- Academic Editor
†These authors contributed equally.
Background: Accumulating evidence suggests that acupuncture may serve as a potent strategy to mitigate the deleterious effects of ischemic stroke on neural tissue. The present investigation delineated the neuroprotective potential of electroacupuncture (EA) administered pre-and post-stroke, with a focus on determining the commonalities and disparities between these two therapeutic approaches in ameliorating ischemic stroke-induced brain injury. The ultimate objective is to inform optimal timing for acupuncture intervention in the clinical management and prevention of stroke. Methods: The extent of cerebral infarction was quantified with 2,3,5-triphenyltetrazolium chloride staining. The integrity of the blood–brain barrier was assessed by evaluating the extravasation of Evans blue (EB) dye, while neurological function was appraised using the Longa neurological scoring system. RNA sequencing was employed to examine the transcriptomic landscape of ischemic brain tissue, with subsequent bioinformatics annotation of the sequencing data facilitated by Metascape. Results: (1) A notable decrease in the ischemic infarct volume was observed in both the EA-preconditioned plus middle cerebral artery occlusion (MCAO), EA-preconditioned plus middle cerebral artery occlusion (EAM) and MCAO plus EA-treated (MEA) groups, compared to the MCAO group. Furthermore, the decreased leakage of EB and reduction in neurological function impairment scores were evident in the EAM and MEA groups compared with the MCAO group. (2) Relative to the Sham group, the MCAO group exhibited a total of 4798 differentially expressed genes (DEGs), with 67.84% demonstrating an expression fold change (FC) greater than 1.5, and 34.16% exceeding a FC of 2. The EAM and MEA groups displayed 4020 and 1956 DEGs, respectively, compared to the MCAO group. In both groups, more than 55% of DEGs showed an expression FC surpassing 1.5, whereas only approximately 10% exhibited a change greater than 2-fold. Remarkably, EA preconditioning and EA treatment resulted in the reversal of 18.72% and 28.91% of DEGs, respectively, in the MCAO group. (3) The DEGs upregulated in response to ischemic stroke were predominantly implicated in immune inflammatory processes and cellular apoptosis, whereas the downregulated DEGs were associated with neurogenesis and neuronal signal transduction. The MEA-induced upregulated DEGs were primarily involved in neural transmission and metabolic processes, whereas the downregulated DEGs were linked to excessive inflammatory responses to physical and chemical stimuli, as well as cell matrix adhesion chemotaxis. In the context of EAM, the upregulated DEGs were chiefly related to protein biosynthesis, and energy and metabolic processes, whereas the downregulated genes were connected to gene transcriptional activity, synaptic function, and neuronal architecture. Conclusions: Both preconditioning and post-event treatment with acupuncture demonstrated efficacy in mitigating pathological damage to brain tissue in a rat model of ischemic stroke, albeit with some divergences in their gene targets. The integration of EA preconditioning and treatment may potentially confer enhanced neuroprotection in the clinical management of stroke patients.
Stroke is a leading cause of death and disability worldwide [1, 2]. In most cases, acute stroke results from the occlusion of a supplying arterial vessel, whereas vascular rupture accompanied by hemorrhage is less common, accounting for only about 15% of cases [3]. Acupuncture, characterized by its affordability, convenience, and minimal adverse effects, has emerged as a promising treatment option for stroke. A wealth of clinical and experimental data have substantiated the role of post-stroke acupuncture treatment in orchestrating multilevel regulation via intricate mechanisms to combat cerebral ischemia [4]. Specifically, electroacupuncture (EA) has been demonstrated to alleviate nitro/oxidative stress-induced mitochondrial dysfunction and inhibit the accrual of damaged mitochondria via Pink1/Parkin-mediated mitophagy clearance, thereby protecting cells from neuronal damage consequent to cerebral ischemia-reperfusion (I/R) [5]. Zhang and colleagues [6, 7] revealed that EA treatment can activate the PI3K/AKT/mTOR signaling pathway during the acute phase post-ischemic stroke, which subsequently suppresses neuronal apoptosis and autophagy, thereby diminishing cerebral I/R injury. Jittiwat [8] reported that laser acupuncture at Baihui acupoint (GV20) can significantly reduce brain infarct volume and malondialdehyde concentrations, while enhancing the activities of catalase, glutathione peroxidase, and superoxide_dismutase in rats with cerebral ischemia. A prospective investigation indicated that acupuncture treatment can improve clinical outcome and cognitive function in patients suffering from cerebral ischemic stroke, potentially attributable to its modulatory effects on immune responses and inflammation in vivo [9]. Moreover, a controlled clinical trial demonstrated that the integration of acupuncture with rehabilitation therapy can effectively improve the neurological function, prognosis, and patient quality of life [10]. Our previous studies demonstrated that post-stroke EA treatment can modulate the equilibrium of regulatory T cells/gamma delta T cells, thereby ameliorating inflammation-induced damage to the brain and intestine [11, 12]. Additionally, mounting evidence suggests that acupuncture preconditioning confers neuroprotective benefits by enhancing ischemic tolerance in experimental models and attenuating pathological brain damage subsequent to ischemic stroke [13, 14, 15, 16, 17]. Lin and colleagues revealed that EA induces a reversible, frequency-dependent alteration of blood–brain barrier (BBB) permeability in rats, which may be related to disrupting interendothelial tight junctions caused by the activation of neurons releasing substance P, and a decrease in zonula occludens 1 and occludin expression, thereby providing new strategies for delivering therapeutics to the central nervous system [18]. Our earlier work also demonstrated that EA preconditioning can partially rectify or mitigate aberrant gene expression in the ipsilateral ischemic brain [19]. However, to date, little attention has been paid to comparing the neuroprotective effects of EA intervention administered pre- and post-stroke [20]. Moreover, no current research has been conducted to determine the optimal timing for acupuncture intervention to confer benefits to stroke patients in the acute phase.
To bridge this knowledge gap, based on the comparation of anti-ischemic efficacy, we utilized RNA sequencing (RNA-Seq) technology to acquire transcriptomic data from the cerebral ischemic regions of Sprague-Dawley (SD) rats following EA preconditioning and EA treatment for bioinformatics analysis. Our aim is to provide an experimental basis for the clinical application of acupuncture in the prevention and treatment of stroke.
Male adult SD rats, provided by Xipu Bikai Laboratory Animal Co., Ltd. (Shanghai, China), were used in this experiment. Animals were housed under a 12 h light/12 h dark cycle and had free access to food and water. All experiments followed the Guidance on the Good Treatment of Laboratory Animals issued by the Ministry of Science and Technology of China.
All male adult SD rats (260–280 g) were adaptively bred for 1 week and then randomly divided into four groups (Fig. 1A): sham surgery (Sham), middle cerebral artery occlusion (MCAO), EA-preconditioned plus MCAO (EAM), and MCAO plus EA-treated (MEA) groups. In the MCAO, EAM, and MEA groups, cerebral I/R injury was induced by MCAO experiments in the rats. During the operation, rats were deeply anesthetized by inhalation of 5% isoflurane (R510-22-10; Reward, Shenzhen, China) and maintained under anesthesia with 2% isoflurane in a mixture of 70% nitrous oxide and 30% oxygen. To maintain the rat’s body temperature at 37 °C, we used a heating pad during and after the operation. After 2 h of MCAO, the intraluminal suture (JiaLing, Guangzhou, China) was withdrawn to induce post-ischemic reperfusion. The rats without significant neurological deficits, intraoperative hemorrhage, subarachnoid hemorrhage, or thrombosis of the circle of Willis were excluded. For the Sham group, the external carotid artery was isolated and ligated, while the suture was not inserted.
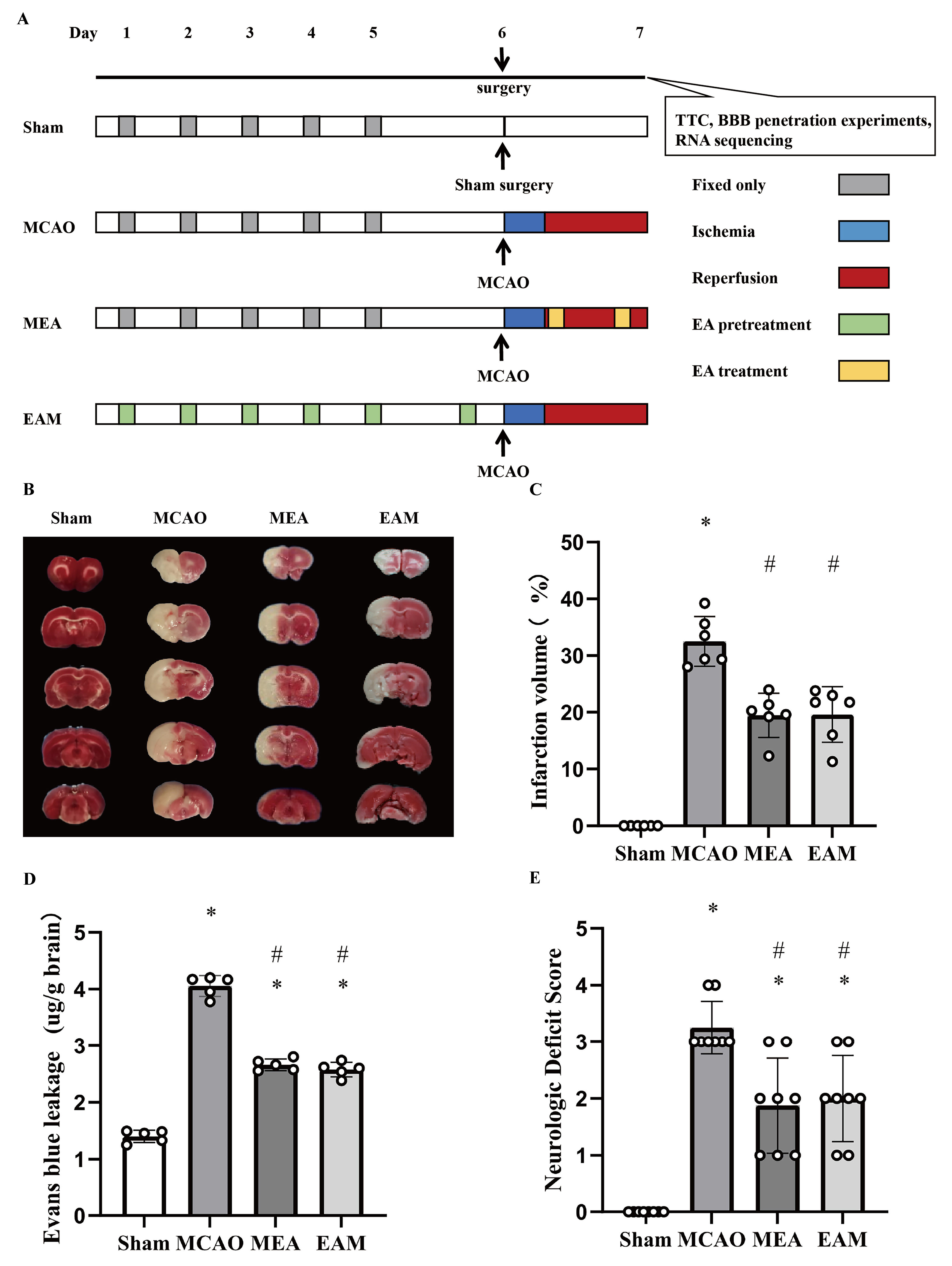
Electroacupuncture Preconditioning and Treatment Both Reduce Cerebral Ischemia-Reperfusion Injury Stroke-Induced Rats. (A) Animal group and experimental protocol. All rats were randomly
divided into four groups: sham surgery (Sham), middle cerebral artery occlusion
(MCAO), EA-preconditioned plus MCAO (EAM), and MCAO plus EA-treated (MEA) groups.
In brief, cerebral ischemia-reperfusion (I/R) injury was induced by MCAO
experiments in rats in the MCAO, EAM, and MEA groups. (B) Representative image of
TTC staining. (C) Quantitative results of TTC staining. The ischemic area was
evaluated by calculating the hemispheric lesion area with ImageJ software, and
the relative infarction volume percentage is presented as a bar graph. Data are
presented as the mean
The Baihui acupoint (GV20), located at the midpoint of the connecting line between the auricular apices, was chosen for both EA preconditioning and treatment. In the MEA group, rats were subjected to EA treatment at the GV20 acupoint for 30 min per session, with a frequency of 2/15 Hz and an intensity level of 1 mA, which was stimulated with a stimulus isolation unit (WQ1002F; HanAcuten, Beijing, China). EA treatment at the GV20 acupoint was performed at 1 min after reperfusion and again at 2.5 h before sacrifice (all rats were sacrificed at 24 h after I/R or sham surgery), for a total of two times. In the EAM group, rats received EA preconditioning at the GV20 acupoint for 30 min per day for six consecutive days, with the same parameters as those in the MEA group. MCAO surgery was performed 2 h after the sixth EA pretreatment. Rats in the Sham and MCAO groups were immobilized in the same manner for 30 min without EA stimulation.
Neurologic function was assessed according to the Longa neurologic score, where a score of 0 indicates no neurologic deficit, 1 indicates a mild focal neurologic deficit (failure to extend left forepaw fully), 2 indicates a moderate focal neurologic deficit (circling to the left), 3 indicates a severe focal deficit (falling to the left), and 4 indicates rats that did not walk spontaneously and had a depressed level of consciousness. The first neurologic function assessment was performed at 2 h after ischemia, and the second evaluation was performed at 24 h after reperfusion.
2,3,5-triphenyl tetrazolium chloride (TTC; T8877; Sigma, St. Louis, MO, USA) was
used to visualize and measure the ischemia infarct volume. First, rat brains were
isolated and frozen at –20 °C for 30 min after 24 h of reperfusion. Then the
brains were sliced into coronal sections that were approximately 2 mm thick. TTC
staining was performed on brain slices using 2% TTC (1 g/50 mL) at 37 °C for 30
min, and the sections were fixed in 4% paraformaldehyde. The total infarcted
area (IVA) and total area (TA) on the back side of five coronal slices per rat
were evaluated using ImageJ software (Version 1.8.0; ImageJ, MD, United States).
The relative infarction volume percentage (RIVP) was calculated as: RIVP = IVA/TA
BBB permeability was evaluated by measuring the extravasation of Evans blue (EB). Five rats were randomly selected from each group and injected with 2% EB (E2129; Sigma) solution (3 mL/kg) via the femoral artery at 1 h prior to sacrifice. Then the rats were euthanized by intraperitoneal injection of an overdose of sodium pentobarbital and immediately perfused with saline through the left ventricle until colorless fluid was obtained from the right atrium. The left and right brains were quickly removed and weighed. Then the appropriate amount of formamide (3 mL/600 mg) was added separately, and the samples were soaked in water at 60 °C overnight. After 24 h, the supernatant was extracted by centrifugation at 1000 rpm (radius 10 cm) for 5 min at room temperature. Subsequently, different concentrations of EB standard solution and supernatant from each group were added to a 96-well enzyme plate with three replicate wells for both standards and samples. The EB content was finally determined at 632 nm using a microplate reader (Synergy H1; BioTek, Winooski, VT, USA). A standard curve was constructed using gradient concentrations of EB to quantify the amount of EB retained in the hemisphere.
Total RNA was extracted from ischemic brain tissue with TRIzol reagent
(15596018; Invitrogen). The RNA concentration was measured with the Qubit 1.2
fluorometric analyzer (Invitrogen; Carlsbad, CA, USA) using the Qubit RNA BR
Assay Kit (Q10211, Invitrogen). RNA quality was assessed using the Agilent 2100
Bioanalyzer System (Agilent Technologies, Inc., Santa Clara, CA, USA) with an RNA
integrity number of at least 8.0. Then RNA samples (3 replicates for each group,
totaling 12 samples) were processed with the TruSeq RNA Kit (15025062; Illumina)
to create DNA libraries, which were cluster-generated using cBOT recombinant
hybridization plates and sequenced on the Illumina HiSeq 2000 System (Illumina,
San Diego, CA, USA) using the TruSeq SBS kit v3 (15021668; Illumina). The
resulting FASTQ files were extracted using Illumina’s CASAVA software (v.1.8.2;
Illumina), and the sequencing data were assembled and quantified against the rat
reference genome (UCSC Rn4) using the TopHat program and DESeq2 program, followed
by analyses of the differentially expressed genes (DEGs) with the Cuffdiff
program. Gene annotation, signaling pathway, functional analysis, and
protein–protein interaction (PPI) network interaction analyses were performed
with ClusterProfiler and Metascape. Only DEGs with p
First, we examined the neuroprotective effects of EA pretreatment and EA
treatment on I/R injury in SD rats. All rats were randomly divided into four
groups: Sham, MCAO, EAM, and MEA groups. In the MEA group, rats were treated with
EA at 1 min after reperfusion and 2.5 h before execution. In the EAM group, rats
were pretreated with EA for six consecutive days, and MCAO surgery was performed
2 h after the sixth EA pretreatment. Cerebral infarction, BBB integrity, and
neurological function of these four groups were evaluated at 24 h after
reperfusion. The extent of I/R-induced cerebral injury was quantified by TTC
staining. A marked escalation in infarct size was observed in the MCAO group
relative to the Sham group, whereas both the MEA and EAM groups exhibited
significantly diminished infarct volumes compared to the MCAO group (p
This study assessed the impact of EA on the DEG profile in the ischemic
ipsilateral brain of stroke-induced rats. In the MCAO group, relative to the Sham
group, 4798 DEGs were discerned, with 67.84% exhibiting an expression fold
change (FC) of at least 1.5 (1.5 FC,
MCAO vs. Sham | MEA vs. MCAO | EAM vs. MCAO | |||||||
Up | Down | Total | Up | Down | Total | Up | Down | Total | |
Total DEGs | 2514 | 2284 | 4798 | 836 | 949 | 1785 | 1956 | 2064 | 4020 |
1.5 FC DEGs | 1862 (74.07%) | 1393 (60.99%) | 3255 (67.84%) | 419 (50.12%) | 574 (60.48%) | 993 (55.63%) | 993 (50.77%) | 1375 (66.62%) | 2368 (58.91%) |
2.0 FC DEGs | 1160 (46.14%) | 479 (20.79%) | 1639 (34.16%) | 37 (4.43%) | 154 (16.23%) | 191 (10.70%) | 97 (4.96%) | 401 (19.43%) | 498 (12.39%) |
The number of upregulated (Up) and downregulated (Down) differentially expressed
genes (DEGs) of the middle cerebral artery occlusion (MCAO), MCAO plus EA-treated
(MEA), and EA-preconditioned plus MCAO (EAM) groups; total DEG number; and the
percentage of corresponding total DEGs are shown. Total DEGs: total DEGs with the
p values adjusted using the Bonferroni procedure (padj)
Upon considering all DEGs across the three groups, 1395 genes were found to be co-regulated by the MEA and MCAO groups, with 771 MCAO-upregulated genes downregulated by MEA, and 616 MCAO-downregulated genes upregulated by MEA. These MEA reverse-regulated genes accounted for 28.91% of the total DEGs in the MCAO group, and accounted for 77.7% of the total DEGs in the MEA group. In the context of the EAM and MCAO groups, 1550 coregulated DEGs were identified, with 428 genes upregulated post-MCAO surgery but downregulated by EA, and 470 genes downregulated post-MCAO surgery but upregulated by EA. Additionally, 299 co-downregulated genes and 353 co-upregulated genes were observed in these two groups. EAM reverse-regulated genes accounted for 18.72% of the total DEGs of the MCAO group, and accounted for 22.34% of the total DEGs in the EAM group (Table 2). These results suggest that post-stroke, EA mainly specifically attenuates aberrant gene expression induced by I/R injury, whereas pre-stroke EA preconditioning not only exerts specific regulatory effects on the abnormal genes induced by I/R injury but also modulates other genes, potentially contributing to the neuroprotective effects of acupuncture preconditioning on ischemic stroke.
DEGs | MEA Up | MEA Down | EAM Up | EAM Down | |
MCAO Up | 7 | 771 | 353 | 428 | |
MCAO Down | 616 | 1 | 470 | 299 | |
1.5 FC DEGs | MEA Up | MEA Down | EAM Up | EAM Down | |
MCAO Up | 6 | 468 | 116 | 185 | |
MCAO Down | 277 | 0 | 141 | 93 | |
2.0 FC DEGs | MEA Up | MEA Down | EAM Up | EAM Down | |
MCAO Up | 1 | 139 | 11 | 50 | |
MCAO Down | 24 | 0 | 6 | 5 |
The numbers of co-regulated differentially expressed genes (DEGs) in MCAO plus EA-treated (MEA) or EA-preconditioned plus MCAO (EAM) groups and the middle cerebral artery occlusion (MCAO) group at different expression levels are shown.
Upon examination of the distribution of DEG expression magnitudes, we found that approximately 60% of the total DEGs in each group exhibited an expression FC exceeding 1.5. This provides a comprehensive reflection of transcriptome changes of the brain tissue following I/R injury under different conditions. To explore functional changes at the cellular levels, we performed Gene Ontology (GO) annotation enrichment and functional analyses of these DEGs, employing the Metascape bioinformatics platform. Furthermore, we conducted cluster comparative analysis of the three subgroups based on different gene expression levels to gain a better understanding of the relatively significant and unique characteristics of each intervention.
GO enrichment analysis outcomes revealed that the upregulated DEGs in the MCAO
group were significantly enriched in biological processes related to both
internal and external stimuli, with the top 20 processes encompassing a response
to wounding, response to lipopolysaccharide, oxygen levels, and cellular response
to growth factor stimulus. Response to these stimuli also manifested in immune
and inflammatory reactions, encompassing cytokine production, innate immune
response, regulation of cell adhesion, leukocyte migration, and myeloid leukocyte
activation. Additionally, processes related to cell death, such as positive
regulation of cell death and negative regulation of cell population proliferation
were discerned (Fig. 2A). Among the top 20 Kyoto Encyclopedia of Genes and
Genomes (KEGG) signaling pathways, the pathways related to these functions
included the tumor necrosis factor (TNF) signaling pathway, cytokine–cytokine
receptor interaction, PI3K/AKT signaling pathway, nuclear factor kappa B (NF-
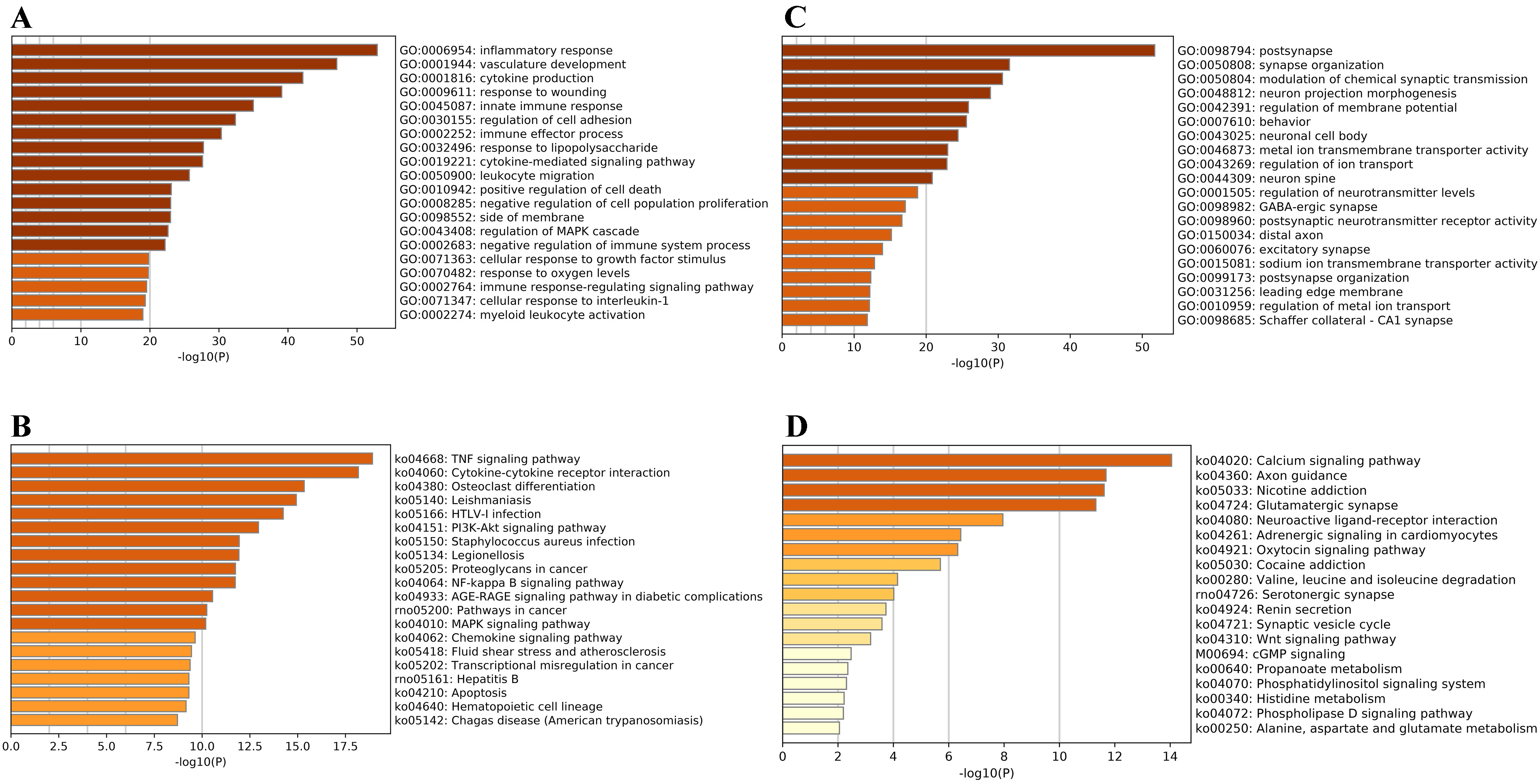
Bioinformatics Analyses of Differentially Expressed Genes in Sprague Dawley Rat Brains After Ischemic Stroke. (A) The top 20 Gene Ontology (GO) categories enriched by the
upregulated differentially expressed genes (DEGs) with
In the MEA group, the upregulated DEGs were primarily implicated in ion and
neurotransmitter transport, regulated by G protein-coupled receptor (GPCR) signal
transduction. These processes encompassed regulation of ion transport, potassium
ion transmembrane transporter activity, regulation of neurotransmitter levels,
active ion transmembrane transporter activity, and presynaptic membrane.
Additionally, a variety of metabolic processes were noted such as positive
regulation of organic acid transport, catecholamine metabolic process, regulation
of catecholamine secretion, fatty acid binding, and positive regulation of
alkaline phosphatase activity (Fig. 3A). KEGG analyses identified eight signaling
pathways enriched by the upregulated DEGs including the cell cycle, neuroactive
ligand–receptor interaction, adrenergic signaling in cardiomyocytes, calcium
signaling pathway, and gap junction (Fig. 3A). On the other hand, the
downregulated DEGs in the MEA group were significantly enriched in biological
processes related to the response of the organism to physical and chemical
stimuli including cellular response to growth factor stimulus, inflammatory
response, wound healing, response to mechanical stimuli, response to
lipopolysaccharides, and cellular response to interleukin 1 (IL-1). The top 20
enriched KEGG signaling pathways were also closely associated with the
aforementioned functions such as the inflammatory responses mediated by the TNF,
PI3K/AKT, NF-
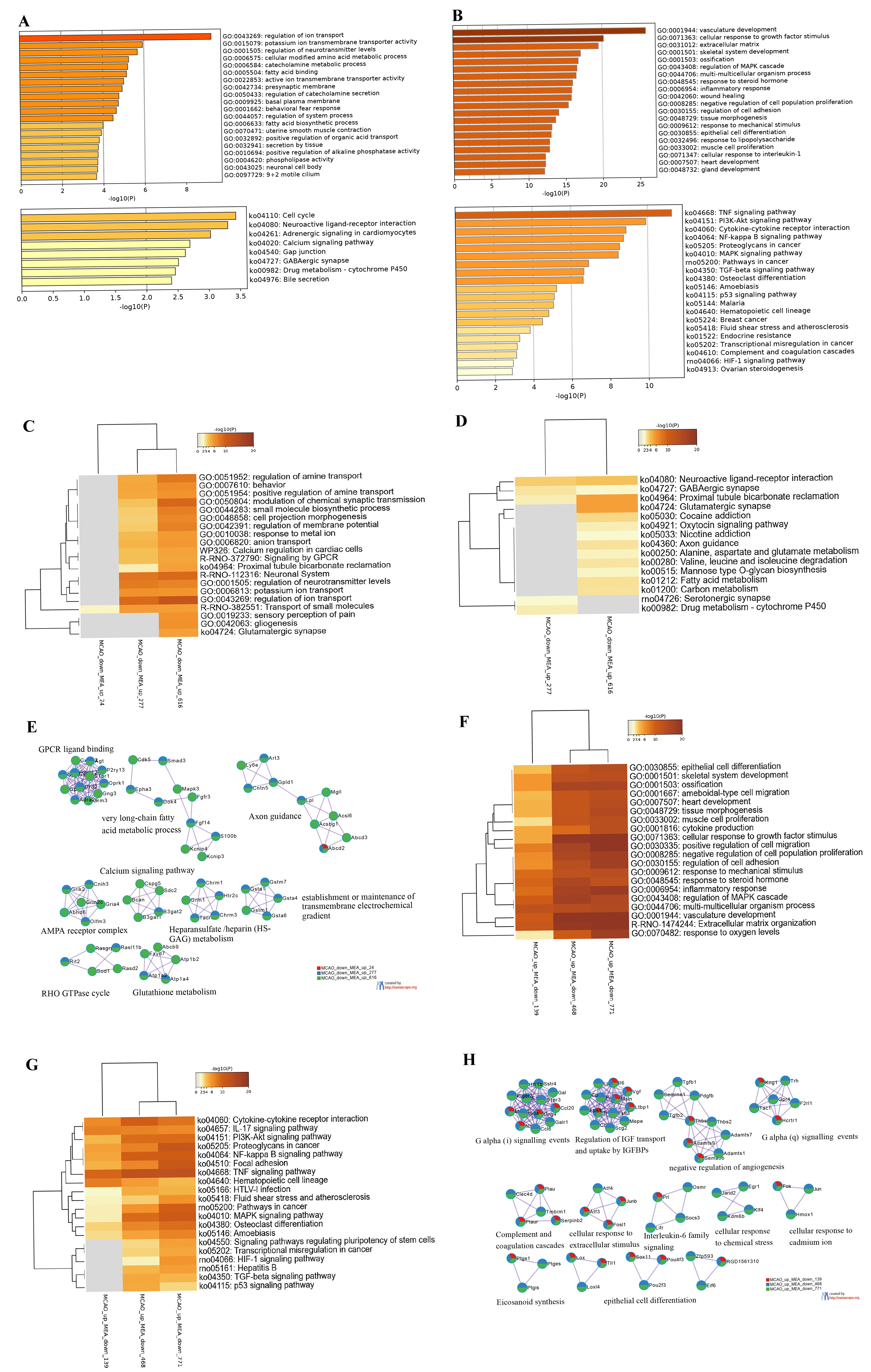
Bioinformatics Analyses of the Differentially Expressed Genes in
Rat Brains in the Middle Cerebral Artery Occlusion Plus
Electroacupuncture-Treated (MEA) Group. (A) The top 20 Gene Ontology (GO)
categories and Kyoto Encyclopedia of Genes and Genomes (KEGG) pathways enriched
in the middle cerebral artery occlusion (MCAO) plus electroacupuncture
(EA)-treated (MEA)-upregulated DEGs with
To gain a comprehensive understanding of the protective effects of EA treatment
on brain injury in stroke-induced rats, we further focused on the DEGs that were
induced by stroke but reversed by EA treatment. Among the 277 DEGs that were
downregulated by MCAO but upregulated by MEA with
In the EAM group, GO analyses of the upregulated DEGs indicated that the top 20 significant biological processes were primarily related to three main categories: energy metabolism, protein synthesis, and stress response. Examples of these processes include the mitochondrial protein-containing complex, cytoplasmic side of the rough endoplasmic reticulum membrane, and cellular response to toxic substances. In addition, KEGG analyses revealed that energy supply and substance metabolism accounted for the largest proportion, involving oxidative phosphorylation, proteasome, and glycolysis, among others. Furthermore, these DEGs were also found to participate in the synaptic vesicle cycle, which is associated with neural transmission signaling and RNA degradation (Fig. 4A). For the downregulated DEGs, the top 20 significant biological functions clusters were mainly related to the composition and function of synapses and neurons such as integral component of the synaptic membrane/presynaptic membrane, positive regulation of cell projection organization, forebrain development, and negative regulation of neurotransmitter secretion. Furthermore, these DEGs were involved in the regulation of gene expression such as mRNA metabolic processes, ATP-dependent chromatin remodeler activity, histone modification, and mRNA processing. The results of KEGG analyses revealed eight enriched signaling pathways including lysine degradation, axon guidance, and the extracellular matrix (ECM)–receptor interaction (Fig. 4B).
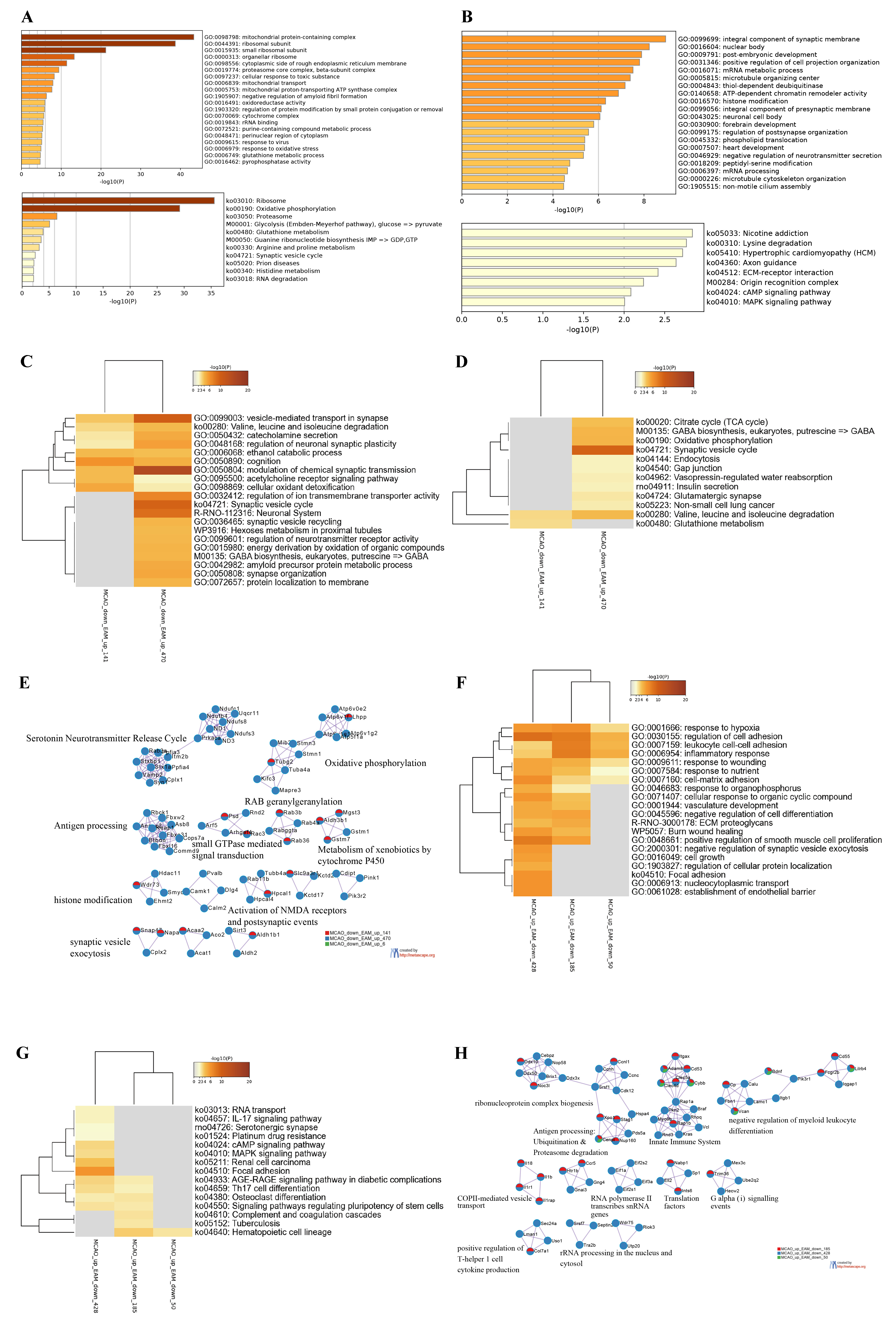
Bioinformatics Analyses of Differentially Expressed Genes in Stroke-Induced Rats with Electroacupuncture Preconditioning.
(A) The top 20 Gene Ontology (GO) categories and Kyoto Encyclopedia of Genes and
Genomes (KEGG) pathways enriched by EA-preconditioned plus middle cerebral artery
occlusion (MCAO) (EAM)-upregulated differentially expressed genes (DEGs) with
We subsequently conducted functional enrichment and protein interaction analyses on the DEGs that were co-regulated by MCAO and EAM. GO enrichment analyses revealed that the protein products, translated by these DEGs that were downregulated by MCAO but upregulated by EAM, particularly those with expression FC exceeding 1.5, were predominantly implicated in two clusters of biological processes. The first cluster pertained to regulating neurotransmitter transmission and the maintenance of neural excitability, encompassing modulation of chemical synaptic transmission, vesicle-mediated transport in synapse, cognition, regulation of neuronal synaptic plasticity, and catecholamine secretion (Fig. 4C). The corresponding enriched KEGG signaling pathways included the synaptic vesicle cycle, GABA biosynthesis, endocytosis, gap junction, and the glutamatergic synapse (Fig. 4D). The second cluster was associated with the brain’s energy supply and the regulation of substance metabolism including the ethanol catabolic process, energy derivation by oxidation of organic compounds, and cellular oxidant detoxification. The corresponding enriched KEGG signaling pathways included valine, leucine, and isoleucine degradation; glutathione metabolism; the tricarboxylic acid cycle; oxidative phosphorylation; and insulin secretion (Fig. 4C,D). PPI analyses confirmed that these DEGs were primarily involved in the biological function of neural excitability (e.g., serotonin neurotransmitter release cycle, activation of N-methyl-D-aspartate receptors, and synaptic vesicle exocytosis), energetic metabolism (e.g., metabolism of xenobiotics by cytochrome P450, oxidative phosphorylation, Rab geranylgeranylation), and protein modification (e.g., neddylation and histone modification) (Fig. 4E). The DEGs that were upregulated in the MCAO group but downregulated in the EAM group were largely associated with immune inflammatory responses and gene transcriptional activity including the response to hypoxia, regulation of cell adhesion, leukocyte cell-cell adhesion, and inflammatory responses (Fig. 4F). The corresponding enriched signaling pathways were the IL-17 signaling pathway, T helper 17 (Th17) cell differentiation, focal adhesion, and others (Fig. 4G). The functional clusters enriched by PPI analyses were in alignment with the GO and KEGG results including ribonucleoprotein complex biogenesis, antigen processing: ubiquitination and proteasome degradation, the innate immune system, negative regulation of myeloid leukocyte differentiation, and positive regulation of Th1 cell cytokine production (Fig. 4H). Additionally, these DEGs also regulated the advanced glycation end product (AGE)-receptor for AGE (RAGE) signaling pathway in diabetic complications, which was associated with vascular injury (Fig. 4F,G).
Excluding the EAM-reversed co-regulated DEGs, we observed that approximately 40% (652/1550) of the co-regulated DEGs in the MCAO and EAM groups exhibited the same expression pattern. To thoroughly decipher the potential mechanisms through which acupuncture pretreatment mitigates brain injury in stroke rats, we subjected these genes to biological analyses. The co-upregulated DEGs in both the MCAO and EAM groups were primarily linked to the augmentation of immune capacity (e.g., immunoglobulin-mediated immune response, innate immune response, regulation of the defense response), the promotion of non-neurotic cell death (e.g., apoptotic signaling pathway, ferroptosis, positive regulation of programmed cell death), and the regulation of ribosomal protein synthesis (Fig. 5A,C). PPI analyses demonstrated that these co-upregulated DEGs were predominantly enriched in functions such as the ribosome, p53-dependent G1 DNA damage response, neutrophil degranulation, defense response to bacterium, and cellular response to oxidative stress (Fig. 5E). Conversely, the co-downregulated DEGs in these two groups were chiefly tasked with regulating neuronal excitability including modulation of the neuronal membrane potential, glutamate receptor complex, and potassium ion transmembrane. Additionally, these DEGs were also implicated in the regulation of myocardial contractile function (Fig. 5B,D). PPI analyses indicated that these downregulated DEGs were primarily involved in neuron recognition, chloride transmembrane transport, neuronal action potential, and protein ubiquitination (Fig. 5F). In summary, the co-regulated DEGs in the MCAO and EAM groups appeared to bolster the organism’s defense ability and maintain neural excitability and function.
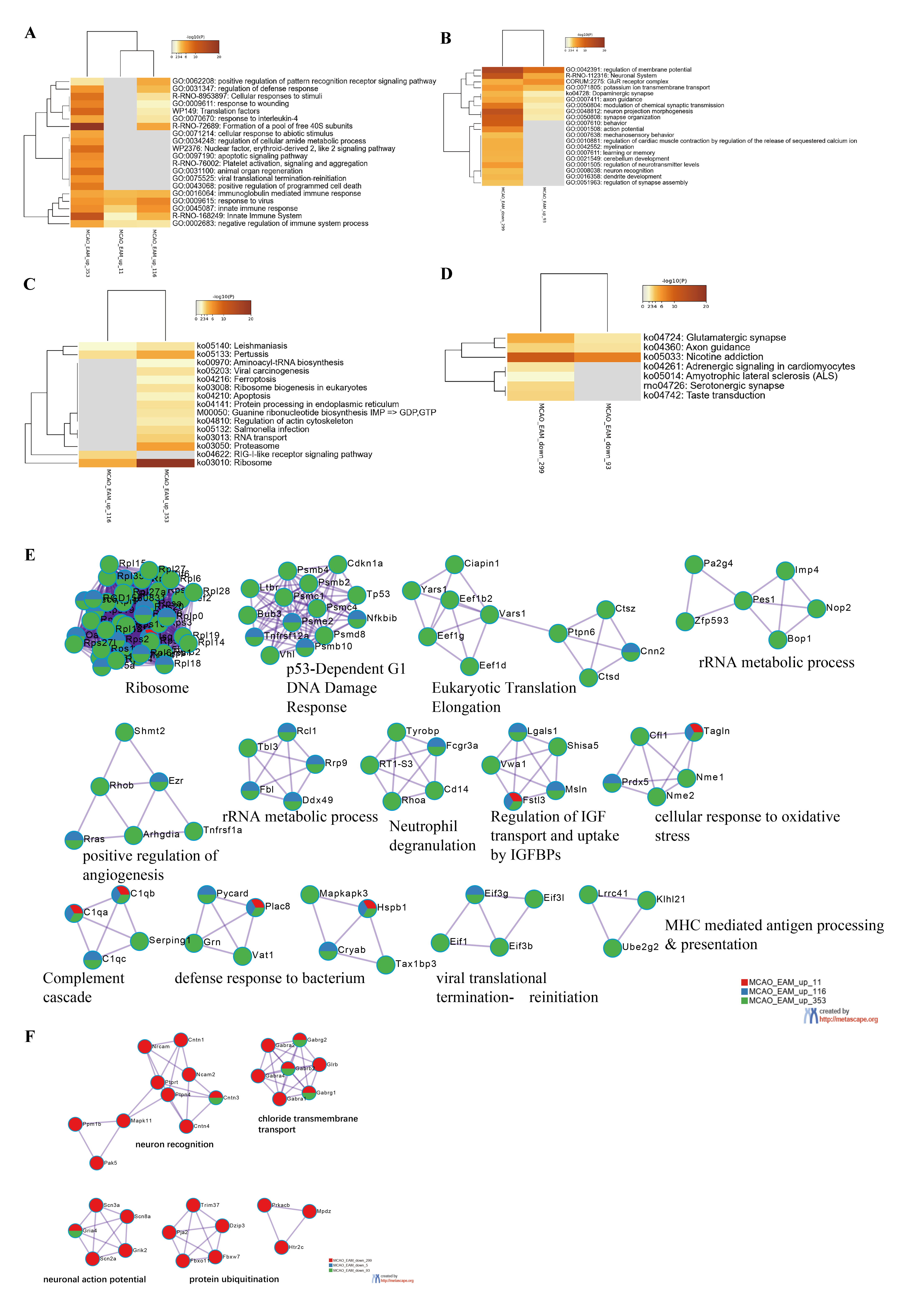
Bioinformatics Analyses of the Co-upregulated or Co-downregulated Differentially Expressed Genes in the Electroacupuncture-Preconditioned Plus Middle Cerebral Artery Occlusion (MCAO) (EAM) and MCAO groups. (A,B) The top 20 functional clusters enriched by co-upregulated or co-downregulated differentially expressed genes (DEGs) between the middle cerebral artery occlusion (MCAO) and electroacupuncture-preconditioned plus MCAO (EAM) groups. (C,D) The Kyoto Encyclopedia of Genes and Genomes pathways enriched by co-upregulated or co-downregulated regulated DEGs between the MCAO and EAM groups. (E,F) Protein-protein interaction network analyses of the co-upregulated or co-downregulated DEGs between the MCAO and EAM groups.
Based on the analyses, we determined that the biological processes implicated in the DEGs coregulated by either the MEA or EAM group and the MCAO group individually bore resemblance to each other, such as oxidative stress, immunoinflammatory responses, cell death, excitatory neurotoxic responses, and neurological dysfunction, which are the primary pathological processes of stroke. This observation prompted us to determine whether the mechanisms of EA preconditioning and treatment were identical. To this end, we compared the DEGs between the MEA and EAM groups and discovered that there were 532 identical genes in these two groups, constituting only 29.8% and 13.23% of the total DEGs in the MEA and EAM groups, respectively. Among these identical DEGs, 185 genes were upregulated and 201 genes were downregulated in both groups. Interestingly, 80 genes were upregulated in the EAM group but downregulated in the MEA group, while 66 genes exhibited the opposite pattern (Fig. 6A). To delve deeper into the shared mechanisms of EA preconditioning and treatment, we compared the co-regulatory genes of the MEA and EAM groups with the DEGs in the MCAO group. We found that a significant majority (81.08% or 150/185) of the co-upregulated genes in these two groups were the downregulated DEGs in the MCAO group. These genes were primarily involved in substance and energy metabolism and glial cell activation such as the citric acid cycle and respiratory electron transport, cellular biogenic metabolic process, and mitochondrial ATP synthesis-coupled proton transport (Fig. 6B). On the other hand, of the 201 co-downregulated genes in the MEA and EAM groups, 159 were upregulated DEGs in the MCAO group. These genes were primarily involved in the stress and immune response to hypoxic environments such as the response to hypoxia, ECM–receptor interaction, regulation of cell adhesion, and cellular response to organic cyclic compounds (Fig. 6C).
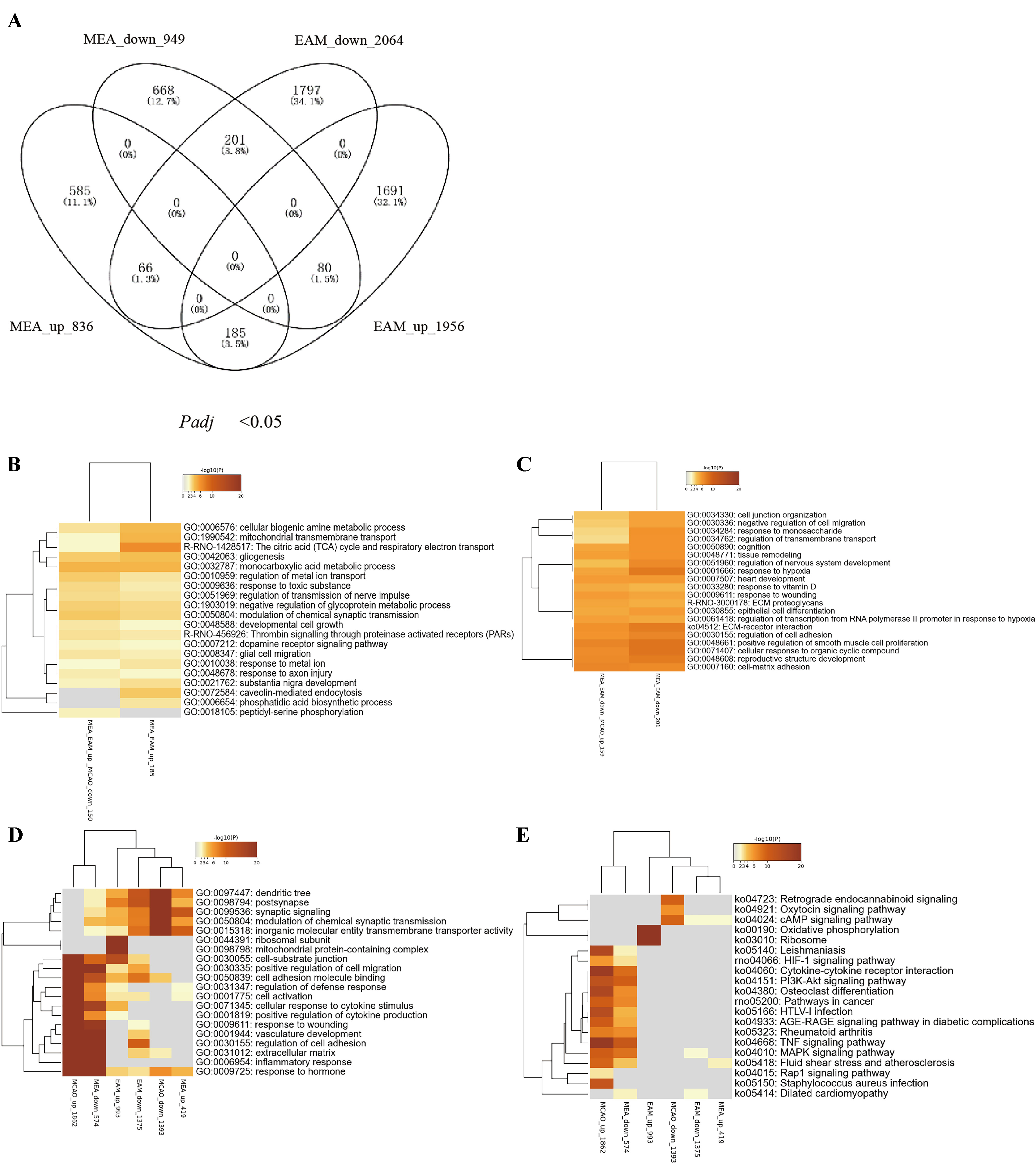
Bioinformatics Analyses of the Differentially Expressed Genes in the Middle Cerebral Artery Occlusion (MCAO) Plus Electroacupuncture (EA)-Treated (MEA) Group Versus EA-Preconditioned Plus MCAO (EAM) group. (A) Venn diagrams show the numbers of differentially expressed genes (DEGs) overlapping in the middle cerebral artery occlusion (MCAO) plus electroacupuncture (EA)-treated (MEA)-upregulated, MEA-downregulated, EA-preconditioned plus MCAO (EAM)-upregulated, and EAM-downregulated DEGs at different expression levels. (B,C) The top 20 functional clusters enriched by co-upregulated or co-downregulated DEGs between the MEA and EAM groups. (D,E) Comparative enrichment analyses of Gene Ontology and Kyoto Encyclopedia of Genes and Genomes pathways enriched by the upregulated and downregulated DEGs in the MCAO, MEA, and EAM groups.
Subsequently, we conducted comprehensive analyses of all upregulated and downregulated DEGs in the MEA, EAM, and MCAO groups using GO and KEGG pathways, with the aim of identifying the key characteristics and pathways of EA treatment and preconditioning in the regulation of ischemic stroke pathophysiological processes. Our findings revealed clear directionality in the biological processes and signaling pathways enriched by I/R-induced DEGs. Specifically, These I/R-induced upregulated DEGs were primarily involved in the activation, migration, and differentiation of immune inflammatory cells; and the downregulated DEGs were highly enriched in neural functions that primarily regulate synaptic excitability (Fig. 6D,E). The biological functions involved in the DEGs of the MEA group were mainly specifically targeted at the abnormal pathological processes caused by the MCAO group. Interestingly, for the EAM group, although most of the GO clusters enriched by the DEGs overlapped with those in the MCAO and MEA groups, the three top significant GO and KEGG clusters were related to mitochondrial energy metabolism (Fig. 6D,E), which were not the main regulatory targets of DEGs in the MCAO and MEA groups.
Acupuncture, a key component of traditional Chinese medicine, has been shown in our work and others to effectively alleviate cerebral infarction, BBB damage, cell death, and neuroinflammatory responses in the brain after a stroke [5, 6, 7, 8, 10, 11, 12, 13, 14, 15, 16, 18]. However, these studies have solely focused on the effects and mechanisms of acupuncture interventions either before or after a stroke. As a result, it is unclear whether there are any differences in the therapeutic effects and mechanisms of acupuncture interventions administered at these two distinct time points on post-stroke pathological damage. Therefore, the current study compared the effects of pre-stroke acupuncture with those of post-stroke acupuncture treatment on post-stroke pathological damage. The results indicated that both interventions significantly reduced infarct volume, EB permeability, and neurological injury scores in stroke-induced rats, and the neuroprotective effects of acupuncture on I/R injury were comparable at these two different time points.
To gain a better understanding of the mechanisms involved in acupuncture intervention before and after a stroke, we employed RNA-Seq technology to obtain gene transcriptome profiles of rat brain tissue from three groups: the MCAO, MEA, and EAM groups. The expression levels in each group revealed that the proportion of DEGs with an expression FC more than 1.5 in the ischemic brain tissue of rats in the three groups was similar. However, when comparing the DEGs with an expression FC greater than 2.0, it was found that both the absolute number and proportion of DEGs of the MCAO group (1639, 34.16%) were significantly higher than those in the EAM (191, 10.70%) and MEA groups (498, 12.39%). This suggests that EA stimulation is milder than I/R injury in rats. Although EA can cause changes in gene expression, the intensity of regulation of DEG expression is less than that of I/R injury. Additionally, the MCAO group demonstrated a significantly higher abundance of upregulated DEGs compared to downregulated ones. By contrast, in the MEA and EAM groups, downregulated DEGs outnumbered the upregulated ones, a finding that aligns with previous research [19, 21].
In relation to the counteractive effects of acupuncture intervention on stroke-induced DEGs, it was observed that pre- and post-stroke acupuncture intervention could inversely regulate 20% and 30% of stroke-induced DEG expression, respectively, consistent with our previous findings [19]. Interestingly, despite the lower total number of DEGs in the MEA group compared to the EAM group, the specificity of acupuncture treatment in modulating the aberrantly expressed genes induced by MCAO was notably superior in the former. Specifically, in the MEA group, approximately 80% of DEGs were abnormally expressed genes triggered by I/R injury. By contrast, in the EAM group, less than 40% of DEGs were genes co-regulated by MCAO, with more than 60% being non-MCAO co-regulated genes. The quantity of upregulated and downregulated genes in MCAO that were reversed by EA treatment exceeded that of EA pretreatment. Moreover, among the DEGs reversed by EA, the proportion of genes with an expression FC greater than 2 in the MEA group was more than double that in the EAM group.
Our bioinformatics analysis revealed that DEGs in the MCAO rats predominantly
pertained to cell death, stress response, immune inflammatory response, and
synaptic functions triggered by post-ischemic hypoxia. The top three GO and KEGG
signaling pathways enriched by upregulated DEGs due to I/R injury were
inflammatory responses, vasculature development, and cytokine production; and the
TNF signaling pathway, cytokine–cytokine receptor interaction, and osteoclast
differentiation, respectively. Conversely, the top three GO and KEGG signaling
pathways linked to the downregulated DEGs were post-synapse, synapse
organization, and modulation of chemical synaptic transmission; and calcium
signaling pathway, axon guidance, and glutamatergic synapse, respectively.
Interestingly, a comparison of the KEGG signaling pathways between the MCAO and
MEA groups showed that 11 of the top 20 KEGG signaling pathways enriched by
downregulated DEGs in the MEA group were identical to those enriched by the
upregulated DEGs in the MCAO group, including the top three significantly
enriched signaling pathways of the MCAO group. The key pathophysiological
processes identified were primarily associated with excessive inflammatory
response, stress response, and cell death, which contributed to brain tissue
damage and loss of neurological function post-stroke. Specifically,
neuroinflammation, angiogenesis, and neuroplasticity are the hotspots in ischemic
stroke and acupuncture. For example, Xian et al. [22] reported that
acupuncture treatment mitigated the level of inflammatory factors
(TNF-
Although only three signaling pathways, namely, the calcium signaling pathway, neuroactive ligand–receptor interaction, and adrenergic signaling in cardiomyocytes, were enriched by the upregulated DEGs in the MEA group, these pathways were all downregulated in the MCAO group. Interestingly, the enriched signaling pathways of upregulated genes in the MEA group and downregulated genes in the MCAO group shared nine KEGG pathways, four of which were also among the top five enriched KEGG signaling pathways of downregulated DEGs in the MCAO group. These pathways play a role in regulating neurological excitability and neurotransmitter metabolism, and some have previously been shown to be influenced by acupuncture. For instance, it has been found that acupuncture can induce calcium influx into cells, which triggers mast cell degranulation and the release of cellular mediators, thereby activating surrounding nerve cells and exciting the local neural network [26]. Additionally, EA can inhibit GABAergic neurons and activate glutamatergic neurons in the ventrolateral periaqueductal gray through cannabinoid receptor type 1 receptors, resulting in EA-induced analgesia [27]. Pak et al. [28] showed that EA may exert protective effects on dopaminergic neurons by upregulating the expression of brain-derived neurotrophic factor and glial cell line-derived neurotrophic factor in both the substantia nigra and striatum. Overall, these findings suggest that post-stroke acupuncture treatment exerts strong, specific reverse regulatory effects on abnormally expressed genes induced by I/R injury.
We carried out comprehensive bioinformatics analyses of the DEGs in the EAM group. The functional clusters enriched by the DEGs in the EAM group presented a distinct pattern compared to those in the MEA group. The majority of the top 20 GO clusters enriched by the downregulated DEGs in EAM group were not implicated in the inflammatory stress response. Instead, they were predominantly involved in the regulation of neurogenesis, gene transcriptional activity, and cardiac function. The top three signaling pathways enriched by the downregulated DEGs in the EAM group were nicotine addiction, lysine degradation, and hypertrophic cardiomyopathy signaling pathways. By contrast, the major clusters of biological functions enriched by the upregulated DEGs in the EAM group were not primarily related to neurofunctional regulation. Instead, they were more associated with substance metabolism and energy production. However, bioinformatics analyses of DEGS that were inversely regulated by acupuncture preconditioning showed that the functional clusters enriched by DEGs upregulated by MCAO but downregulated by EAM were related to immune inflammatory responses, cell adhesion, and vascular damage-related signaling pathways. In terms of the KEGG pathways enriched by these co-regulated DEGs, osteoclast differentiation, the AGE-RAGE signaling pathway in diabetic complications, the MAPK signaling pathway, and the hematopoietic cell lineage were among the top 20 pathways in the MCAO group. On the other hand, the functional clusters enriched by DEGs downregulated by MCAO but upregulated by EAM were not only involved in substance and energy metabolism but also in the generation of neural action potentials mediated by synaptic transmission and metabolism (Fig. 4C). The top 20 signaling pathways enriched by these co-regulated DEGs included the glutamatergic synapse; synaptic vesicle cycle; and valine, leucine, and isoleucine degradation. Recent studies have highlighted the critical role of mitochondrial damage in stroke-induced injury, and the importance of glycogenolysis in astrocytic glycogen accumulation and brain damage after reperfusion in ischemic stroke [29, 30]. The functional profile of immune cells is heavily influenced by their metabolic state, which is disrupted during I/R injury and can lead to an increase in glycolysis that supports proinflammatory cells and inhibits the anti-inflammatory function of regulatory T cells [31, 32, 33]. This suggests that substance metabolism and energy production may be potential targets for acupuncture pretreatment of stroke. While acupuncture preconditioning has reverse regulatory effects on major pathophysiological processes after stroke, it may not be the most important factor in protecting against I/R injury. Instead, the primary mechanisms by which acupuncture preconditioning reduces neuroinflammatory response and alleviates neurological hypofunction after ischemia involve the upregulation of brain energy synthesis capacity, downregulation of gene transcriptional activity in the brain, and regulation of cardiac contractility to improve blood flow supply.
To delve deeper into the neuroprotective effects of acupuncture in preventing and treating ischemic stroke, we conducted comparative enrichment analyses of DEGs that were upregulated and downregulated across three distinct groups. Our findings underscore that the most detrimental pathological impacts on rat brain tissue following a stroke are an overactive immune inflammatory response and diminished function of nerve impulse conduction. We discovered that timely intervention with post-stroke EA could specifically downregulate the expression of genes linked to immuno-inflammation in stroke-induced rats. This intervention also increased the expression of genes associated with nerve impulse transmission in the brain, thereby mitigating the brain damage instigated by ischemia and hypoxia. In addition, we found that acupuncture preconditioning prior to a stroke could not only attenuate the post-stroke inflammatory response to some extent but also significantly enhanced the metabolism and energy supply of substances in the brain following an ischemic stroke. This preconditioning also had the effect of downregulating gene transcriptional activity, which in turn reduced the excessive stress response of brain tissue to both internal and external stimuli.
This study demonstrates that administering acupuncture both before and after a stroke can significantly reduce the damage to the brain tissue caused by an ischemic stroke in rats. This is the first study to compare the changes in gene expression in the brain of rats with ischemic stroke that received acupuncture preconditioning compared to those that received acupuncture treatment. The results showed that while there are some similarities in the regulation of abnormal gene expression between the two interventions, each intervention has unique targets. Therefore, a combination of acupuncture preconditioning and acupuncture treatment may offer a more comprehensive and effective form of neuroprotection for stroke patients.
BBB, blood-brain barrier; EA, electroacupuncture; I/R, ischemia-reperfusion; MCAO, middle artery occlusion; DEGs, differentially expressed genes; TTC, 2,3,5-triphenyl tetrazolium chloride; GO, Gene Ontology; KEGG, Kyoto Encyclopedia of Genes and Genomes; PPI, protein-protein interaction.
The datasets used or analyzed during the current study are available from the corresponding author on reasonable request.
SF and SL initiated and designed the experiments. BW, RP, LOY, MZ, TX, CC performed the experiments. HX and XJ analyzed the data. BW and SF wrote and edited the manuscript. All authors contributed to editorial changes in the manuscript and approved the final manuscript.
Experiments were performed under the NIH Guide for the Care and Use of Laboratory Animals. All surgical procedures as well as animal executions were performed under isoflurane anesthesia, and every effort was made to minimize animal suffering. Laboratory animal license number: SCXK(Shanghai) 2013-0016. The animal study was reviewed and approved by the Institutional Animal Care Unit Committee of the Nanjing University of Chinese Medicine (No.202103A003).
Not applicable.
This research was funded by the National Natural Science Foundation of China (No. 81774403), the Key University Science Research Project of Jiangsu Province (22KJA360003), the School of Elderly Care Services and Management (Nanjing University of Chinese Medicine) (2023YLFWYGL016).
The authors declare no conflict of interest.
Publisher’s Note: IMR Press stays neutral with regard to jurisdictional claims in published maps and institutional affiliations.