasted until
240 min after the treatment (Fig. 7). Furthermore, 240 min treatment with aspirin
and TNF-
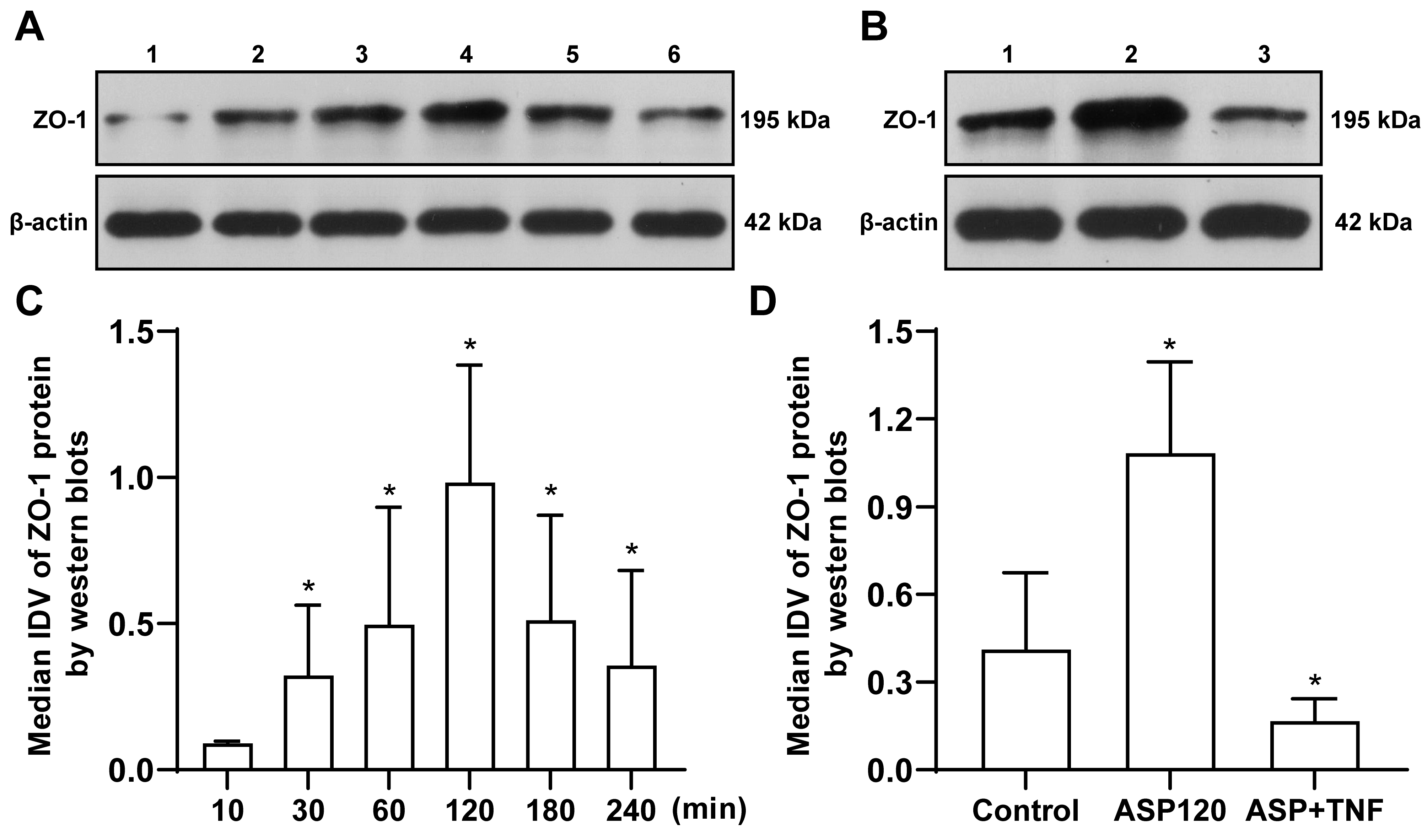
The effects of ASP on ZO-1 protein level in rat brain
microvascular endothelial cells at different time points after treatment were
detected. (A) Lane 1: ASP, 10 min group; lane 2: ASP, 30 min group; lane 3: ASP,
60 min group; lane 4: ASP, 120 min group; lane 5: ASP, 180 min group; and lane 6:
ASP, 240 min group. (B) Lane 1: control group; lane 2: ASP, 120 min group; and
lane 3: ASP + TNF-
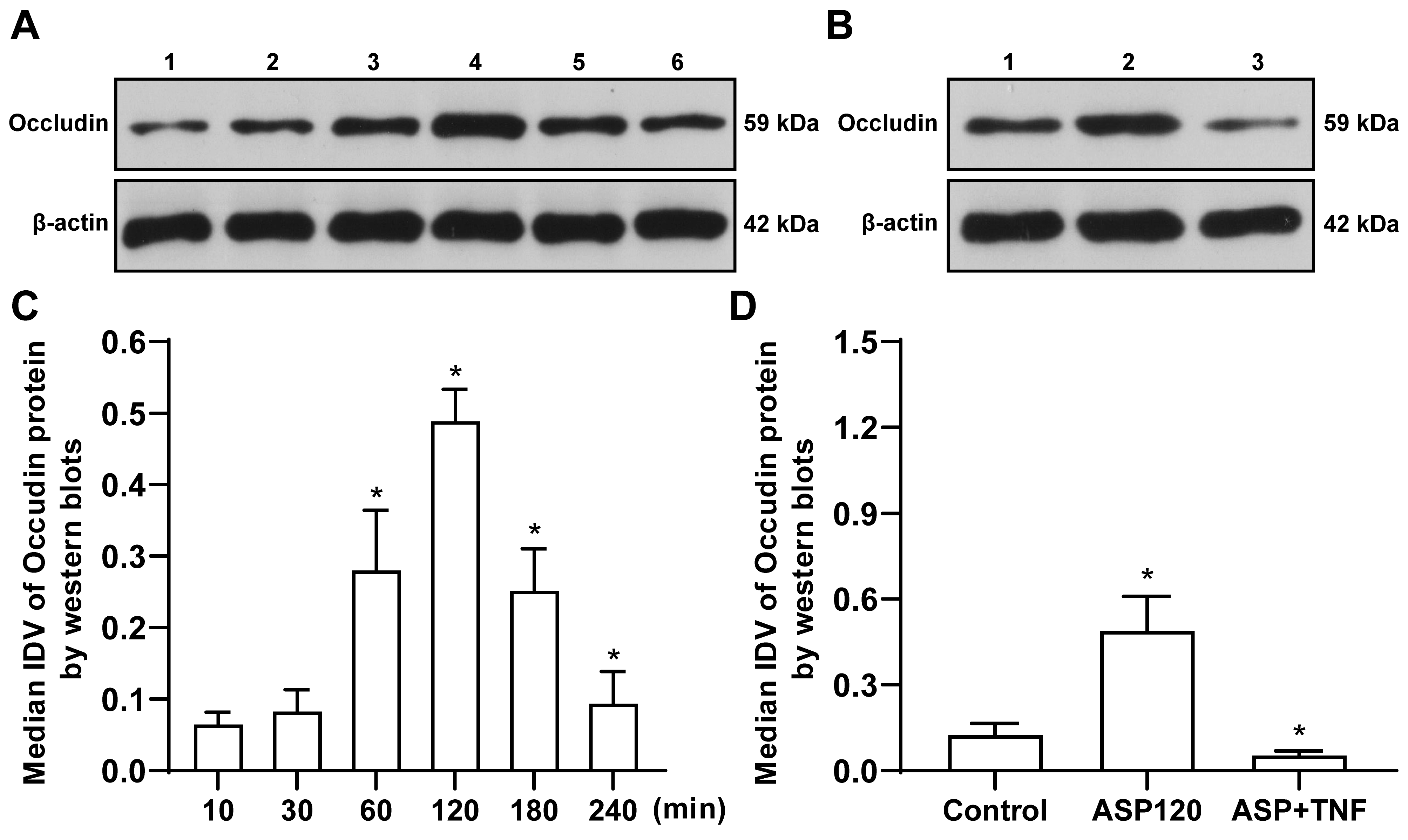
The effects of aspirin on occludin protein level in rat brain
microvascular endothelial cells at different time points after treatment were
detected. (A) Lane 1: ASP, 10 min group; lane 2: ASP, 30 min group; lane 3: ASP,
60 min group; lane 4: ASP, 120 min group; lane 5: ASP, 180 min group; and lane 6:
ASP, 240 min group. (B) Lane 1: control group; lane 2: ASP, 120 min group; and
lane 3: ASP+ TNF-
The BBB is a complex multicellular structure that separates the central nervous system (CNS) from the peripheral blood circulation and protects the CNS from toxins and pathogens, which maintains internal environmental homeostasis and enables the function of neurons [15, 16, 17, 18, 19]. The vast majority of patients with small cell lung cancer are prone to brain metastasis, which can be largely attributed to the increased permeability of the BBB. The tight junctions between BBB endothelial cells construct a robust physical barrier that can control the paracellular passage of molecules [20]. The tight junction complex is composed of different junctional molecules, including occludin, claudins, ZOs, and junctional adhesion molecules [21]. Accumulating studies have provided evidence that dysregulated tight junction proteins can contribute to altered BBB permeability [22, 23]. ZO-1 is a member of the membrane-associated guanylate kinase-like protein family, and its presence at the plasma membrane is closely associated with the integrity of the tight junction [24, 25]. Occludin, a class of transmembrane proteins, is specifically associated with tight junctions, contributing to the function of the intercellular seal [26].
Despite the remarkable progress in the development of in-vitro models of BBB, most studies are limited to simulating the BBB of the human body in-vitro [27, 28, 29]. Moreover, little is known about the factors that maintain the integrity of the BBB and the molecular mechanisms of various structures involved in the BBB formation. In the present study, the average values of multiple samples were obtained to compensate for the deficiencies of the modeling method, which can reduce the possible differences between the in-vitro model of BBB and the in-vivo BBB. Specifically, the in-vitro BBB model was established by the co-culture of astrocytes and rBMECs as previously reported [13]. Astrocytes reside in the abluminal surface of the microvasculature of the BBB, where the cells constitute the major cell population in the neurovascular unit and play an important role in BBB maintenance. As previously evidenced, astrocytes can mediate metastatic cancer growth into the brain parenchyma by regulating matrix metalloproteinases [30]. Accumulating studies have also indicated the critical involvement of pericytes in BBB formation and BB permeability maintenance. However, the in-vitro BBB model in the present study did not contain pericytes and thus the effect of pericytes is not factored in in the present study.
In the present study, the
effect of TNF-
In prior works, aspirin displays notable inhibitory effects on the proliferation
of lung cancer cells in a time- and concentration-dependent manner [34, 35]. In
the present study, after a series of preliminary experiments and dynamic index
monitoring, the optimal dose of aspirin was determined to be 8
mmol/L. The results of this study revealed that lung cancer cells released
TNF-
In conclusion, the underlying molecular mechanism of aspirin reducing BBB
permeability and further inhibiting the brain metastases of lung cancer is
complicated. The results of the present study reveal that aspirin upregulates the
expression of tight junction proteins via the
TNF-
Raw data can be obtained by contacting corresponding author.
LQ accounted for all aspects of the work in ensuring that questions related to the accuracy or integrity of any part of the work. DW, MT and WG performed the experiments and collected data and analyzed the data. DW, MT, WG and JL designed the study and wrote the manuscript. All authors read and approved the final manuscript. All authors contributed to editorial changes in the manuscript. All authors read and approved the final manuscript. All authors have participated sufficiently in the work and agreed to be accountable for all aspects of the work.
Not applicable.
Not applicable.
The present study was supported by the Natural Science Foundation of China (grant no. 81101912), the Science and Technology Project for Colleges and Universities in Hebei Province (grant no. ZD20160082), and the Scientific Research Foundation of Hebei Health Department (grant no. 20170901).
The authors declare no conflict of interest.
Publisher’s Note: IMR Press stays neutral with regard to jurisdictional claims in published maps and institutional affiliations.