- Academic Editor
†These authors contributed equally.
Food is an integral part of our civilization. It is a cultural phenomenon that, while having evolved, is associated with societal traditions and identity. This work analyzes studies conducted to highlight the health properties of the most common ethnic foods. Although these foods were originally created from the need to preserve perishable produce, presently, we know that the fermentation process makes them nutritionally more complete. The basis of these transformations lies in that vast range of prokaryotic and eukaryotic microorganisms that, similar to small biochemical factories, can transform the initial nutrients into metabolically more active biomolecules through fermentation. Although naturally occurring microbes work together for mutual benefit, environmental conditions enhance or inhibit their development. Starting from a selection of microorganisms naturally present on a substrate, we attempt to select the most suitable species to obtain a fermented food with the best nutritional qualities and the richest in nutraceuticals.
Since prehistory, fermentation has been used to meet food storage needs. Numerous discoveries have allowed the reconstruction of the first forms of fermentation in different continents—in Egypt, bread, beer, and dairy products were part of the traditional diet [1]; in Europe and the Middle East, there is evidence of fermented kinds of milk being produced [2]; in Asia: there was the production of fermented foods based on vegetables and rice [3].
Today, fermentation continues to be practiced worldwide to increase the shelf-life of raw produce and obtain foods with more pleasant organoleptic characteristics and superior nutritional properties. Indeed, standardized fermentation processes have been developed to improve the traditional fermentation method, which exploits the microbial flora naturally present on the substrate and whose presence is influenced by environmental conditions. These are necessary to obtain hygienically safer products with organoleptic characteristics that are repeatable over time. Thus, to achieve these results, the use of selected starter cultures has become indispensable [4].
Recently, the identification of microbial species best suited to different industrial biotechnological processes has been based on studying the genomes of the most useful microorganisms [5]. From a biochemical point of view, fermentation is an anaerobic metabolic process capable of allowing microorganisms to produce energy from nutrients without oxygen. In the context of industrial food microbiology, fermentation refers to both the anaerobic and aerobic processes that are performed by microorganisms, which meet the chemical ideal for transforming primary nutrients into industrially interesting secondary substances. Moreover, based on these biochemical transformations, we can identify four main types of fermentation: acid, alkaline, alcoholic, and lactic [6, 7].
During acetic fermentation, chemically referred to as oxidation, bacteria belonging to the genus Acetobacter, which are Gram-negative, non-sporigenic, and aerobi, convert alcohol into acetic acid by producing foods such as vinegar and kombucha [8]. During alkaline fermentation, mixed bacterial cultures, mainly dominated by Bacillus subtilis, a Gram-positive, rod-shaped bacterium, and obligated aerobic [7], are responsible for transforming nitrogen compounds present in the starting substrates through the release of ammonia and consequent increase in pH. Thus, the obtained fermented foods include Japanese natto [7]. Further, by exploiting the fermentative capacities of yeasts, mainly of the genus Saccharomyces, alcoholic fermentation produces beer, wine, and bread.
During lactic fermentation, sugars are fermented into lactic acid by homofermenting lactic acid bacteria or into lactic acid, acetic acid, ethanol, and carbon dioxide by heterofermenting lactic acid bacteria (LAB), which are Gram-positive microorganisms. Moreover, the optional anaerobic and rod cells involved in these fermentation processes allow the production of many foods, such as yogurt, kefir, sauerkraut, and kimchi: foods with recognized health values [9].
Nonalcoholic cereal-based beverages that have a positive impact on the health of all consumers and are suitable for people with lactose intolerance, vegans, and vegetarians can be prepared [10].
In the fermentation processes just described, it is frequently not a single microbial species that is responsible for the final product but a collaboration between different organisms and strains. This synergy is obvious in the case of food and beverages obtained spontaneously since the agents of such biochemical transformations will be the microorganisms naturally present on the food substrate. Additionally, in industrial processes, which use starter cultures, the combination of microbial agents ensures a process that is more easily controllable, and produces known and reproducible results over time.
This microbial collaboration has been found in the production of sauerkraut, kimchi, kefir, and yogurt, and even, on a broader scale, in the production of kombucha, which takes place because of the symbiosis of bacteria and yeast (SCOBY). SCOBY is the acronym for Symbiotic Culture of Bacteria and Yeast—cultures of bacteria and yeasts that live symbiotically. The SCOBY usually appears as a light brown gelatin disc, a few centimeters thick and a little slimy and shiny, with an intense acetic odor. When healthy, it appears compact, meaning dividing it into smaller pieces by hand can sometimes be difficult.
Thus, the fermented foods obtained are classified as functional foods due to their recognized nutritional properties and their health-related interaction with the human microbiome.
In 1996, under the coordination of the ILSI (International Life Science Institute), an action was initiated by the European Commission on Functional Food Science in Europe (FU.FO.S.E) with the aim of establishing and developing an evidence-based scientific approach to support the development of food products that can be defined as functional foods. The final document was published in 1999 [11]. According to the FU.FO.S.E., functional food is defined as food that can both reduce the risk of disease and improve health and well-being. Functional foods should be able to perform their function in the quantities found within a balanced diet [12].
Sauerkraut is one of the best-known fermented vegetable products (Fig. 1). It is believed that 2000 years ago, cabbage fermentation was already customary in China, while this technique arrived in Europe about 1000 years ago. Fermented cabbage products are currently consumed in Europe, North America, and Asia [13].
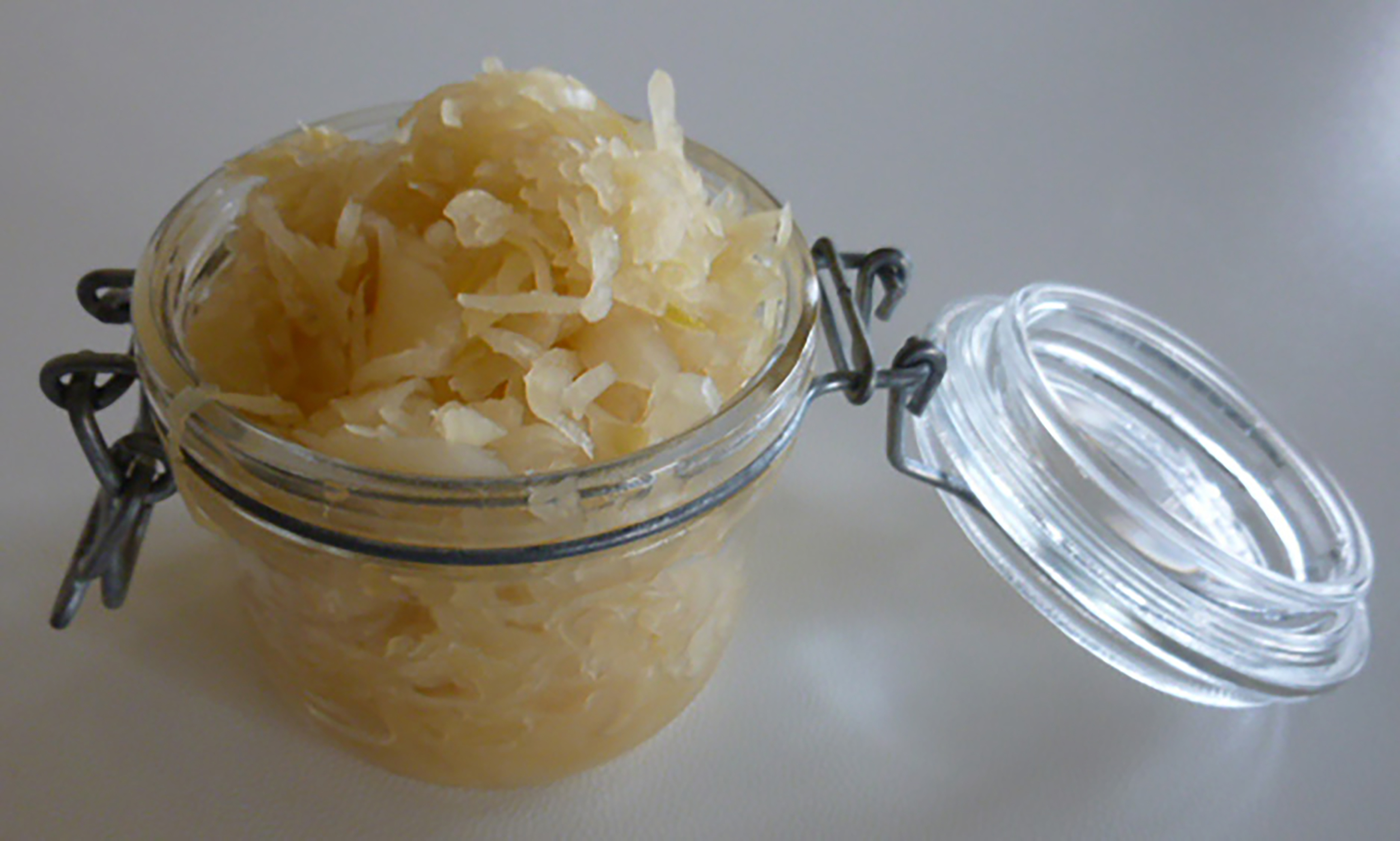
Sauerkraut. Sauerkraut is obtained by the fermentation of cabbage (Brassica oleracea L.).
The cabbage (species: Brassica oleracea L.- Var. capitata L.) belongs to the Brassicaceae family [14]; it is one of the most widely cultivated crops in the world, owing to its adaptability to different climates and soils.
The members of this family are rich in antioxidant molecules, such as vitamin C, polyphenols, thiols, and carotenoids, in addition to glucosinolates (GLS), which are recognized as being valuable against degenerative diseases and cancer [15, 16]. Hence, owing to these characteristics, they are classifiable as functional foods.
In the production of sauerkraut, the cabbage is cleaned, then cut into thin strips and salted. The addition of sodium chloride salt facilitates the release of vegetation water through osmosis, which then forms a brine [17]. Salt plays a fundamental role in the preparation of sauerkraut, as it limits the development of Gram-negative microorganisms, naturally present in the food, and promotes the growth of lactic acid bacteria [18].
The production of sauerkraut is carried out by the synergistic fermentative action of numerous indigenous microbial species present on the surface of the aerial parts of the plant.
Today, the use of a microbial starter culture is preferred on an industrial level to obtain a finished product with constant organoleptic characteristics.
A recent study [19] showed the order of action of epiphytic microorganisms. The fermentation process begins with the heterofermenting lactic bacteria Leuconostoc mesenteroides and Leuconostoc citreum, which initiate the fermentation of glucose and fructose through the production of lactic acid, acetic acid, mannitol, and carbon dioxide; these bacteria poorly tolerate the subsequent acidification of the substrate, and their presence decreases after a week. They are replaced by L. casei, P. parvulus, and L. plantarum, which initiate homolactic fermentation, resulting in a sharp increase in lactic acid. Fermentation, which takes place over 35 days, will be completed by L. plantarum.
Nutritionally, sauerkraut is considered a functional food due to its actions: antioxidant action caused by the presence of vitamin E, vitamin C, and phenolic compounds [17, 19, 20, 21]; anti-cancerogenic action due to ascorbigen and isothiocyanates [21, 22, 23]; anti-inflammatory action due to allyl isothiocyanate and indol-3-carbinol [20, 24, 25, 26].
It should also be noted that sauerkraut, similar to other fermented foods, is a source of LAB. These probiotics increase innate and adaptive immunity, allowing a reduction in inflammatory states with the re-modulation of the intestinal microbiota [27, 28, 29].
Kimchi (Fig. 2) is a fermented food product that has typified Korean food culture for thousands of years. The primary vegetable is Chinese cabbage, while other ingredients include radish, onion, garlic, chili powder, and ginger, and it is used as a substrate for subsequent lactic fermentation [30]. More than 167 types of kimchi exist, which vary according to their ingredients, the geographical area of production, and their production method [31].
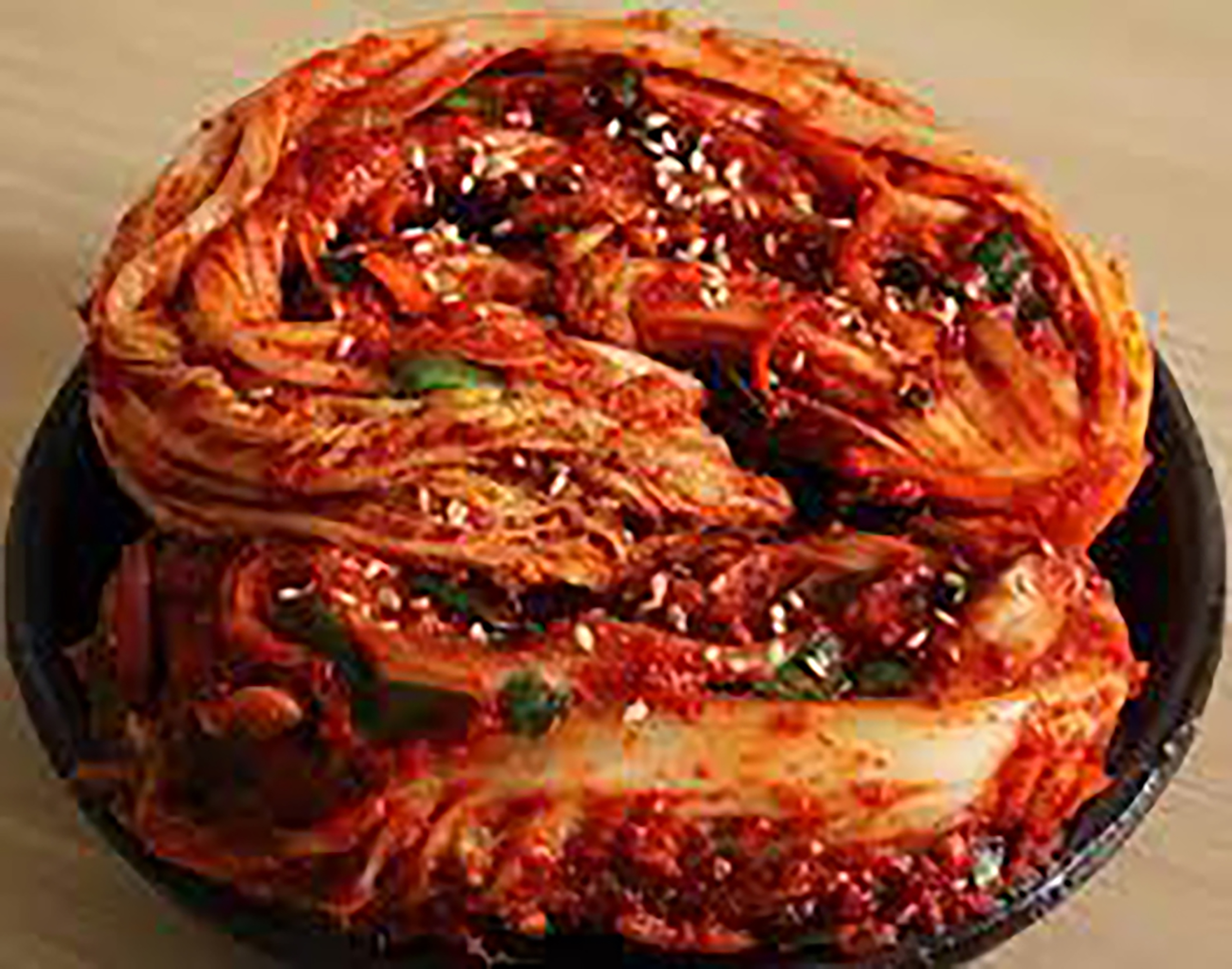
Kimchi. Kimchi, a typical Korean food.
For Korean people, it is considered a symbol of identity (it is estimated that the annual consumption per capita reached almost 40 kg in 2017) [32, 33, 34], so much so that in 2013, it was included by UNESCO on the Intangible Cultural Heritage list [33].
The nutritional properties of Kimchi derive from both the chemical composition of the raw material and the metabolites formed during the fermentation process. For the scientific community, it is considered a healthy food.
The fermentation of vegetables occurs through the action of numerous LABs, whose composition varies according to the type of ingredients used and the environmental conditions in which fermentation occurs.
Kimchi is produced using ingredients that, although washed, have not been sterilized and, therefore, have maintained their natural microbiome. The stages of fermentation are similar to the production of sauerkraut, meaning, initially, the predominant species are Leuconostoc mesenteroides and Leuconostoc citreum, which exploit the added sucrose; subsequently, heterolactic fermentation continues through the development of Weissella koreensis, Weissella cibaria, and Lactobacillus plantarum; hence, the dominant LABs are all heterofermentative [34, 35].
During fermentation, kimchi can ferment differently, meaning the type and concentration of the metabolites found are strongly influenced by the strains of the LAB and fermentation time; usually, lactate, acetate, mannitol, ethanol, vitamins, and carbon dioxide will be detected [34] in different percentages; these different compositions influence their sensory characteristics.
Regular kimchi consumption has been shown to improve the lipid situation and decrease fasting glucose levels [36, 37]. Hypolipidemic effects have also been demonstrated in studies on obese and diabetic rats [38, 39, 40].
Studies analyzing the effects of kimchi on irritable bowel syndrome (IBS) are of interest: patients following regular consumption of this food reported a significant decrease in the main symptoms [41].
Notably, the naturally present microbial population, in particular Weissella cibaria, has an anti-inflammatory action [42].
Kimchi appears to have a preventative effect on the onset of colorectal cancer [43]. In contrast, its consumption does not significantly affect intestinal function in patients already who have rectal cancer [44].
Sohn et al. [31] isolated a nitrate-reducing bacterium from kimchi, i.e., Lactiplantibacillus plantarum LPLB5, whose probiotic potential is demonstrated by its antioxidant and anti-inflammatory effect, adhesion ability, auto- and co-aggregation.
Park et al. [45] showed the effectiveness of using maltodextrins as a starch source instead of gelatinized glutinous rice to slow the fermentation rate of kimchi. The addition of maltodextrins to kimchi extended the fermentation period and produced a lower content of metabolites through the degradation of LAB [45].
It can be said that for kimchi, as for sauerkraut, the definition of functional food is linked to it being a fermented food that is rich in lactic bacteria, dietary fiber, minerals, and vitamins.
Kombucha (Fig. 3) is an example of symbiosis between different microbial species; in the production of this fermented drink, the starter culture (SCOBY) consists of both bacteria and yeast [46].
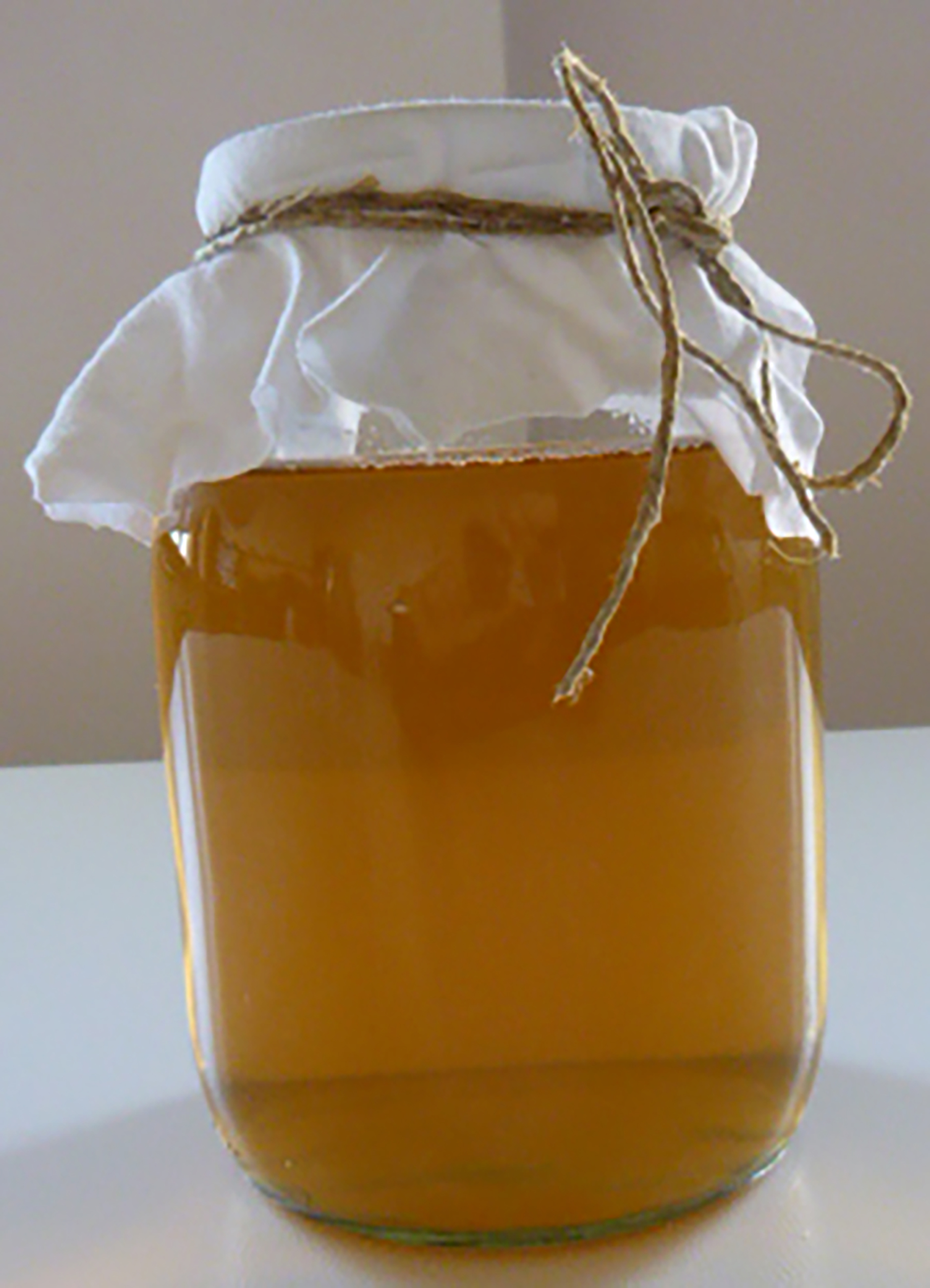
Kombucha. Kombucha, originally from China, nowadays is popular in many Countries of the world.
Originally from China, kombucha uses sugared black tea as the starting substrate. Subsequently, during its production, three different fermentation processes alternate: alcoholic, lactic, and acetic, due to the simultaneous presence of yeast and bacteria.
Sucrose is hydrolyzed into fructose and glucose by invertase of Saccharomyces cerevisiae, and these monosaccharides are used by Acetobacter and Gluconobacter (Gram-negative aero bacilli), producing organic acids: acetic, gluconic, and glucuronic [47, 48, 49].
The production of ethanol and acetic acid inhibits the growth of pathogenic bacteria in kombucha [46, 50]. Meanwhile, due to the enzymatic action of yeasts, the polyphenol content, already naturally present in black tea, increases the antioxidant action of the drink and favors the production of bacterial cellulose [51, 52]. Thus, the formation of cellulose supports the formation of the classic floating kombucha biofilm, in which bacteria and yeasts can co-exist and oxygenate on the surface [53].
The other aspect that should be considered is the usefulness of such microbes even after they die, as they become, through decomposition, sources of vitamins and nutrients beneficial for microbial renewal [54].
The chemical composition of kombucha is both complex and variable, depending on the symbiotic culture used for its production, the fermentation temperatures, and the type of tea being used.
This fermented drink can be considered a source of bioactive components, such as glucuronic acid, polyphenols, water-soluble vitamins (B1, B2, B6, B12, C), amino acids, minerals, and LAB [47].
Glucuronic acid (GlcUA) improves liver function by promoting liver detoxification from xenobiotics [55], while polyphenols are known to reduce the oxidation of the LDL fraction of cholesterol by regulating its metabolism and reducing the risk of cardiovascular disease [56].
Majid et al. [57] prepared kombucha with butterfly pea powder and
Lactiplantibacillus plantarum subsp. plantarum Dad-13 culture powder.
They found that adding probiotics at 10
The production of kefir may use goat’s milk, sheep’s milk, cow’s milk, or a soy-based drink (Fig. 4). During home preparation, live kefir (grains) is added as a starter to pasteurized milk. Fermentation is carried out at room temperature for approximately 24 hours, and subsequently, after the acidification of the milk, the grains are removed to be re-used for further production [58].
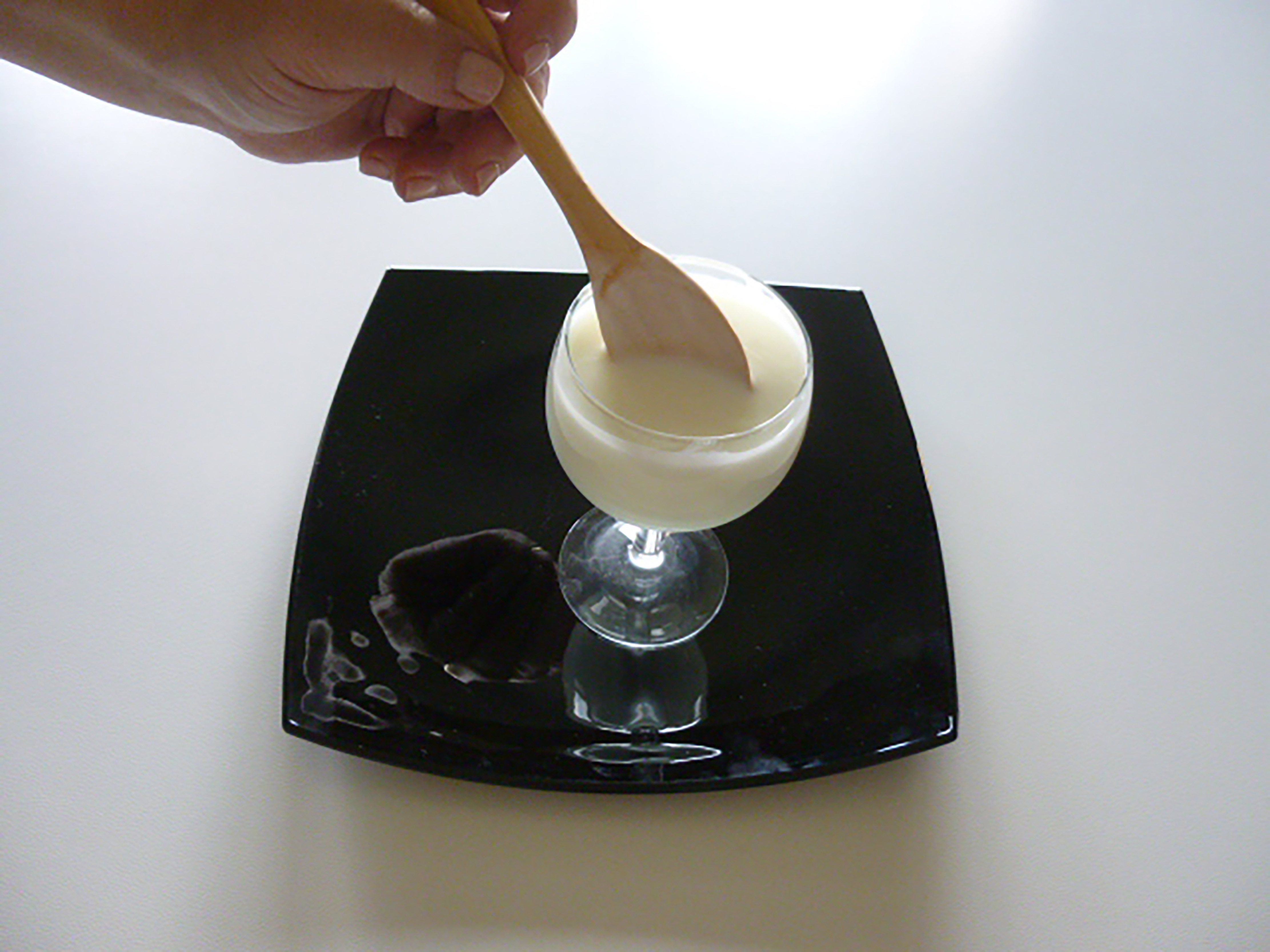
Kefir. Kefir is obtained by the fermentation of milk.
Industrially, to avoid possible contamination and produce an organoleptically constant finished product, the use of selected and freeze-dried starters is preferred [59].
Domestically, water kefir may also be produced. This is particularly suitable for vegans or those with lactose intolerance. In this case, to allow for fermentation, sucrose is added to water. Microbiologically, the grains of water kefir have characteristics very similar to those of milk kefir [60]. In the kefir grains, the main polysaccharide is kefiran, a heteropolysaccharide composed of equal proportions of glucose and galactose, produced mainly by Lactobacillus kefiranofaciens [61].
The production of kefir is a synergy between numerous metabolically different microorganisms involving LAB, acetic acid bacteria (AAB), and yeasts. Their specific composition, as for other fermented foods, is variable because they are conditioned by the starting substrate and the environmental conditions of fermentation [62, 63, 64].
Biochemically, lactose is used by LAB in the homofermentative production of lactic acid and heterofermentative production of acetic acid, lactic acid, ethanol, and carbon dioxide [65, 66]. The formation of glucose is exploited by yeast, which produces ethanol and carbon dioxide [67]. The presence of acetic acid is due to the oxidation of ethanol by AAB [68]. All organic acids found during the fermentation process, by acidifying the food, hinder the development of pathogenic bacteria [69]. In addition to the main metabolites already mentioned, peptides, amino acids, vitamins B1 and B2, folic acid, vitamin K, and riboflavonoids can be found in the final product [70].
Numerous studies have shown that kefir has many beneficial properties for the consumer’s health:
Bengoa et al. [81] showed that kefir can be a source of probiotic strains, such as Lacticaseibacillus paracasei CIDCA 8339, CIDCA 83123, and CIDCA 83124, whose EPS-production on MRS broth functions alongside the incubation temperature.
Natto is a traditional Japanese food made from fermented soybeans (Fig. 5). The production of natto includes the following steps: washing, soaking of whole soybeans in water overnight, cooking for 20–30 minutes, and refrigeration at 4–5 °C.
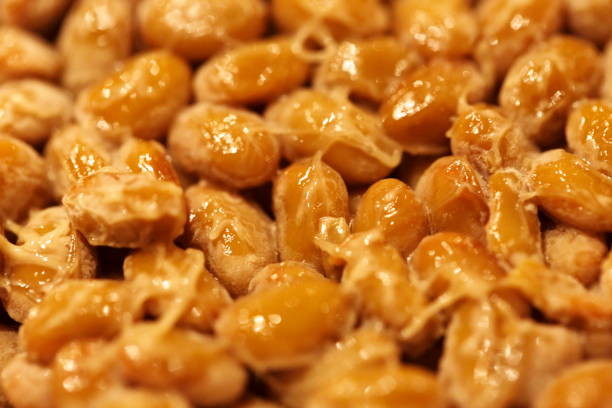
Natto. Natto, is a traditional Japanese food made from fermented soybeans.
Subsequently, a starter bacterial strain, Bacillus subtilis var. natto, a rod-like, aerobic, spore-like, Gram-positive bacterium, is added. Finally, fermentation proceeds at 40–45 °C for about 18–20 hours [7, 82].
Heat treatment is intended to kill other microbial forms on the starting substrate, mainly lactobacilli, which would trigger lactic fermentation.
The production of natto occurs through an alkaline fermentation in which B. subtilis initially transforms the present carbohydrates by lowering the pH before producing ammonia and amines to hydrolyze the proteins, ultimately causing the pH to increase to around 8.3. The present lipids and carbohydrates undergo hydrolysis by releasing fatty acids and simple carbohydrates [83].
A nutritional composition analysis shows a protein content of 19%, a lipid content of 11%, and a carbohydrate content of 13%, which includes both simple carbohydrates and fiber [84].
Natto is a source of minerals (potassium, calcium, iron, magnesium, manganese) and vitamins C and K.
This chemical composition makes it a food with very good nutritional properties and can be included in a balanced diet.
Another aspect of its composition, which has recently been considered, is the presence of nattokinase, a proteolytic enzyme present in the food that has been studied for its preventive activity in the onset of cardiovascular diseases in both humans and animals.
Natto appears to have the following assets:
Yogurt is made from fermented milk. It was the first fermented food used by man, and today, it is perhaps the best-known and most widespread (Fig. 6). It is associated with protection from type 2 diabetes [90, 91]. Yogurt, as it is prepared today, is the result of tradition combined with technological and scientific advances in microbiological, nutritional, and plant engineering alongside packaging, which allow a consistent product to be obtained. It is a product of both compositional and organoleptic quality, with a high biological value that offers an alternative form of milk conservation.
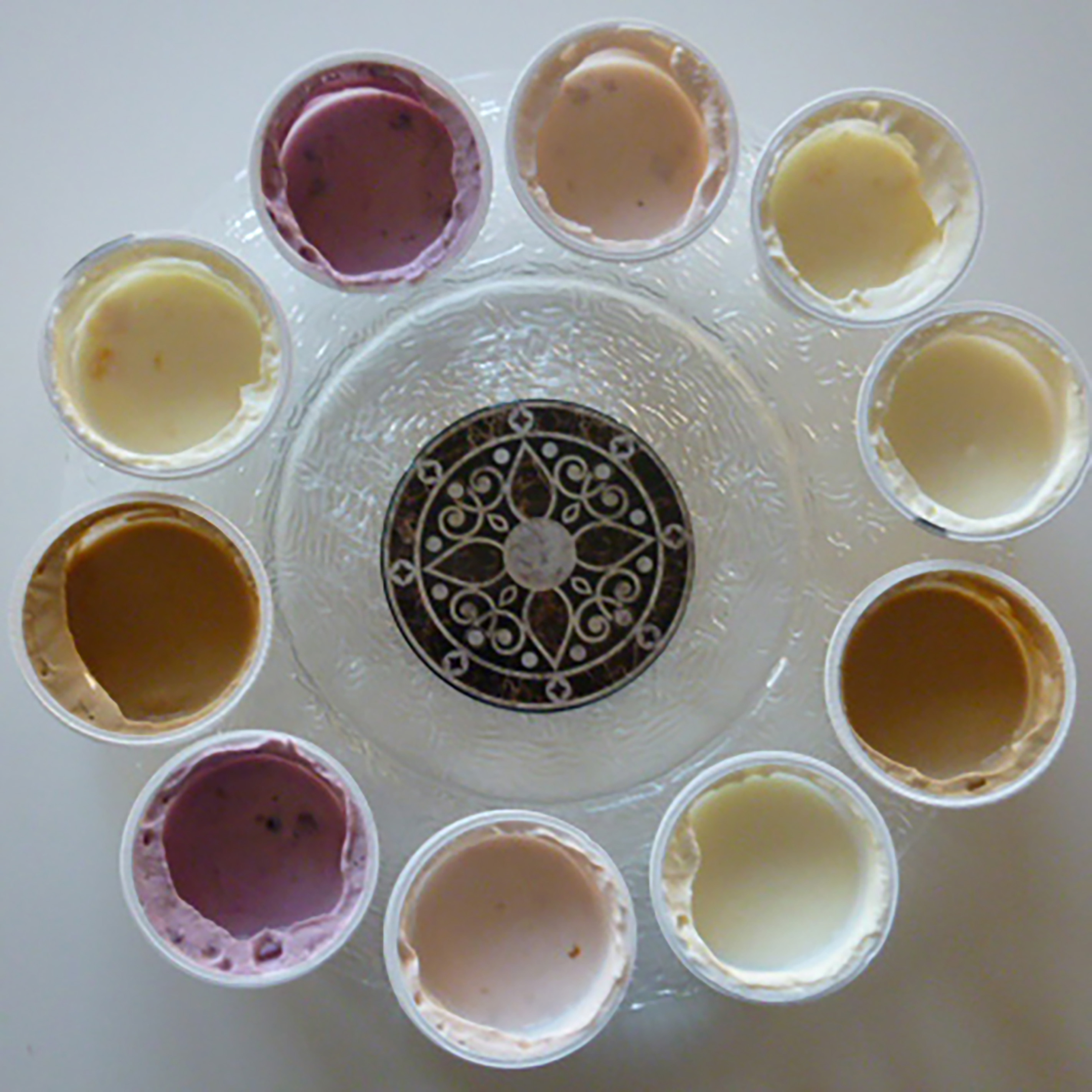
Yogurt. Different tastes of yougurt.
Following inoculation and incubation, biochemical changes occur in the milk; in particular, lactic acid, together with acetaldehyde (giving the typical acidic and aromatic taste), causes the casein micelles to destabilize, determining the coagulation of the milk and the formation of gel that provides yogurt with its consistency.
To prepare yogurt, the milk initially undergoes treatment (addition or subtraction of cream, homogenization, and pasteurization), followed by fermentation. Here, the yogurt can be marketed as is or with other ingredients, such as pieces of fruit, honey, or coffee extracts. In some cases, the yogurt is packed in a double carton, where the second part contains cereal derivatives, which are added to the yogurt immediately before eating to maintain their flavor and crunchiness. Yogurt has to be stored in the refrigerator at 4 °C until consumed.
Akdeniz [92] studied a yogurt starter culture containing Streptococcus thermophilus and Lactobacillus delbrueckii subsp. bulgaricus, and probiotic yogurt culture Bifidobacterium animalis subsp. Lactis is enriched with carob flour by performing ultrasonication at different stages of production. This study found that ultrasonicated samples had a higher pH, higher counts of lactic acid bacteria, higher probiotic bacteria, and lower lactic acid values than non-ultrasonicated samples. Ultrasonication produced different results depending on the production stage; however, it improved the physical–chemical–sensory properties of the yogurt in all cases.
Cerda-Bernad et al. [93] studied yogurt when fortified with different concentrations of saffron and extracts of its floral by-products. They found that alginate microencapsulation provided protection and maintained the antioxidant properties of saffron flowers in the yogurt matrix over 21 days (4 °C). Furthermore, the saffron extracts did not influence the microbiological profile and physical–chemical parameters of the yogurt, which showed a good composition of organic acids and soluble sugars useful for improving shelf life.
Kariyawasam et al. [94] prepared yogurts supplemented with probiotic organisms, such as Lacticaseibacillus rhamnosus, Lantiplantibacillus plantarum KCTC 3108, and Lantiplantibacillus plantarum 200655 and compared this prepared yogurt to a control without probiotics. They found that probiotics reduced fermentation time, decreased syneresis, and increased water-holding capacity and viscosity. The yogurt inoculated with Lantiplantibacillus plantarum 200655 had the highest antioxidant activity, which protects against inflammatory bowel disease by reducing oxidative stress in intestinal cells [94].
Mango peel powder was tested with the dual aims of reducing food industry waste and simultaneously improving the properties of the yogurt. In this context, the phytochemicals in the mango peel powder showed prebiotic effects during colonic fermentation. Yogurt fortified with mango peel powder reduced the pH and increased both the LAB count and the production of short-chain fatty acids [95].
Olives are the fruit of Olea europaea L. and must be cured before they are ready for human consumption. Fermentation used in the curing process reduces the pH, which inhibits bacterial growth and, thus, contributes to the extension of the olives’ shelf-life (Fig. 7).
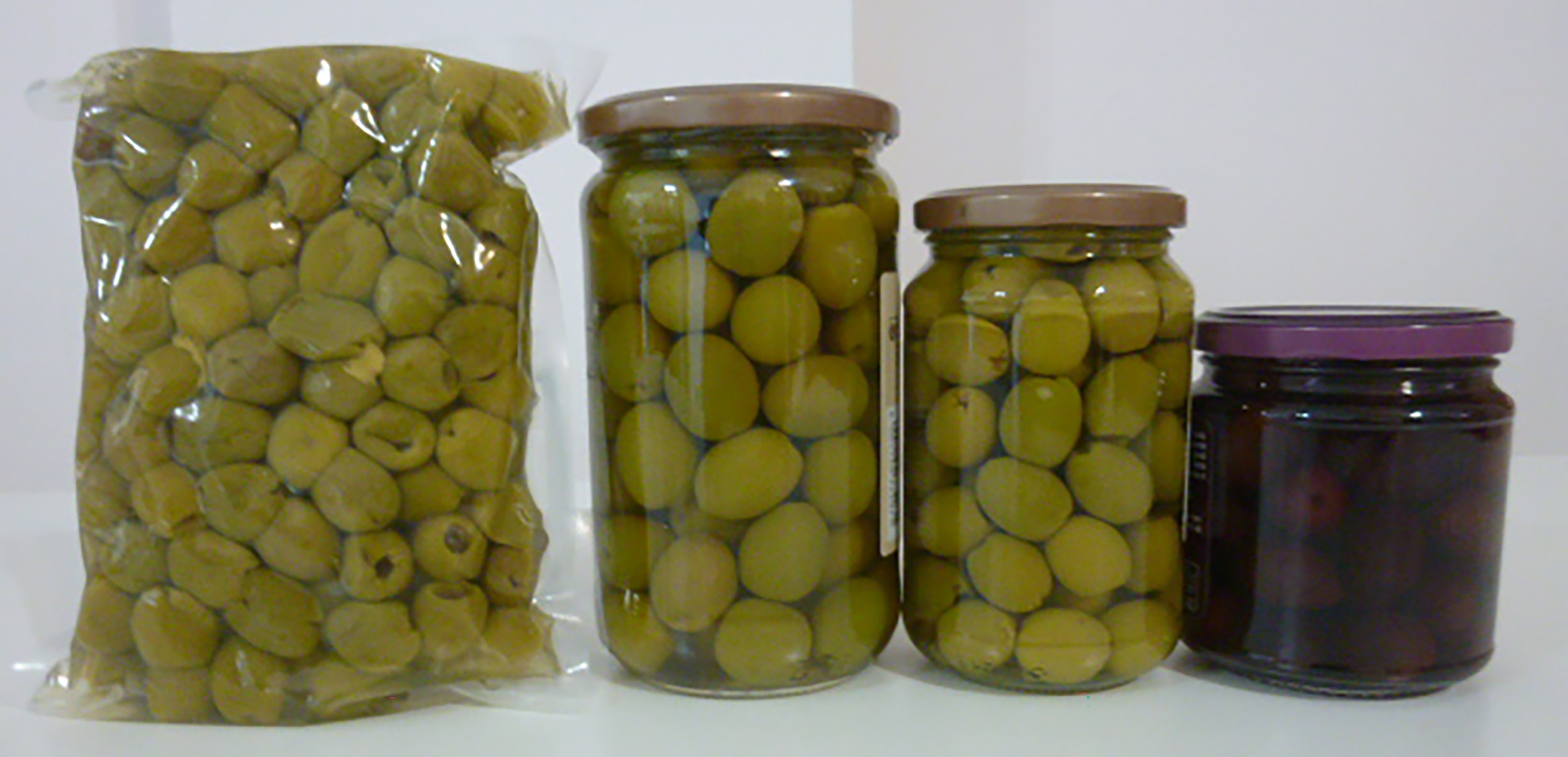
Table olives.
Bacterial fermentation of table olives begins when the pH is around 6.0 and lasts until LAB is developed. During this phase, Gram-negative bacteria and some molds and yeasts progressively decrease. Meanwhile, LAB belonging to the Pediococcus and Leuconostoc genera develop and transform the sugars into lactic acid, further reducing the pH. Finally, there is a proliferation of a wide variety of LAB, including L. plantarum and L. pentosus. However, yeasts of the genus Saccharomyces, Pichia, and Candida can also develop, which increases the growth of the LAB through the release of nutritional compounds and the synthesis of amino acids and vitamins. Fermentation lowers the pH to 4, stabilizing the food by creating an unsuitable habitat for unwanted microorganisms and increasing the organoleptic and sensory properties of the olives. The fermentative methods mainly applied to table olives are Spanish or Sevillan, Castelvetrano, natural, Californian, and Greek. The Spanish or Sevillian method is the fastest applied to green olives. Diluted solutions of sodium hydroxide are used, followed by repeated washing to remove excess soda, and finally, the olives are preserved in brine with a 6–8% salt concentration. Using this method, table olives are obtained in 2–3 months. In the Castelvetrano method used in Sicily (Italy), the olives are treated with a sodium hydroxide solution for a few hours; then, they are repeatedly washed to eliminate the residual sodium hydroxide. Next, they are immersed in brine and left to ferment for 3–4 months, after which they will be ready for packaging and consumption or for subsequent processing (pitting or washing). In the natural method applied in Sicily (Italy), fruits are harvested at the green or pigmented stage and placed in brine (8 to 12%). The production of natural table olives is a spontaneous, traditional acid fermentation, which is performed by the microorganisms present in the fruits and environment [96]. The Californian method involves using a low concentration of sodium hydroxide solution. Subsequently, the drupes are subjected to air insufflation under pressure. These phases alternate until the final product is obtained and preserved in brine.
In the Greek or natural method, LAB spontaneously grows in the brine. The debittering of the black drupes is obtained with alternating phases of immersion in the brine and washing with water. This process is very long, and the final product is often preserved in brine.
Piga et al. [97] studied the Greek method (150 days) applied to Bosana, Manna, and Sivigliana Sarda cvs. Total phenolic content decreased in all samples. The behavior varies depending on both the initial phenolic content and the biometrics of the fruits in each cultivar. Bosana cv produced the smallest fruit. Drupes of Bosana cv showed the highest phenolic content at the initial stage of fermentation and during the entire fermentation process. This is important from the functional point of view because phenols are antioxidants and are useful in the human diet [97].
Poiana and Romeo applied the Natural method to the following olive cultivars: Nocellara Etnea, Nocellara Messinese, Moresca, Ogliarola, and Tonda Iblea. The fermentation process was found to be influenced by olive cultivars. In Moresca cv, brine, the highest amount of mesophilic bacteria, yeast, and mold were found throughout the brining period. The LAB count showed different behavior in
De Bruno et al. [98] studied different fermentation treatments to olive
tables cv Carolea, Grossa di Gerace; Nocellara Messinese, i.e., fruits with
different weights and dimensions. In the experimental design, they studied
different NaCl concentrations in acidified and non-acidified brines. They found
the best physicochemical and sensory results with the highest NaCl content (8%).
Carolea cv showed the best properties both from the physicochemical and
antioxidant points of view. Total phenolic content,
2,2
Fermentation was applied to the cv. Kalamata natural black olives, which were inoculated with five yeast strains in starter cultures with multifunctional potentials belonging to Candida boidinii and Saccharomyces cerevisiae. Drupes were processed according to the traditional anaerobic Greek-style method. Fermentation was conducted in plastic vessels containing olives and NaCl brine acidified with vinegar. Drupes were inoculated in monoculture with four strains of C. boidinii (Y27, Y28, Y30, and Y31) and one strain of S. cerevisiae (Y34). The yeast starters at the beginning of fermentation showed a positive effect on the reduction of Enterobacteriaceae in the brines compared to spontaneous fermentation. The authors found an acceptable pH only in the cultures prepared with C. boidinii Y27, C. boidinii Y28, and C. boidinii Y31 [99].
Ruiz-Barba et al. [100] studied green table olives from the Manzanilla cultivar and monitored the microbial community and volatilome of brines during the spontaneous fermentations of the Spanish and natural styles. Fermentation in the Spanish style was conducted using LAB and yeasts, whereas fermentation in the natural style was conducted using halophilic Gram-negative bacteria, archaea, and yeasts. Between the two types of table olives, microbial profiles showing significant differences influencing their volatilome and their physical–chemical–biochemical characteristics were found. The greatest differences in relation to the volatilome were between the two olive styles were found for acids, with a high content of acetic acid in the Spanish style olives, whereas the natural style olives had a high content of carbonyl compounds (mainly methyl ketones). Their study demonstrated that the growth of lactic acid bacteria was unnecessary for a better volatile profile [100].
Sourdough bread is obtained from a dough that has been leavened for many hours or days and incorporates the natural presence of bacteria and yeasts in the air instead of adding leavening agents (Fig. 8). The fermentation process consists of mixing water and flour. With time and exposure to air (and often also thanks to the addition of probiotics or lactic bacteria from sugar fermentation), the mixture is characterized by a form of slow but effective spontaneous fermentation. Therefore, the bread made using these techniques is derived from a dough without added active yeasts, not even natural sourdough. The natural contamination with bacteria and yeasts present in the environment allows the mixture of water and flour to develop a population of native, endogenous, and exogenous yeasts and bacteria. Martorana et al. [101] studied autochthonous LAB and yeasts isolated from sourdough samples and investigated their technologically useful properties. They demonstrated the effect on physicochemical–sensory parameters in relation to LAB and yeasts isolated from mature sourdough products obtained from artisanal bakeries [101].
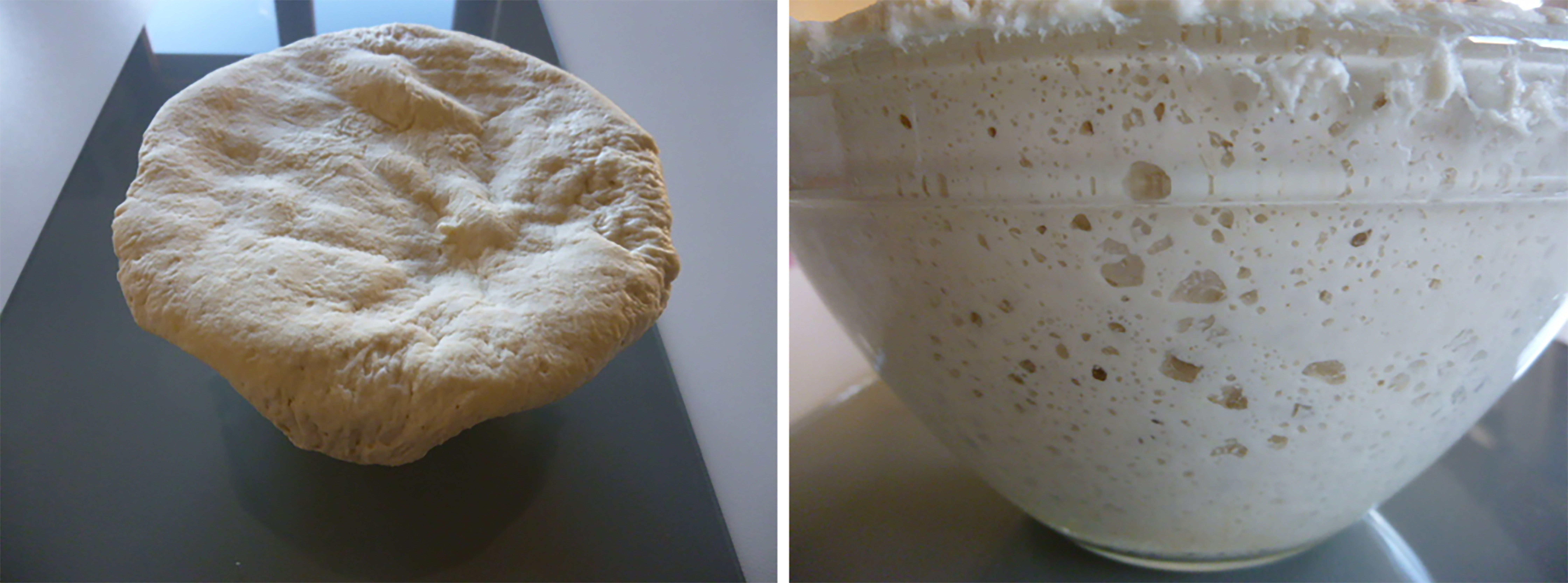
Sourdough bread.
A scientific approach was applied to artisanal sourdough breadmaking to improve the properties of bread. Fermentation of sourdough was conducted using a multistrain starter culture with strains previously isolated from artisanal sourdoughs and selected for their useful breadmaking properties. Thus, the prepared sourdoughs had a phenolic content and antioxidant activity similar to the artisanal sourdoughs made using durum wheat. This shows the usefulness of the starter culture in a laboratory sourdough formulation. Using a multistrain starter in artisanal breadmaking was found to improve the antioxidant and textural properties of the bread [102].
Rizzello et al. [103] studied the digestibility of three fermented breads that were prepared as follows: baker´s yeast bread, leavened with baker’s yeast 1.5% at 30 °C for 90 min; sourdough bread leavened with S4 (sourdough fermented 4 h) (20%) and baker’s yeast 1.5% at 30 °C for 90 min; sourdough bread leavened with S24 (sourdough fermented 24 h) (20%) at 30 °C for 4 h. The authors observed that the intake of these three breads did not influence gastric emptying (basal antral area and maximal postprandial antral area) and partially influenced the residual antral area. In gallbladder emptying, no differences were found in relation to fasting volume, ejection volume, residual volume, ejection rate, refilling volume, refilling rate, and half-refilling rate. However, partial differences were found in relation to the time for the residual volume and half-emptying time. The authors showed that the limitations in their study mainly involved subjects without gastrointestinal symptoms and the use of questionnaires that had not been largely validated [103].
Vinegar is the product of the fermentation of alcoholic liquids, such as beer and wine, due to the action of aerobic bacteria in the Acetobacter genus (Fig. 9). It has an acidic taste, and it is used as a condiment and for the preservation of food products by lowering the pH of the preserving liquid.
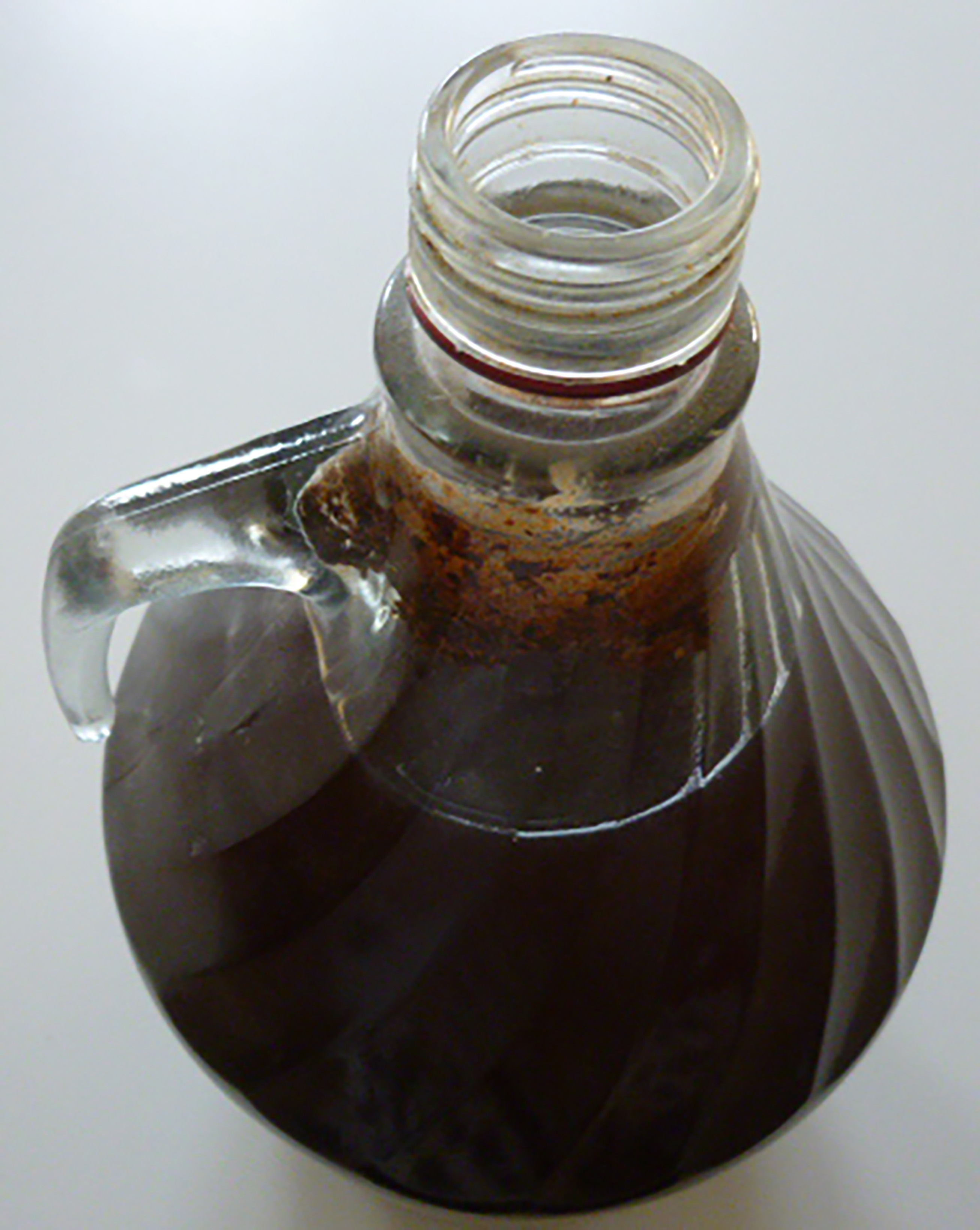
Vinegar.
In Italy, the law prescribes that the name ‘vinegar’ is reserved for products obtained from the acetic fermentation of wines with a minimum acetic acid content of 6 g/100 mL. In other countries, vinegar is obtained from wine and by fermenting cider, malt, beer, molasses, rice, and even by diluting pure acetic acid. Wine vinegar is produced from white, rosé, and red wines. Its quality depends on that of the starting wine and the production technology. Aromatic kinds of vinegar are primarily those from champagne, sherry, and balsamic vinegar from Modena. The latter is obtained from filtered grape must, cooked, and simultaneously subjected to alcoholic and acetic fermentation.
The addition of vinegar creates an acidic environment to which microorganisms are susceptible and contributes, together with heat, to breaking down any microbial loads that may be present in foods. This is of considerable importance in food preservation.
Bergamot (Citrus bergamia, Risso) is a plant growing in the coastal strip of Reggio Calabria province (south Italy) [104, 105]. The different parts of the fruit have shown many beneficial effects on human health [106, 107]. Di Donna et al. [108] and Giuffrè et al. [109] studied how to valorize the by-products of bergamot fruit and obtained an alcoholic product by using filtered bergamot percolate, filtered bergamot juice, water, filtered mandarin juice, and sucrose. This mixture was inoculated with two selected yeast strains, Sc734 of Saccharomyces cerevisiae and L797 of Hanseniaspora guilliermondii. Fermentation was maintained at 25 °C for 1 month. The alcoholic product thus obtained was inoculated with selected Acetobacter strains to obtain vinegar. These studies demonstrated how to conduct the bioconversion of citrus wastes into vinegar while maintaining the biochemical, nutraceutical, and sensory properties. Flavonoids contained in bergamot of an alcoholic product were not found in the same quantity in bergamot vinegar, even if a significant amount of Brutieridin and Melitidin was detected in the vinegar [108, 109]. This procedure could be applied to the alcoholic product and vinegar obtained from other citrus fruits after proper adjustments.
Wang et al. [110] studied the variation in the volatile flavor components by applying a solid-state vinegar fermentation using a rotary drum bioreactor compared with a traditional fermentation. During both fermentation processes (30 days), no differences were found in the amino, nitrogen, alcohol, reducing sugar, and total acid contents. Acetic and lactic acid were the main organic acids produced in both cases. The fermentation conducted in the rotary drum bioreactor was characterized by controllable fermentation conditions, a shorter fermentation cycle time, and timely removal of the generated heat. In the earlier period of fermentation (25 days), the total phenolic and flavonoid contents were higher in the vinegar obtained by rotary drum bioreactor. Thereafter, these compounds disappeared in the so-produced vinegar, with a loss of functional properties, while remaining only in the traditionally produced one [110].
Recently, there has been much talk about the “revolution of fermented foods”, yet what seems like innovation is nothing more than a rediscovery of foods that are part of culinary traditions.
Fermented foods are seen as more ecological than mass-produced foods. They are seen as homemade and artisanal and thus of superior quality. Artisanal or semi-artisanal fermented foods can help sustain local economies.
If the living microorganisms responsible for fermentation reach the intestine in sufficient quantities, they can multiply and help re-balance the intestinal microflora. Such foods are labeled ‘probiotic’. In addition to local gut activity, probiotic foods can also improve the body’s physiological balance.
The desire to return to natural, artisanal, homemade, traditional foods is a want for products of superior quality. Modern scientific research has shown that these fermented foods may also play an important role in human health by restoring the intestinal microbiota and preventing some of the most common diseases of modern times.
DG and AMG designed the research study. DG and AMG analyzed the data. DG and AMG wrote the manuscript. Both authors have participated sufficiently in the work to take public responsibility for appropriate portions of the content and agreed to be accountable for all aspects of the work in ensuring that questions related to its accuracy or integrity. Both authors contributed to editorial changes in the manuscript. Both authors read and approved the final manuscript.
Not applicable.
Not applicable.
This research received no external funding.
The authors declare no conflict of interest.
Publisher’s Note: IMR Press stays neutral with regard to jurisdictional claims in published maps and institutional affiliations.