The objective of the present study was to investigate the effects of troxerutin (TX) on Nω-nitro-L-arginine methyl ester hydrochloride (L-NAME) induced hypertension in male albino Wistar rats. L-NAME (40mg/kg body weight (bw)) administration caused a sustained increase in systolic blood pressure (SBP), levels of thiobarbituric acid reactive substances (TBARS), lipid hydroperoxides (LOOH), tissue lipids (liver and kidney) such as total cholesterol (TC), triglycerides (TG), free fatty acids (FFA), and significant decrease in the activities of enzymatic antioxidants, phospholipids (PL) and levels of non enzymatic antioxidants such as reduced glutathione (GSH), vitamin C and vitamin E. To assess the toxicity if any of TX treatment, hepatic and renal function markers were measured. TX supplementation throughout the experimental period significantly brings back all the above parameters. Among the three doses (25, 50, and 100 mg/kg) of TX, 100 mg/kg dosage exerts optimum protection against L-Name induced hypertension. These results suggest that TX has enough potential to attenuate hypertension, oxidative stress and dyslipidemia in L-NAME induced hypertensive rats.
Hypertension is the most common cardiovascular disorder both in developed and in developing countries and has emerged as one of the major causes of mortality and morbidity worldwide.(1) Hypertension, frequently occurs in conjunction with metabolic disturbances and in particular with dyslipidemia.(2) Dyslipidemia is a broad term which implies imbalance between elevated circulating levels of cholesterol and it occurs concomitantly in over one-third of patients with hypertension.(3)
Nitric oxide (NO) has an important role in the physiologic control of blood pressure and myocardial injury. Alterations in NO synthesis or bioavailability cause vasoconstriction and might be involved in the pathogenesis of hypertension.(4,5) Abnormality in nitric oxide synthase (NOS) causes an alteration in the production of NO, which may contribute to the pathogenesis of hypertension and atherosclerosis.(6) Chronic inhibition of basal NO with an orally active NOS inhibitor (L-NAME), a particularly interesting model of hypertension. The chronic administration of L-NAME causes significant increase in blood pressure in experimental animals.(7,8)
Recent evidence indicates that oxidative stress, as the main mechanism is responsible for cardiovascular complications such as hypertension and alteration in lipid metabolism.(9,10) Oxidative stress, originally described as an altered balance between the production of free radicals and antioxidant defences.(11) The generated reactive oxygen species (ROS) induce lipid peroxidation, altered membrane structure and enzyme inactivation.(12)
Many modern drugs are effective in preventing cardiovascular disease (CVD), but their use is often limited because of their adverse effects. Therefore, the screening and development of drugs for CVD are still in progress. Plants have always been an exemplary source of drugs and many of the currently available drugs have been derived directly or indirectly from them.(13) Flavonoids are the most abundant polyphenolic compounds present in fruits, vegetables and plant-derived beverages such as tea and red wine.(14) Several epidemiological studies have reported an inverse correlation between flavonoid consumption and CVD risk.(15) Troxerutin (TX), a trihydroxyethylated derivative of the natural bioflavonoid rutin is present in tea, coffee, cereal grains and a variety of fruits and vegetables. TX possesses a variety of biological activities, such as vasoprotective, anti-oxidative and anti-inflammatory property.(16) Hence, the present study was undertaken to investigate the effect of TX on systolic blood pressure, oxidative stress, enzymatic and non-enzymatic antioxidants, lipid level, hepatic and renal function markers in L-NAME induced hypertensive rats.
Healthy male albino Wistar rats (180-220g), were obtained from the Central Animal House, Department of Experimental Medicine, Rajah Muthiah Medical College and Hospital, Annamalai University, India. They were housed (3 rats/cage) in polypropylene cages (47 × 34 × 20 cm) lined with husk, renewed every 24 h and maintained in an air-conditioned room (25 ± 3 °C) with a12 h light/12 h dark cycle. Feed and water were provided ad libitum to all the animals. The whole experiment was carried out according to the guidelines of the Committee for the Purpose of Control and Supervision of Experiments on Animals, New Delhi, India and approved by the Institutional Animal Ethics Committee of Rajah Muthiah Medical College and Hospital (Reg No. 160/1999/CPCSEA, Proposal number: 925), Annamalai University, Annamalainagar.
L-NAME and TX were purchased from Sigma-Aldrich (St. Louis, Missouri, USA). All other chemicals used in this study were of analytical grade obtained from Merck and Himedia, India.
Animals were given L-NAME in drinking water at a dosage of 40 mg/kg body weight (bw) for 4 weeks. TX was dissolved in water (vehicle) and administered at a dosage of 25, 50 and 100 mg/kg bw to rats orally everyday using an intragastric tube for a period of 4 weeks.
The animals were randomly divided into six groups of six animals each as given below.
Group I: Control+vehicle
Group II: Control+ troxerutin (100 mg/kg bw)
Group III: L-NAME control (40 mg/kg bw)
Group IV: L-NAME+ troxerutin (25 mg/kg bw)
Group V: L-NAME+ troxerutin (50 mg/kg bw)
Group VI: L-NAME+ troxerutin (100 mg/kg bw)
After the completion of experimental period, the rats were anaesthetized and killed by cervical dislocation. Blood was collected in a tube without anticoagulant. Serum was separated by centrifugation at 490 × g for 10 min.
Heart, aorta, liver and kidney tissues were sliced into pieces and homogenized in 0.1. M Tris-HCl buffer in cold condition (pH 7.4.) to give 20% homogenate (w/v). The homogenate was centrifuged at 560 × g for 10 min at 4 °C. The supernatant was separated and used for various biochemical estimations.
Before commencement of the experiment, animals were trained with the instrument for measuring blood pressure. In all groups of animals, systolic blood pressure (SBP) was measured every week during the entire period of the study noninvasively using a tail cuff method (IITC, model 31, USA) according to standard procedures. Values reported are the average of three readings. All the recordings and data analyses were done using a computerized data acquisition system and software.
The levels of thiobarbituric acid reactive substances (TBARS) and lipid hydroperoxides (LOOH) in tissues (Heart, aorta, liver and kidney) were estimated by the methods of Niehaus and Samuelsson(17) and Jiang et al.(18) respectively. The activities of enzymatic antioxidants such as superoxide dismutase (SOD), catalase (CAT) and glutathione peroxidase (GPx) in tissues were estimated by the methods of Kakkar et al.(19) Sinha(20) and Rotruck et al.(21) respectively. The levels of non-enzymatic antioxidants reduced glutathione (GSH), vitamin C and vitamin E in tissues were estimated by the methods of Ellman,(22) Roe and Kuether(23) and Baker et al.(24) respectively.
Tissue lipids were extracted by the method of Folch et al.(25) using chloroform: methanol mixture (2:1 v/v). The levels of total cholesterol (TC), triglycerides (TG), free fatty acids (FFA) and phospholipids (PL) in tissues (liver and kidney) were estimated by the methods of Zlatkis et al.(26) Fossati and Prencipe,(27) Falholt et al.(28) and Zilversmit and Davis(29) respectively.
The activities of serum aspartate aminotransferase (AST), alanine aminotransferase (ALT), alkaline phosphatase (ALP), and the levels of total proteins were estimated by using commercially available kits (Fisher Scientific, Kerala). The activity of gamma glutamyl transferase (GGT) was measured by the method of Rosalki and Rau.(30) The serum urea, uric acid and creatinine were estimated by using diagnostic kits based on the methods of Fawcett and Scott,(31) Caraway(32) and Jaffe(33) respectively.
Data were analyzed by one-way analysis of variance (ANOVA) followed by Duncan’s multiple range test (DMRT) using statistical package for the social science software version 20.0. Values are represented as mean ± S.D. for six rats in each group. Values were considered significant when P < 0.0.5.
Figure 1 shows the level of SBP measured by plethysmography in conscious rats. L-NAME treated rats showed increase in SBP (P<0.0.5) which was significantly attenuated by administration of TX (25, 50, and 100 mg/kg bw) throughout the study. Oral treatment of TX in control rats did not significantly modify SBP. Among the three doses 100 mg/kg bw improved the SBP to near-normal level. Hence, in the present study, all the evaluations were carried out with the optimum dosage 100 mg/kg.
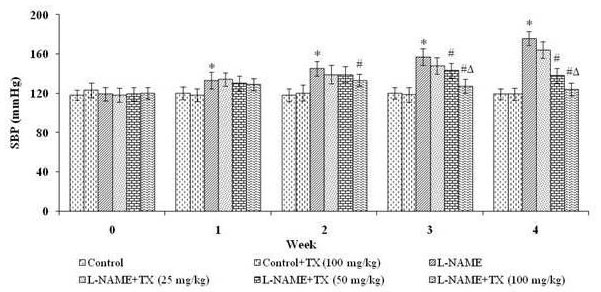
Effect of troxerutin on systolic blood pressure. L-NAME: Nω-nitro-L-arginine methyl ester hydrochloride. Values are represented as mean ± S.D. (n = 6). Values not sharing common symbol (*, # and Δ) are significant with each other at P < 0.0.5.
Table 1 depicts the effect of TX on the levels of TBARS and LOOH in the tissues (liver, kidney, heart and aorta) of control and L-NAME induced hypertensive rats. The L-NAME hypertensive rats exhibited significantly increased levels of TBARS and LOOH. Oral administration of TX at the dose of 100 mg/kg reduced the levels of lipid peroxidation products in L-NAME hypertensive rats.
Parameter | Sample | Control | Control+TX |
L-NAME | L-NAME+TX (100 mg/kg) |
---|---|---|---|---|---|
TBARS |
Heart | 0.53 ± 0.02a | 0.49 ± 0.01a | 2.38 ± 0.11b | 0.89 ± 0.03c |
Aorta | 0.42 ± 0.02a | 0.45 ± 0.03a | 1.72 ± 0.07b | 0.71 ± 0.02c | |
Liver | 0.73 ± 0.04a | 0.77 ± 0.05a | 1.9 ± 0.12b | 1.07 ± 0.06c | |
Kidney | 1.2 ± 0.06a | 1.24 ± 0.05a | 3.59 ± 0.16b | 1.94 ± 0.05c | |
LOOH |
Heart | 57.48 ± 3.85a | 54.59 ± 3.48a | 112.86 ± 8.74b | 73.64 ± 3.87c |
Aorta | 68.51 ± 5.02a | 66.34 ± 4.39a | 98.47 ± 7.36b | 79.33 ± 5.04c | |
Liver | 73.47 ± 4.29a | 71.68 ± 4.45a | 99.83 ± 5.42b | 86.69 ± 3.91c | |
Kidney | 59.31 ± 3.98a | 56.43 ± 3.11a | 135.74 ± 7.29b | 81.62 ± 4.11c | |
L-NAME: Nω-nitro-L-arginine methyl ester hydrochloride, Values are represented as mean ± S.D. (n = 6). Values not sharing common superscript (a, b and c) are significant with each other at P < 0.05 (Duncan’s multiple range test) |
The activities of SOD, CAT and GPx in tissues of control and L-NAME hypertensive rats are presented in Table 2. The activities of these enzymatic antioxidants were significantly (P<0.0.5) decreased in L-NAME hypertensive rats. Treatment with TX significantly (P<0.0.5) restored the activity of these enzymatic antioxidants in tissues. Table 3 illustrates the levels of non-enzymatic antioxidants such as vitamin C, vitamin E and glutathione in tissues of control and L-NAME hypertensive rats. The levels of non-enzymatic antioxidants were significantly decreased in L-NAME hypertensive rats. Oral administration of TX significantly improved these parameters towards normalcy.
Parameter | Sample | Control | Control+TX (100 mg/kg) | L-NAME | L-NAME+TX (100 mg/kg) |
---|---|---|---|---|---|
SOD (U*/mg protein) | Heart | 6.87 ± 0.24a | 6.74 ± 0.19a | 3.49 ± 0.22b | 5.19 ± 0.32c |
Aorta | 10.81 ± 0.76a | 11.14 ± 0.57a | 7.13 ± 0.31b | 9.45 ± 0.58c | |
Liver | 6.94 ± 0.34a | 7.12 ± 0.36a | 4.87 ± 0.22b | 6.13 ± 0.38c | |
Kidney | 12.8 ± 1.07a | 13.24 ± 1.19a | 7.85 ± 0.35b | 10.68 ± 0.7c | |
CAT (U#/mg protein) | Heart | 42.36 ± 3.39a | 46.18 ± 2.59a | 27.73 ± 2.58b | 36.27 ± 3.29c |
Aorta | 49.74 ± 3.11a | 52.13 ± 2.52a | 31.29 ± 2.38b | 41.76 ± 3.34c | |
Liver | 68.65 ± 3.87a | 69.71 ± 5.19a | 51.32 ± 3.07b | 61.85 ± 4.13c | |
Kidney | 29.85 ± 2.14a | 30.71 ± 1.56a | 16.42 ± 1.12b | 23.34 ± 1.26c | |
GPx (U$/mg protein) | Heart | 5.78 ± 0.31a | 5.85 ± 0.29a | 3.91 ± 0.26b | 4.95 ± 0.28c |
Aorta | 7.23 ± 0.38a | 7.18 ± 0.29a | 3.95 ± 0.27b | 5.64 ± 0.25c | |
Liver | 6.21 ± 0.34a | 6.53 ± 0.37a | 3.93 ± 0.28b | 5.54 ± 0.36c | |
Kidney | 7.09 ± 0.35a | 7.21 ± 0.43a | 4.1 ± 0.31b | 6.45 ± 0.34c | |
L-NAME: Nω-nitro-L-arginine methyl ester hydrochloride, Values are represented as mean ± S.D. (n = 6). Values not sharing common superscript (a, b and c) are significant with each other at P < 0.05 (Duncan’s multiple range test). U* - enzyme concentration required to inhibit the chromogen produced by 50% in one min under standard condition; U# - µM of H2O2 consumed/min; U$ - µg of GSH utilized/min |
Parameter | Sample | Control | Control+TX (100 mg/kg) | L-NAME | L-NAME+TX (100 mg/kg) |
---|---|---|---|---|---|
Vitamin C (µg/mg protein) | Heart | 0.44 ± 0.02a | 0.42 ± 0.03a | 0.21 ± 0.02b | 0.35 ± 0.02c |
Aorta | 0.45 ± 0.03a | 0.51 ± 0.04a | 0.28 ± 0.02b | 0.40 ± 0.03c | |
Liver | 0.70 ± 0.04a | 0.72 ± 0.06a | 0.43 ± 0.03b | 0.57 ± 0.04c | |
Kidney | 0.63 ± 0.04a | 0.66 ± 0.05a | 0.39 ± 0.02b | 0.52 ± 0.04c | |
Vitamin E (µg/mg protein) | Heart | 3.94 ± 0.13a | 4.05 ± 0.18a | 2.47 ± 0.07b | 3.42 ± 0.11c |
Aorta | 4.46 ± 0.28a | 4.51 ± 0.29a | 3.02 ± 0.09b | 4.05 ± 0.18c | |
Liver | 6.12 ± 0.21a | 6.19 ± 0.23a | 3.54 ± 0.15b | 5.21 ± 0.19c | |
Kidney | 4.25 ± 0.26a | 4.31 ± 0.27a | 2.12 ± 0.13b | 3.58 ± 0.17c | |
GSH (µg/mg protein) | Heart | 7.85 ± 0.37a | 7.67 ± 0.38a | 4.95 ± 0.27b | 6.68 ± 0.30c |
Aorta | 7.05 ± 0.21a | 7.11 ± 0.22a | 4.05 ± 0.19b | 5.81 ± 0.20c | |
Liver | 11.33 ± 0.62a | 12.32 ±1.07a | 6.31 ± 0.32b | 9.56 ± 1.06c | |
Kidney | 10.05 ± 0.41a | 11.13 ± 0.44a | 6.12 ± 0.25b | 8.13 ± 0.31c | |
L-NAME: Nω-nitro-L-arginine methyl ester hydrochloride, Values are represented as mean ± S.D. (n = 6). Values not sharing common superscript (a, b and c) are significant with each other at P < 0.05 (Duncan’s multiple range test) |
Table 4 portray the levels of lipids (TC, TG, FFA, and PL) in liver and kidney of control and L-NAME hypertensive rats. The increase in the levels of TC, TG and FFA and decrease in the level of PL were observed in L-NAME rats as compared to control rats. Treatment of TX significantly decreased the levels of TC, TG and FFA and increased the level of PL in L-NAME hypertensive rats.
Parameter | Sample | Control | Control+TX (100 mg/kg) | L-NAME | L-NAME+TX (100 mg/kg) |
---|---|---|---|---|---|
TC (mg/g wet tissue) | Liver | 4.41 ± 0.28a | 4.2 ± 0.24a | 7.85 ± 0.52b | 5.78 ± 0.21c |
Kidney | 3.67 ± 0.18a | 3.74 ± 0.22a | 6.85 ± 0.46b | 4.73 ± 0.26c | |
TG (mg/g wet tissue) | Liver | 4.12 ± 0.31a | 3.95 ± 0.25a | 7.34 ± 0.56b | 5.26 ± 0.23c |
Kidney | 4.85 ± 0.25a | 5.11 ± 0.23a | 6.75 ± 0.48b | 5.64 ± 0.27c | |
FFA (mg/g wet tissue) | Liver | 7.69 ± 0.32a | 7.85 ± 0.41a | 13.15 ± 0.83b | 9.61 ± 0.46c |
Kidney | 3.24 ± 0.19a | 3.56 ± 0.24a | 7.15 ± 0.42b | 4.86 ± 0.27c | |
PL (mg/g wet tissue) | Liver | 18.23 ± 0.72a | 17.78 ± 0.84a | 13.24 ± 0.65b | 16.45 ± 0.74c |
Kidney | 16.79 ± 0.73a | 16.51 ± 0.63a | 12.42 ± 0.71b | 15.12 ± 0.62c | |
L-NAME: Nω-nitro-L-arginine methyl ester hydrochloride; TC: Total cholesterol; TG: Triglycerides; FFA: Free fatty acids; PL: Phospholipids, Values are represented as mean ± S.D. (n = 6). Values not sharing common superscript (a, b and c) are significant with each other at P < 0.05 (Duncan’s multiple range test) |
Table 5 summarizes the effect of TX on the activities of hepatic marker enzymes such as AST, ALT, ALP and GGT, and levels of renal function markers such as urea, uric acid and creatinine in serum of control and L-NAME hypertensive rats. The activities of these pathophysiological marker enzymes were significantly (P<0.0.5) elevated in L-NAME hypertensive rats. Treatment with TX significantly (P<0.0.5) declined these levels towards normal.
Parameter | Control | Control+TX (100 mg/kg) | L-NAME | L-NAME+TX (100 mg/kg) |
---|---|---|---|---|
AST (IU@/l) | 69.36 ± 3.15a | 67.21 ± 3.11a | 97.45 ± 6.56b | 81.23 ± 3.76c |
ALT (IU@/l) | 32.25 ± 2.67a | 29.58 ± 2.23a | 59.35 ± 4.23b | 40.33 ± 3.21c |
ALP (IU*/l) | 70.21 ± 4.63a | 73.42 ± 4.16a | 118.23 ± 9.42b | 87.27 ± 5.53c |
GGT (IU$/l) | 19.34 ± 1.67a | 17.24 ± 1.45a | 30.56 ± 2.18b | 23.41 ± 2.15c |
Urea (mg/dl) | 25.34 ± 1.65a | 21.53 ± 1.78a | 40.18 ± 3.68b | 30.43 ± 2.11c |
Uric acid (mg/dl) | 1.78 ± 0.07a | 1.59 ± 0.06a | 3.06 ± 0.21b | 2.21 ± 0.07c |
Creatinine (mg/dl) | 0.88 ± 0.06a | 0.92 ± 0.05a | 2.57 ± 0.19b | 1.52 ± 0.06c |
L-NAME: Nω-nitro-L-arginine methyl ester hydrochloride, AST: Aspartate aminotransferase; ALT: Alanine aminotransferase; ALP: alkaline phosphatase; GGT: gamma glutamyl transferase, IU@ - μM of pyruvate liberated/h. IU* - μM of phenol liberated/min. IU$ - μM of p-nitroanilide liberated/min. Values are represented as mean ± S.D. (n = 6). Values not sharing common superscript (a, b and c) are significant with each other at P < 0.05 (Duncan’s multiple range test). |
Nitric oxide synthesis and release by endothelial cells play an important vascular relaxation effect contributing to the modulation of vascular tone.(34) A reduced NO production by vascular endothelial cells is closely associated with endothelial dysfunction or injury, which is proposed to be an important factor in cardiovascular diseases, especially in the development of atherosclerosis and hypertension.(35) Chronic blockade of NO synthesis by NOS inhibitors like L-NAME causes endothelial dysfunction, a significant increase in blood pressure and further pathological injuries to the cardiovascular system, which may lead to aggravation of hypertension.(36,37) In this study we demonstrated that chronic inhibition of NOS in L-NAME hypertensive rats significantly increased systolic blood pressure. Previous studies reported that the phenolic compounds reduce blood pressure and prevent target organ damage in hypertensive rats.(38) Treatment with TX significantly reduced the systolic blood pressure due to its antioxidant property.(16)
Oxidative stress, characterized by increased bioavailability of ROS such as superoxide anion, hydrogen peroxide and lipid peroxides, plays an important role in the development and progression of cardiovascular dysfunction associated with hypertensive disease.(39) Lipid peroxidation is an important pathogenic event in hypertension and accumulation of LOOH reflects the various stages of this disease and its complications.(40) The present study shows increased levels of lipid peroxidation products such as TBARS and LOOH as a consequence of oxidative stress-induced lipid membrane damage.(41) TX supplementation inhibited lipid peroxidation in L-NAME rats. This reveals the antilipid peroxidative role of TX that is probably mediated by its ability to scavenge free radicals.(16)
SOD plays an important role in scavenging superoxide anion, which is the initial free radical, among the oxygen radicals. Catalase prevents oxidative hazard by catalyzing the formation of water and oxygen from hydrogen peroxide.(42) GPx offers protection to the cellular and subcellular membranes from the peroxidative damage by eliminating hydrogen peroxide by utilizing reduced glutathione and H2O2 as substrates to yield H2O and oxidized glutathione and its declined activity may be due to the reduced availability of GSH.(43) TX supplementation significantly restored the activities of these enzymes in tissues of L-NAME rats.
The second line of defense consists of non-enzymatic antioxidants namely, vitamin C, vitamin E, and reduced glutathione which scavenge the residual free radicals escaping from decomposition by the antioxidant enzymes.(44) In the present study, the level of vitamin E significantly decreased in L-NAME hypertensive rats which may be due to the increased utilization of free radical scavenging reaction or could be due to the decreased vitamin C because there is a well-established synergism between vitamin C and E levels. Glutathione plays a marked role in detoxification reaction because it is a direct radical scavenger.(45) Our results depict that the levels of non-enzymatic antioxidants that were decreased in L-NAME-treated rats. Treatment with TX significantly elevated the levels of these non-enzymatic antioxidants.
Hypercholesterolemia and hypertriglyceridemia were seen in nitric oxide deficient rats which might be due to increased mobilization of lipids from liver and kidney tissues.(46,47) High levels of circulating cholesterol and its accumulation in tissues are well associated with cardiovascular damage.(48) Hypertriglyceridemia is independent risk factor that can accelerate the development of coronary artery disease and progression of atherosclerotic lesions.(49) In our study, we observed increased levels of TC and TG in liver and kidney of hypertensive rats. TX supplementation decreased the levels of TC and TG in hypertensive rats which may be due to the suppression of endogenous cholesterol biosynthesis by inhibiting the activity of HMG-CoA reductase.
PLs are vital components of biomembrane. These PLs and FFA are important for the maintenance of cellular integrity, microviscosity, and survival.(50) L-NAME rats showed decreased phospholipid content in tissues due to the oxidative damage of membrane by increased lipid peroxidation resulting in the release of phospholipids into blood.(51) Treatment with TX protects tissues from lipid peroxidation by quenching free radicals thereby increasing phospholipids level and decreasing the levels of FFA in tissues of L-NAME hypertensive rats.
Oxidative stress and alteration of cellular redox status are linked to many types of acute and chronic liver and kidney injury.(52) Leakage of hepatic enzymes from damaged cells leads to elevation of AST, ALT, ALP and GGT activities in the serum.(53) Kidney plays a central role in regulating the balance between body salts and water. Levels of renal markers such as urea, uric acid and creatinine were significantly increased in the serum of L-NAME hypertensive rats. Treatment with TX controlled the levels of hepatic and renal function markers with its antioxidant capacity.
In conclusion, present finding clearly portrays TX possesses antihypertensive effect in nitric oxide deficient hypertensive rats which is evidenced by a considerable decrease in the blood pressure, lipid peroxidation products, improved antioxidant status and altered lipid level. According to this study, TX does not have any adverse effects in 100 mg/kg dose. Our findings illustrate that; in future TX may be used as a beneficial molecule in the treatment of hypertension associated dyslipidemia.
Authors do not declare any conflict of interest. Indian Council of Medical Research (ICMR), New Delhi, India, funded this work.