Vascular dementia (VaD) refers to the loss of reasoning, planning, judgment, and memory as a result of reduced blood flow, inflammation, activation of immune cells, and neurodegenerative processes. Here, we tested the preventive effects of alpha-naphthoflavone (ANF) on a model of vascular dementia induced by four-vessel occlusion (4VO) in rats. Animals were treated orally for 5 consecutive days with 10 mg/kg of donepezil (a traditional therapeutic drug) and 40 and 80 mg/kg ANF. The treatment with ANF exerted effects that were similar to those induced by donepezil. This included prevention of cognitive impairment, alterations in the plasma levels of homocysteine and nitrate and the activity of acetylcholinesterase and myeloperoxidase and the tissue level of glutathione, and thiobarbituric acid reactive substances in the brain tissues. These findings suggest that herbal-derived ANF is as effective as traditional drugs in vascular dementia.
Vascular dementia entails a decline in cognitive skills caused by conditions that block or reduce blood flow to the brain, depriving brain cells of vital oxygen and nutrients. In vascular dementia, changes in thinking skills sometimes occur suddenly following strokes that block major brain blood vessels (1). Problems with cognition also may begin as mild changes that worsen gradually as a result of multiple minor strokes or other conditions that affect smaller blood vessels, leading to cumulative damage (2). Globally, the pervasiveness rate of VaD is higher in developing countries. Worldwide, around 6 to 12 cases of VaD have been reported per 1000 people. Although it can occur at any age, vulnerability is generally higher in old age, i.e. the age of 70. The rate of VaD is faster than Alzheimer's illness (AD), and the survival rate is exceptionally lower than the AD (3). Furthermore, with the involvement of various pathological factors such as hypertension, diabetes, atherosclerosis, uremic toxin, cardio-renal failure, and neurotrauma, VaD occurs at any age (4). Some of the modifiable factors such as chronic alcoholism and smoking also play a key role in the progress of VaD. The above-mentioned factors are involved in the impairment of cerebral blood flow (5). The chronic alteration of cerebral blood flow induces the neurovascular inflammatory and immunological reactions. In addition, nutrients and energy are highly utilized by the neurological system (6). The lack of blood flow causes the hypoxic and ischemic environments of the microvascular and neurovascular regions in the brain. Furthermore, the abnormal cellular ion fluxes, enzymatic actions, mineral and metal handling and protein replication process are also activated (7). Crucially, the mitochondria-dependent and independent cell death processes also play a role in the neurodegeneration and cognitive impairments. Clinically, VaD occurs with lack of cerebral blood flow (8). Experimentally, numerous studies have documented that cerebral blood flow is altered by the occlusion of two carotid arteries (2VO).
Paradoxically, the 2VO processes enhance collateral blood circulation and may not produce significant neurological damage in rats (9). Hence, the present study employed clinically relevant models of VaD by four-vessel occlusion (4VO) induced VaD in rats. This is done to avoid collateral blood circulation and angiogenesis process and achieve accurate results of the test compounds. The currently marketed nootropic agents i.e.,donepezil [acetylcholinesterase (AChE) inhibitor], galantamine (partial AChE inhibitor, positive allosteric modulator of nicotinic acetylcholine receptors and inhibitor of beta-amyloid aggregation) and rivastigmine (dual inhibitor of acetylcholinesterase and butyrylcholinesterase) are used for the treatment of cognitive disorders including AD and VaD (10). Unfortunately, these agents are documented to produce the various unwanted adverse drug reactions like organ liver damage, hematological and psychiatric changes and reproductive toxicity (11). However, these are the recommended medications for the management of VaD. Since none of the medicines are found to produce the potential management of VaD (due to lack of efficacy and multiple toxicities), newer medicines are required to manage neurovascular disorders including VaD. Some of the herbal moieties and synthetic derivatives are reported to prevent cognitive disorders via amelioration of neurovascular disorders. Alpha-naphthoflavone (ANF), is also known as 7,8-benzoflavone, is a natural flavone available in Russian knapweed in large quantities (12). It possesses multiple biological and pharmacological actions under certain pathological conditions. The primary pharmacological actions are cardiovascular protection, cancer prevention, hepatoprotective and neuroprotective actions via free radical scavenging, reduction of inflammatory and immunological reactions and anti-apoptotic actions (13). In addition, it attenuates the H2O2-induced neuron SH-SY5Y cell damage (14). In addition, naphthoflavone showed ameliorative potential against oxidative stress-induced damage of the neurovascular system (14, 15). Thus, ANF may produce potential therapeutic action in the management of vascular dementia. Experimentally, it is not evidenced by the effect of ANF in vascular dementia. Therefore, the present study was designed to investigate the role of ANF in 4VO induced VaD in rats.
Wistar rats were used in the present study. The weight of the rats ranges from 150 - 180 g. Animals were fed with standard laboratory diet (Adita Biosys private limited, Tumkur, Karnataka, India) and allowed free access to tap water ad libitum. 12 hour normal light/dark cycle was maintained in the departmental animal house. In addition, the experiments were kept in a semi-soundproof laboratory and animal house. The experimental protocol was duly approved by the institutional animal ethics committee. Care of the animals was carried out according to the guidelines of the Committee for the Purpose of Control and Supervision of Experiments on Animals (CPCSEA), Ministry of Environment and Forest, Government of India (Reg. No. 155/PO/Re/S/1999/CPCSEA; dated 11/09/2015; IAEC Proposal No.: 252/2017).
Acetylthiocholine and 1,1,3,3-tetra methoxy propane were procured from SD Fine Chemicals Limited (SDFCL, Mumbai, India). Folin-Ciocalteu's Phenol reagent, 5,5′-dithiobis (2-nitrobenzoic acid) (DTNB), reduced glutathione (GSH) and bovine serum albumin (BSA) were purchased from Sisco Research Laboratories Pvt. Ltd., Mumbai. Homocysteine was obtained from Sigma Aldrich, Mumbai. All other reagents were used as an analytical grade. All the solutions were freshly prepared before use.
The four-vessel occlusion of cerebral arteries were made with the standard operating procedure as described by Pulsinelli and Buchan (16) with a slight modification of Schmidt-Kastner et al., (17) and Gulati et al. (18). Animals were anaesthetized by intraperitoneal (i.p.) injection of ketamine (30 mg/kg; i.p.) and xylazine (10 mg/kg; i.p.) solution. The bottom surface of the neck region was supported with cotton plugs to access the occipital skull portion of the upper cervical vertebrae. The vertebral arteries were exposed with mild dissection of cervical back muscles. The vertebral artery was occluded by the application of cautery pen tip on the exposed blood vessels. The opening of skin and muscle layers were closed and topical antiseptic solution and the antibiotic powder was applied once after the closing of skin openings. Then, rats were kept in a separated cage until the recovery process from the anesthetic condition was completed.
On the 3rd day, rats were anesthetized to expose the carotid arteries. The skin and muscle layers were opened to expose the common carotid artery (right as well as left) by adjusting the location of the sternocleidomastoid muscle and infra-hyoid muscles parallel to the trachea. The carotid artery was exposed by damaging vagus nerve. A septic silk thread (No.: 04) was passed below the blood vessels and 07 mm diameter nylon wire (0.5 cm length) was placed parallel to the carotid artery. The silk thread was then tied with blood vessels for 5 minutes to complete ischemic environment. Thereafter, the nylon wire was carefully removed from the carotid artery attachment. In this condition, 2/3 of the carotid blood vessels were blocked and causes about 70 percentage reduction in cerebral blood flow. Sham-operated rats were made with all identical surgical procedures with the exception of carotid artery occlusion. Post-operative care was followed similar to that of first-day surgery. Behavioral observations were made at different time intervals.
Six groups of animals (Wistar rat; 150-180 g) were employed in this study. Each group comprised of 8 animals (n=8). Group I: Normal control group; This group did not receive any vehicle and drugs. Group II: Sham control group; This group employed all surgical procedures without the occlusion of blood vessels i.e., 4VO. Group III: 4VO group. Group IV and V: 4VO + ANF [40 and 80 mg/kg; per oral (p.o.) for 5 consecutive days]; Group VI: 4VO + donepezil (10 mg/kg; p.o. for 5 consecutive days) treated group. The administration of alpha naphthoflavone with a dose of 40 mg/kg for 3 to 9 consective days showed potential pharmacological action (19). The administration of test compounds for 5 days showed clear and maximal difference in the changes of cognitive functions, especially carotid artery occlusion induced vascular dementia (20). Based on this literature, the present study was designed for the administration of ANF with two different doses (i.e., 40 and 80 mg/kg of ANF) for 5 consecutive days. Furthermore, drug administration started from day 3 i.e., completion of the 4VO process. A safety arose as the acute oral toxicity level of ANF was at 24 gm/kg of rat body weight (21). Hence, the oral administrations of ANF with a dose of 40 and 80 mg/kg for 5 consecutive days are safer. At the end of the study protocol, blood samples were collected from all the animals. Then, animals were then sacrificed for the isolation of brain samples for various biochemical estimations. The experimental protocol was illustrated in Figure 1.
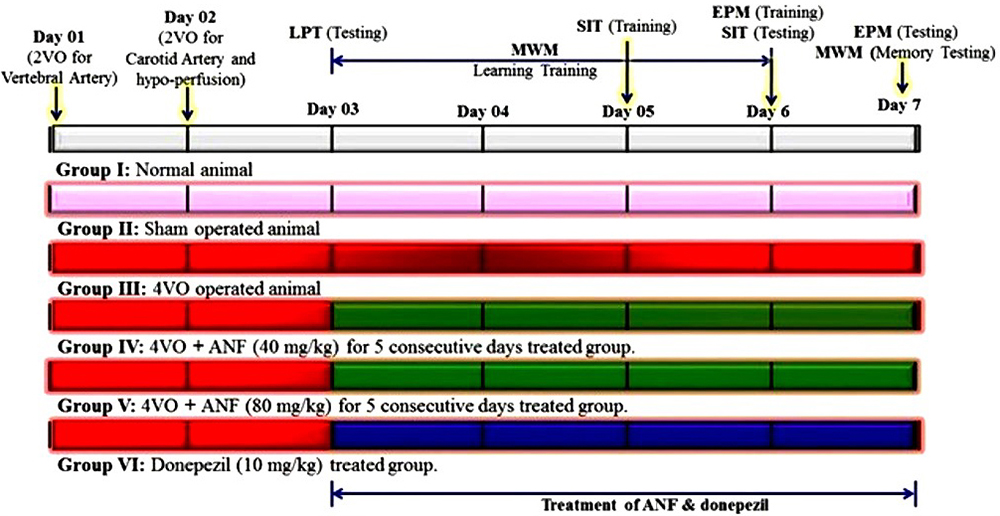
Experimental design for the role of ANF in four-vessel occlusion induced vascular dementia in rats
Rats were engaged in different neurocognitive function test. The cognitive functions were evaluated based on the Morris water maze test, Elevated pulse maze test, and social interaction tests. All the animals were sacrificed at the end of the study protocol. The animal movements were tracked by a USB camera (12 Megapixel USB Camera, Intex, India).
MWM test is one of the established methods of spatial memory assessments in rodents. This MWM test explains the ability (levels) of rodents’ learning and memory processes as described by Morris (22). After 24 hours of four-vessel occlusion (the 3rd day), the rats were acclimatized for 1 h in the test room before placing them in the MWM. The whole experiment lasted for five successive days. Each animal was trained four times to reach the platform with an inter-trial interval of no more than 20 min and escape latency or time required to reach the platform was calculated. On the fifth (final) day of the experiment, low-fat milk was mixed with the water; only one testing episode was required for each animal. The platform was removed from the pool and the animal was placed in the center of the pool, and the time spent with each animal in the target quadrant (the quadrant that used to retain the platform) was then calculated. 110 seconds of observation was completed for the assessment of memory retention power and this trial is named the retrieval trial. The time spent in the target quadrant (TSTQ) was noted as an index of memory function.
EPM test is one of the common methods for the assessment of memory and anxiety disorders in rodents. This EPM test explains the level of short-term spatial memory as described by Kawaguchi et al (23). The animals were placed in the corner of any one of the open arm and were observed for the time taken to reach any one of the corners of the closed arm. This time was noted as transfer latency (TL) and is considered one of the parameters for learning and memory process in the EPM apparatus. The TL reading was noted on the 6th day of the experimental protocol as basal / training segment for learning. Transfer latency on the 7thday was noted as a testing segment for memory (24, 25).
LPT is a well-accepted method for the assessment of grip strength. It indicates the strength and coordinated function of the neuromuscular system and this method is described by Petullo et al. (26) with a slight modification by Schaar et al. (27). Lateral push stimuli were applied five times on each side of the animal body. The test was performed at the end of the experimental protocol. The percentage resistance against the lateral push (pressure) was calculated for the assessment of grip strength based on a number of stimuli and the number of resistant responses.
SIT is one of the indicators for the psychosocial behavior of laboratory animals, especially for the rodents. This method was described by Varlinskaya and Spear (28) with a slight modification by Kaidanovich-Beilin et al. (29). All the experimental animals were placed individually in the right diagonal corner with their face kept towards the wall surface. The assessment of social interaction was done measuring the time taken for complete interaction with an unfamiliar (circular box) animal. Between each animal testing, the circular chamber and the square chamber were cleaned with non-odorant BioGreen Solutions (a mixture of Natural starch; vegetable oil; and vegetable waste) to avoid olfactory biases. Each chamber boxes allow exchanging air between the interior and exterior of the middle chambers but prevent direct physical interactions between animals. All SITs have been performed between 9:00 AM to 11:00 AM with room lighting of 650 Lux.
Blood samples were collected at the end of the study protocol by the terminal blood sample collection method via tail vein method as described by Vogel and Vogel (30). Briefly, rat’s tail vein was punctured and blood samples were collected in two separate centrifugal tubes. One centrifugal tube was prefilled with 100 μl of anti-coagulant i.e., 11 % w/v of sodium citrate. The centrifugal tubes were centrifuged at 3000 rpm for 15 minutes.
Plasma samples were used for the assessment of homocysteine (HCy) estimation using ultraviolet spectrophotometer method as described by Wang et al. (31). The changes of absorbance in test tube solution were noted spectrophotometrically (DU 640B Spectrophotometer, Beckman Coulter Inc., CA, USA) at 510 nm wavelength. The standard plot was prepared with 1 to 10 μmol of HCy concentration to calculate the concentration of plasma HCy levels. Standard HCy was prepared with 70 percentage of hydro-alcoholic preparation. The results were expressed as µmol per liter.
The level of plasma nitrite changes was estimated using Griess reagent as a described by Green et al (32). The developed pink color chromogen in supernatant solution and their absorbance change was noted spectrophotometrically (DU 640B Spectrophotometer, Beckman Coulter Inc., CA, USA) at 540 nm wavelength. The standard curve of sodium nitrite was plotted with 5 to 50 µmol concentration to calculate the concentration of plasma nitrite levels. The results were expressed as µmol per liter.
At the end of the experimental protocol, all the animals were sacrificed by cervical dislocation after completion of behavioral tests. The brain was isolated immediately and was dissected for the separation of the hippocampus and cortex. All the tissue was homogenated with a phosphate buffer with pH - 7.4. The supernatant was separated by centrifugation process at 3000 rpm for 15 min. It was then used for various biochemical estimations such as acetylcholinesterase (AChE) activity, reduced glutathione (GSH), thiobarbituric acid reactive substances (TBARS), myeloperoxidase (MPO) activity levels in a hippocampus tissue sample. In addition, HCy levels were also estimated in hippocampus and cortex samples.
The AChE activity level of brain samples was estimated by a spectroscopic method as described by Ellman et al. (33). The change of absorbance was noted spectrophotometrically (DU 640B Spectrophotometer, Beckman Coulter Inc., CA, USA) at 420 nm wavelength. These readings were used for further calculation of AChE activity levels in a tissue sample. The results were expressed as μM of acetylthiocholine hydrolysis per milligram of protein per minute.
The GSH level of brain samples was estimated as a described method of Ellman (34). The developed yellow color chromogen changes of absorbance were noted by spectrophotometer (DU 640B Spectrophotometer, Beckman Coulter Inc., CA, USA) at 412 nm wavelength. The results were expressed as μmol of GSH / mg of protein.
The TBARS level of brain samples was estimated as described by Ohkawa et al. (35). The clear pink color chromogen of the supernatant was collected. Change of absorbance was noted spectrophotometrically (DU 640B Spectrophotometer, Beckman Coulter Inc., CA, USA) at 535 nm wavelength. The results were expressed as nmol per mg of protein.
The MPO levels were estimated as a described method of Grisham et al. (36) and Patriarca et al. (37). The change of absorbance was noted spectrophotometrically (DU 640B Spectrophotometer, Beckman Coulter Inc., CA, USA) at 460 nm wavelength. The results were expressed as myeloperoxidase activity units per milligram of protein at one-minute duration.
HCy levels were estimated as described by Wang et al. (31). After the reaction, the developed solution changed from bright yellow to the brownish-orange chromogen. The changes of absorbance were noted spectrophotometrically (DU 640B Spectrophotometer, Beckman Coulter Inc., CA, USA) at 510 nm wavelength. A standard plot was prepared with 1 to 10 μmol solution of HCy. Standard HCy was prepared with 70 percentage of hydro-alcoholic preparation. The results were expressed as μmol per mg of protein.
Total protein level levels were estimated as described by Lowry et al. (38). Briefly, the change of absorbance was noted spectrophotometrically (DU 640B, UV-Spectrophotometer, Beckman Coulter Inc., CA, USA) at 750 nm wavelength. These readings were used for the further integrated calculation of total protein levels versus AChE, GSH, TBARS, MPO and HCy levels in tissue samples. The results were expressed as mg of protein per ml of supernatant.
All results were expressed as mean ± standard deviation (SD). Data obtained from behavioral tests were statistically analyzed using two-way analysis of variance (ANOVA) followed by Tukey’s test were applied by using Graph pad prism Version-5.0 software. The data of biomarkers changes.e., ChE activity, GSH, TBARS, MPO, and HCy levels were analyzed using one way ANOVA followed by Tukey’s multiple range tests which were applied for Post-hoc analysis by using Graph pad prism Version-5.0 software. A probability value of p < 0.05 was considered to be statistically significant.
The normal animal in acquisition trial days i.e., day 6 ELT value significantly (p < 0.05) decreases when compared to day 3 ELT value. It indicates that normal rats possess significant learning abilities (Figure 2A). In the retrieval test, the normal animal showed significant (p < 0.05) increase in the TSTQ (i.e., Q4 quadrant) value when compared to Q1 quadrant value (Figure 2B). The 4VO of rat brain produced a significant decrease in TSTQ (i.e., Q4) when compared to the sham group. It indicates an index of memory retrieval function. The treatment of ANF (40 and 80 mg/kg; p.o. for 5 consecutive days) showed a significant abolishing effect in 4VO induced reduction of TSTQ value dose-dependent manner. Hence, ANF produced the improvement of memory function in rats against the 4VO induced brain damage. Similarly, the treatment of reference compound i.e., donepezil (10 mg/kg; p.o. for 5 consecutive days) also produced the significant raising of TSTQ value. The results of ANF in ELT and TSTQ changes are illustrated in Figure 2 (A and B).
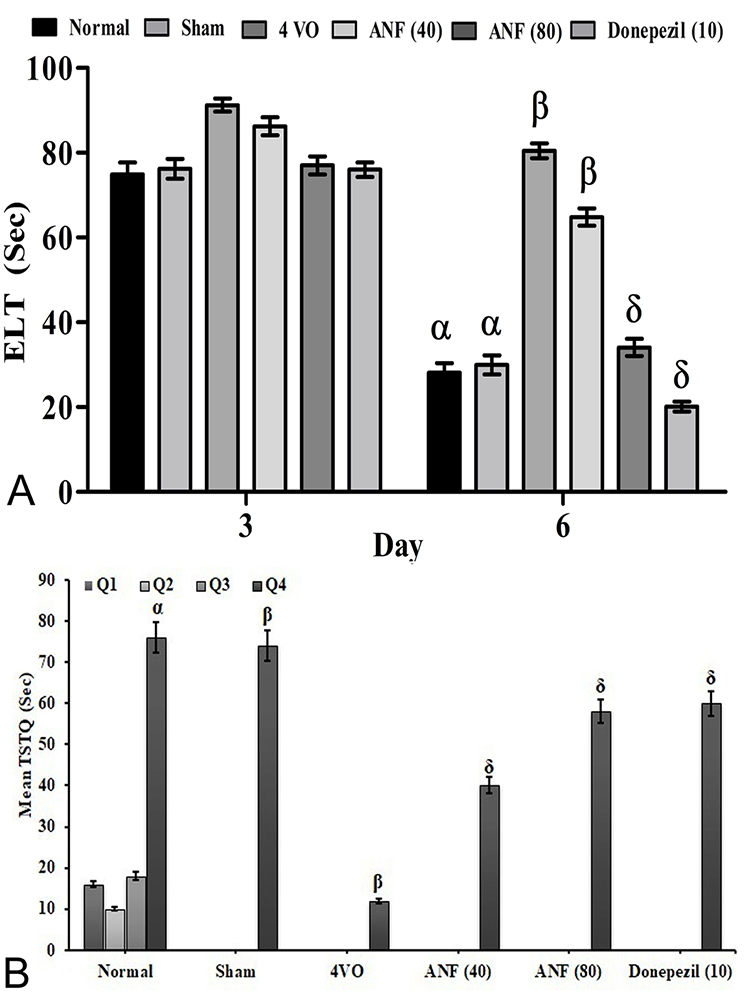
Effect of ANF on 4VO-induced changes of learning and memory process by MWM test. Digits in parenthesis indicate dose mg/kg. Data were expressed as mean ± SD, n = 8 rats per group. αp < 0.05 Vs Q1 normal group βp < 0.05 Vs Q4 normal group, δp < 0.05 Vs 4VO group. Abbreviation: ELT, escape latency time; TSTQ, time spent in target quadrant; 4VO, four vessel occlusion; and ANF, alpha-naphthoflavone.
The 4VO of rat brain produced significant (p < 0.05) increase in the transfer latency (TL) time when compared to the sham control group. The treatment of ANF (80 mg/kg; p.o.) significantly prevented the 4VO induced raising of TL duration indicating its memory enhancement effects in rat against 4VO induced neuronal damage. Whereas, treatment of ANF (40 mg/kg) did show any changes in the EPM test against 4VO in the rat. A similar effect was also observed in the reference compound i.e., donepezil (10 mg/kg; p.o.) treatment group. The results of ANF in changes are illustrated in Figure 3.
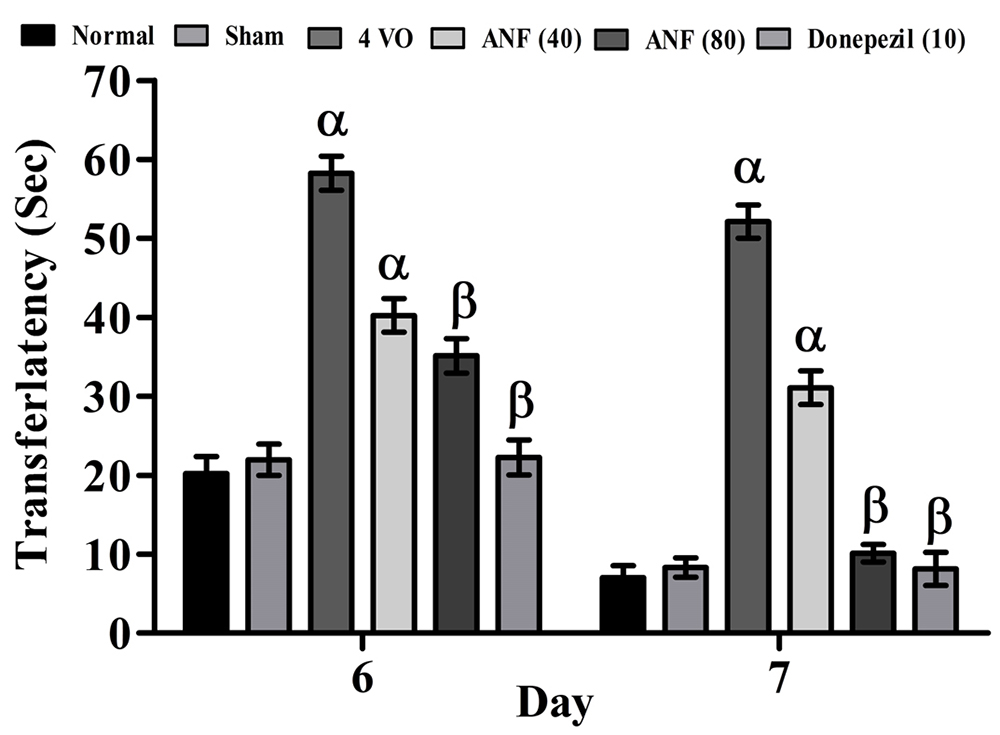
Effect of ANF on 4VO-induced changes of learning and memory process by EPM test. Digits in parenthesis indicate dose mg/kg. Data were expressed as mean ± SD, n = 8 rats per group. αp < 0.05 Vs normal group βp < 0.05 Vs 4VO group. Abbreviation: 4VO, four vessel occlusion; and ANF, alpha-naphthoflavone.
The 4VO of rat brain produced significant (p < 0.05) reduction of percentage grip strength against lateral push stimuli when compared to the sham control group. The treatment of ANF (80 mg/kg; p.o.) has shown significant prevention of 4VO induced changes of grip strength indicating that ANF (80 mg/kg) treatment possesses the improvement of grip strength ability with neuromuscular coordinated function in rats against 4VO induced neuronal damage. Whereas, treatment of ANF (40 mg/kg) did not show any changes in LPT against 4VO in the rat. A similar effect was also observed in reference compound i.e., donepezil (10 mg/kg; p.o.) treatment group (Figure 3). The results of ANF percentage grip strength changes are illustrated in Figure 4.
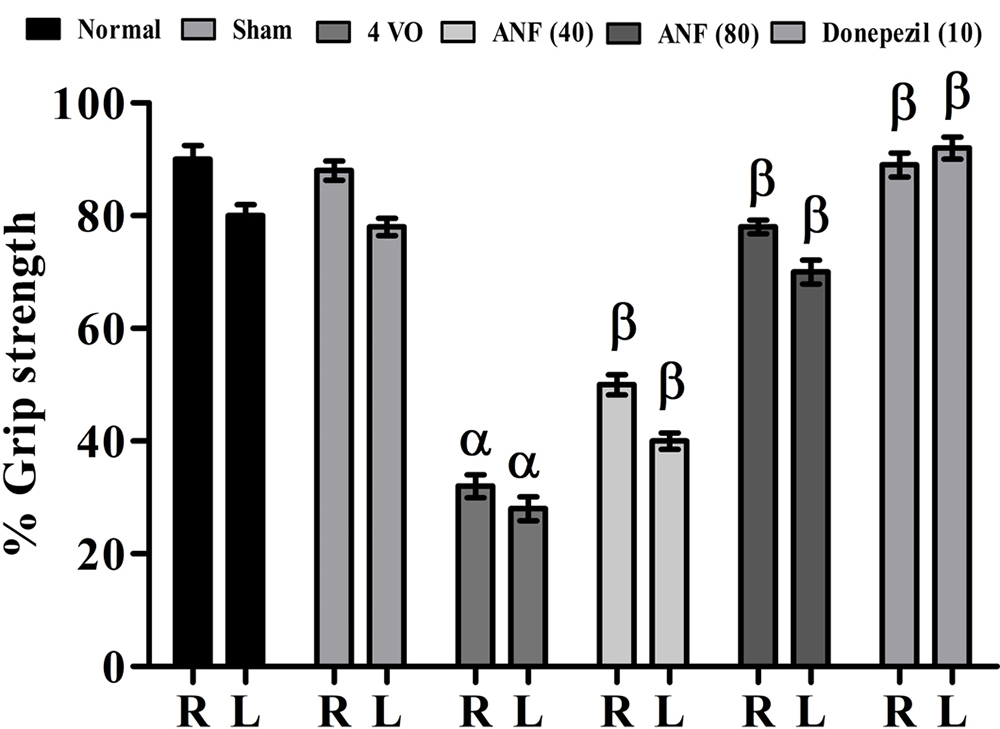
Effect of ANF on 4VO-induced changes of grip strength by LPT Digits in parenthesis indicates dose mg/kg. Data were expressed as mean ± SD, n = 8 rats per group. αp < 0.05 Vs normal group βp < 0.05 Vs 4VO group. Abbreviation: 4VO, four vessel occlusion; and ANF, alpha-naphthoflavone.
The 4VO of rat brain produced significant (p < 0.05) decrease the duration of interaction when compared to the sham control group. The treatment of ANF (80 mg/kg; p.o.) has shown significant prevention of 4VO induced changes in social interaction time. It indicates that ANF (80 mg/kg) treatment possesses the ability to improve psychosocial behavior ability within rats against 4VO induced neuronal damage. Whereas, treatment of ANF (40 mg/kg) did not show any changes in SIT against 4VO in the rat. A similar effect was also observed in reference compound i.e., donepezil (10 mg/kg; p.o.) treatment group. The results of the ANF duration of interaction changes are illustrated in Figure 5.
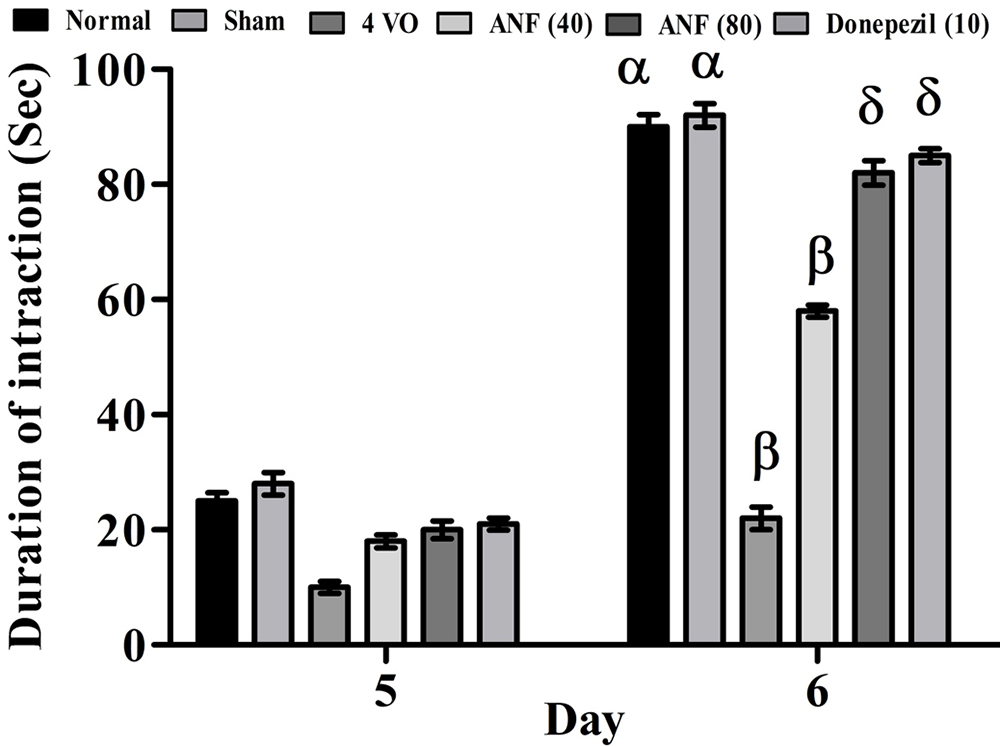
Effect of ANF on 4VO-induced changes of psychosocial behavior by SIT. Digits in parenthesis indicate dose mg/kg. Data were expressed as mean ± SD, n = 8 rats per group. αp < 0.05 Vs 5th day normal group; βp < 0.05 Vs normal group and δp < 0.05 Vs 4VO group. Abbreviation: 4VO, four vessel occlusion; and ANF, alpha-naphthoflavone.
The 4VO induced biochemical changes in rat plasma samples showed significant (p <0.05) rising HCy and nitrite levels when compared to the sham group. The treatment of ANF (40 and 80 mg/kg; p.o.) significantly prevented the 4VO-induced biochemical changes in a dose-dependent manner. It indicates that ANF regulates the accumulation of metabolic toxin and endothelial-derived vasorelaxing factors (EDRF: Nitric oxide) leads to production of neurovascular protection in the rat brain. Similar changes were also observed in reference compound i.e., donepezil (10 mg/kg; p.o.) treatment group against 4VO in rats. The results of ANF plasma biochemical changes were tabulated in Table 1.
Groups | HCy (μmol /L) | Nitrite (μM/L) |
---|---|---|
Normal | 16.4 ± 0.09 | 28.98 ± 0.21 |
Sham | 17.32 ± 2.12 | 30.12 ± 0.08 |
4 VO | 74.12 ± 0.29 α | 4.42 ± 0.49 α |
ANF (40) | 43.12± 0.34 β | 38.56 ± 2.10 β |
ANF (80) | 22.06 ± 0.05 β | 30.24 ± 0.26 β |
Donepezil (10) | 14.12 ± 2.52 β | 24.12 ± 0.88 β |
The 4VO induced biochemical changes in rat hippocampus samples showed significant (p < 0.05) rising AChE activity; TBARS; MPO and HCy along with a decrease in GSH levels when compared to the sham group. The treatment of ANF (40 and 80 mg/kg; p.o.) significantly prevented 4VO-induced biochemical changes in a dose-dependent manner. It indicates that ANF produces anti-oxidative, anti-lipid peroxidative, anti-inflammatory action and regulation of cholinergic neurotransmission as well as metabolic toxin actions. Similar changes were also observed in reference compound i.e., donepezil (10 mg/kg; p.o.) treatment group against 4VO in rats. The results of ANF hippocampus tissue biochemical changes were tabulated in Table 2.
The data of the present investigation has shown 4VO-produced potential neurovascular damage leads to significant changes in learning and memory functions along with the alteration of psychosocial and neuromotor functions. Furthermore, 4VO also induces bio-molecular changes in plasma and hippocampus tissue i.e., rising of AChE, TBARS, MPO and HCy levels, and a decrease in GSH level. The treatment of ANF (40 and 80mg/kg; p.o.) has shown significant (p < 0.05) preventive effects in 4VO-induced cerebrovascular injury, cognitive impairments and neuroinflammation in rats.
The reduction of cerebral blood flow indicated enhancement of neurovascular damage via activation of the free radical synthesis process. Hypoxic and hypoglycemic environments are known to induce abundant accumulation of free radicals via mitochondrial and non-mitochondrial mediated actions (39). Furthermore, free radicals are also interlinked with lipid peroxidative and calcium homeostasis actions in the biological system (40). The primary events of these biochemical changes subsequently alter mitochondrial dysfunction and raise endoplasmic reticulum (ER) stress (41). In mitochondria, ischemic environments raise free radical synthesis via multiple enzymatic reactions followed by releasing stored calcium ions in cytoplasmic regions (42). Later, mitochondria release the apoptotic proteins, damage the mitochondrial DNA and decrease mitochondrial intensity and dynamics leading to acceleration of neuronal death (43). ER also contributes to the development of oxidative stress in the neuronal system via alteration of pro-oxidative and anti-oxidative enzymes activation (44). In addition, nuclear DNA damage and membrane lipid peroxidation enhance neuronal death and neurodegeneration (45). Crucially, cellular and molecular changes are responsible for 4VO-associated cognitive dysfunctions and alteration of neuromotor and psychosocial behavior changes (46).
In addition, 4VO in the rat potentially induces cognitive impairment via damage of cortex and hippocampal neurons (47). In the present study, 4VO also showed cognitive dysfunction with alteration of learning and memory functions in MWM and EPM tests (48). It also alters in the in the muscle strength and psychosocial behavior in LPT and SIT assesments. The pathophysiological mechanism of 4VO-induced vascular dementia is due to neurovascular microenvironmental changes via alteration of nerve blood barrier changes (49), development of hypoxic (50) & hypoglycaemic (51) environments, accumulation of metabolic toxin i.e., homocysteine (52), raising of neuroimmune cell activations i.e., glial cells, astrocytes macrophage, and neutrophil infiltration (53), release of inflammatory cytokines like TNF-α, and raising apoptotic protein expression (54). Data from the present study reveal that 4VO of rat brain show neurocognitive and neurobehavioral changes. It also alters plasma and hippocampus tissue biomarkers. In addition, the ANF flavonoid is one of the natural medicines and it interacts with cellular enzymes and ion channels leading to the reduction of oxidative stress by reduction of lipid peroxidative products and the increasing endogenous reduced glutathione levels. Furthermore, it also controls the toxic metabolics, such as HCy associated neurovascular damage. Crucially, it produces the potential memory boosting effects via reduction of MPO and AChE levels. MPO is a marker of inflammatory reactions and AChE is the hallmark of cholinergic neurotransmitter-associated memory functions( 55). The treatment of ANF possesses multiple pharmacological actions such as free radical scavenging, anti-inflammatory; and regulatory role in metabolic toxin synthesis and cholinergic neurotransmissions. These results are comparable with reference compounds (donepezil) treatments. Various reports have revealed that AF has potential neuroprotective actions and can contribute to the enhancement of memory function (14). The application of 4VO leads cerebral hypo-perfusion of various regions of the brain leading to free radical generation and neuroinflammation. The treatment of ANF (40 and 80 mg/kg; p.o.) attenuates 4VO-associated cognitive impairments along with the reduction of free radicals and neuroinflammation. The conventional medicine i.e., donepezil also produces a similar therapeutic actions. Generally, nature-based medicines are more effective than synthetic molecules due to their multi-targeted actions.
Based on the data in hand and support from literature, it can be concluded that ANF (natural flavonoid) may be useful for the prevention of neurovascular disorders and cerebral hypo-perfusion-associated cognitive dysfunctions viz anti-oxidative, anti-lipid peroxidative, anti-inflammatory effects, lowering effects of neurotoxins and reduction of acetylcholinesterase activity levels.
The authors are thankful to the College of Pharmacy, JSS Academy of Higher Education and Research, Mysuru-570 015, Karnataka, India, and Pharmacology Unit, Faculty of Pharmacy, AIMST University, Semeling, 08100 Bedong, Kedah Darul Aman, Malaysia, for supporting this study and providing technical facilities for this work and we acknowledge the language and technical editing support provided by The Editing Refinery,MD, USA.