- Academic Editor
Background: Diabetes mellitus (DM), a prevalent non-communicable
disease, is a metabolic condition involving defective pancreatic
Mortality rates in diabetes mellitus (DM) are consistently rising across the
globe, affected approximately 10% of the adult population worldwide [1]. DM is
characterized as a chronic disease resulting from insulin resistance, inadequate
insulin production or both. The three main categories of diabetes are: type 1,
type 2, and gestational diabetes [2]. Type 1 diabetes mellitus (T1DM) also known
as insulin-dependent diabetes, accounts for 10% of all diabetic patients and is
distinguished by the almost total loss of pancreatic
The initial steps in managing T2DM are a proper diet, weight management, as well
as regular physical activity [7]. In addition to these, various medication
categories, including metformin, sulphonylureas, thiazolidinediones,
Glucagon-like peptide 1 (GLP-1) analogues, Glucose-dependent Insulinotropic
Peptide (GIP) and GLP-1R co-agonists, dipeptidyl peptidase-IV (DPP-IV)
inhibitors, sodium-glucose co-transporter 2 (SGLT2) inhibitors and synthetic
insulin, are now used as treatments for T2DM [8]. However, these synthetic
medications come with a host of negative side effects, such as gastrointestinal
abnormalities, obesity, hepatic and renal disorders, low blood sugar level, and
are often expensive and unavailable to rural and economically deprived areas
[4, 9, 10]. As a result, researchers are devoting a great deal of time and energy
into studying plants and other natural sources in an effort to discover new
treatments to combat diabetes mellitus [11]. Since ancient times, medicinal
plants, such as Gynura nepalensis DC., Glochidion thomsonii
(Müll.Arg.) Hook.f., Clerodendrum splendens G.Don,
Clerodendrum infortunatum L. and Xanthium strumarium L. have
been employed in local communities to treat illnesses, such as diabetes,
hypertension, liver disease, lung problems, microbial infections, cancer,
inflammation, and gastrointestinal problems, due to their numerous health
benefits [12, 13, 14, 15, 16]. These plants contain a variety of potent bioactive
phytoconstituents, including rutin, quercetin, linolenic acid, epicatechin,
gymnemic acid, kaempferol, berberine, gallic acid and iminosugars which is
particularly present in Casuarina equisetifolia L., are capable of
reducing high blood sugar levels by increasing insulin secretion, inhibiting
glycosidase and glycosyl transferase activity as well as protecting pancreatic
The aim of the current study was to evaluate glucose lowering properties of five traditional medicinal plants (X. strumarium, C. infortunatum, C. splendens, G. thomsonii and G. nepalensis) including the effects on food intake and gut motility in vivo.
The five plants, X. strumarium (leaves), C. infortunatum (roots), C. splendens (leaves), G. thomsonii (bark) and G. nepalensis (leaves) were obtained from Jahangirnagar University, Dhaka, Bangladesh, and a plant taxonomist from Bangladesh National Herbarium identified and allocated their individual accession numbers DACB87271, DACB87272, DACB87273, DACB87274 and DACB87275 respectively. Following rinsing and air drying of the plant parts, 200 g of the dried plant powder was added to 1 liter of 80 % (v/v) ethanol and shaken at a speed of 900 g for 48 to 72 h at room temperature. The mixture was separated with filter paper (Whatman no. 1), and a rotary evaporator (BibbyRE-200, Sterilin Ltd., Newport, UK) was then used to dehydrate the filtered extract. The final product was lyophilized in a freeze dryer vacuum (Savant Speed vac, New York, NY, USA), and then preserved at 4 °C for further experiments [23].
Six to eight weeks old Long Evan male rats (200–250 g) were fed a high-fat diet (20% of protein, 45% of fat, and 35% of carbohydrate: 26.15 KJ/g total energy percent) for 6 to 8 weeks before the start of the studies. For normal control, same aged rats received a standard rodent diet (30% protein, 60% carbohydrate, and 10% fat, making 12.99 KJ/g total energy, Trouw Nutrition, Cheshire, UK) were used. Before conducting the experiments, fasting blood glucose were measured in high fat fed (HFF) diet rats to separate in individual group. Higher than normal fasting blood glucose levels (5.6 to 7.0 mmol/L) were considered as HFF diet-induced obese rats. The fasting blood glucose of 10 rats were within the normal range which had been excluded from the studies. The groups were divided as follows:
Group 1: Lean control (saline)
Group 2: HFF diet control (saline)
Group 3: HFF diet control + G. nepalensis (250 mg/kg)
Group 4: HFF diet control + G. thomsonii (250 mg/kg)
Group 5: HFF diet control + C. infortunatum (250 mg/kg)
Group 6: HFF diet control + X. strumarium (250 mg/kg)
Group 7: HFF diet control + C. splendens (250 mg/kg)
Group 8: HFF diet control + Glibenclamide (5 mg/kg)
Rats given a high-fat diet were starved for 12 h and administered glucose (18 mmol/kg, body weight (b.w.)) alone (control) or in conjunction with ethanolic extracts (250 mg/kg, b.w.) orally. Blood samples were drawn from the tail vein prior to (0 min) and after (30, 60, 120, and 180 min) treatments. The plasma was separated from the blood by centrifugation at 12,000 rpm for 5 min at 4 °C and the samples were stored at –20 °C for the plasma insulin measurement using Rat Insulin ELISA Kit (Crystal Chem, Elk Grove Village, IL, USA). Ascencia Contour glucose meters (Bayer, Newbury, UK) were used to measure blood glucose levels [24]. Glibenclamide, a sulfonylurea drug, used as a positive control, was dissolved in Dimethyl sulfoxide (0.6% DMSO).
HFF rats were used to study the impact of extracts on food consumption. Before the experiment, the rats were fasted for 12 h. Oral administration of saline (5 mL/kg, b.w.), extracts (250 mg/kg, b.w.), or glibenclamide (5 mg/kg, b.w.) was followed by measurement of food intake at 0, 30, 60, 90, 120, and 180 min respectively. Glibenclamide, a standard drug, used as the positive control [25].
Metabolic studies were performed in HFF Long Evan male rats using metabolic cages to measure food and fluid consumption. The rats underwent 12-h fasting after a 24-h adaptation period. HFF diet control group received saline (5 mL/kg, b.w.), and the treatment groups received plant extracts (250 and 500 mg/kg, b.w.). The food and fluid intake were observed and recorded every 1 h for the first 6 h, then every 2 h for the next 6 h and finally at 24 h [25].
BaSO4 milk solution was used to assess the gastrointestinal motility. Rats were starved for 20 h and 1 h before administering 10% BaSO4 (W/V of 0.5% Na-CMC) mixture, the treatment groups received extracts (250 mg/kg), bisacodyl (10 mg/kg) and loperamide (5 mg/kg) respectively. After 15 minutes of consuming the BaSO4 milk solution, the animals were killed, and their entire intestines were removed. The distance of BaSO4 travelled was measured and expressed as a fraction of its full length (from the pylorus to ileocecal junction) [25].
Secondary metabolites such as alkaloids, saponins, steroids, flavonoids, tannins, reducing sugar, and glycosides were identified using previously described techniques [26]. Alkaloids were tested by acidifying 2 mL of the extracts with hydrochloric acid (HCl), to which 1 mL Dragendroff’s reagent was added, and the appearance of a red color showed the presence of alkaloids. To test for tannins, a few drops of 10% lead acetate were added to 2 mL of the extracts and the formation of white sediment confirmed the presence of tannins. Testing for the presence of flavonoids involved heating a mixture of 4 mL of the extracts and 1.5 mL methanol; the appearance of a pink color upon the addition of magnesium and a few drops of HCl suggested the presence of flavonoids. To test for saponins, 1 mL of the extracts was mixed with 9 mL distilled water which produced a stable foam suggesting the presence of saponins. To check the presence of steroids, 2 mL of the extracts were mixed with 10 mL chloroform, 1 mL acetic anhydride, and 2 mL sulphuric acid; the formation of a bluish-green color indicated the presence of steroids. Glycosides were tested by combining 1 mL of the extracts with a few drops of glacial acetic acid, ferric chloride, and concentrated sulphuric acid; visualization of bluish-green color suggested the presence of glycosides. Reducing sugars were tested by combining 1 mL of the extracts, 1 mL of distilled water, and a few drops of Fehling’s reagent. The mixture was heated, and visualization of a red-brick color indicated the presence of reducing sugars.
To analyse and interpret the data, Graph Pad prism 5 (San Diego, CA, USA) was
used. Data analysis was done using an unpaired Student’s t-test
(nonparametric, with two-tailed p values) and a one-way ANOVA with
Bonferroni post hoc testing, and the values were represented as Mean
Oral administration of G. nepalensis, G. thomsonii, C. infortunatum
and C. splendens (250 mg/kg), when given in combination with glucose
(18 mmoL/kg body weight) significantly ameliorated glucose tolerance in HFF rats
at 30 and 60 min (p
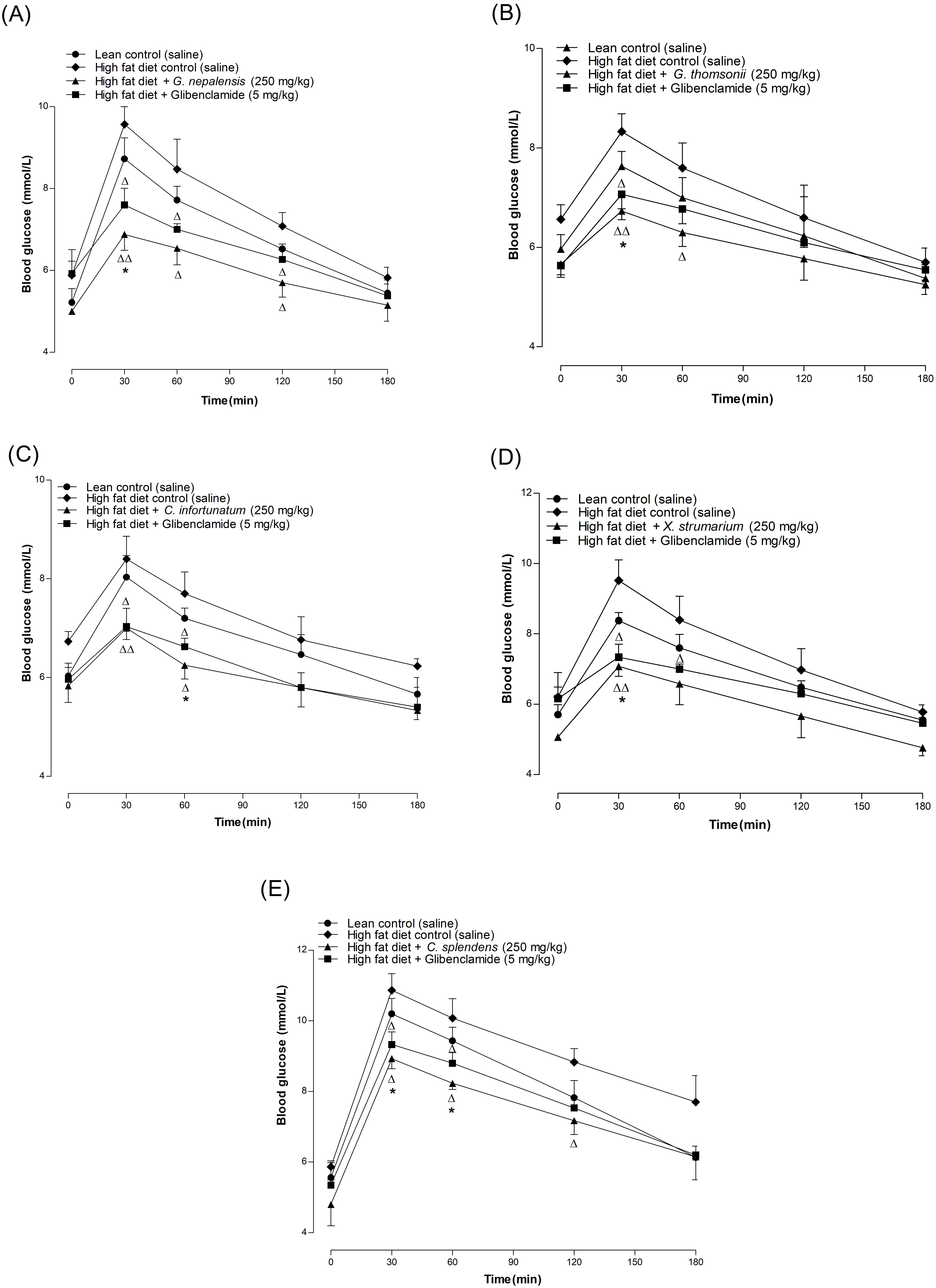
Effects of ethanol extracts of (A) G. nepalensis, (B)
G. thomsonii, (C) C. infortunatum, (D) X. strumarium
and (E) C. splendens on oral glucose tolerance in HFF rats. Blood
glucose was monitored in overnight fasted rats before and after oral gavage of
glucose (2.5 gm/kg, body weight, control), with or without a plant extracts (250
mg/kg, body weight) or glibenclamide (5 mg/kg). Values n = 6 are mean
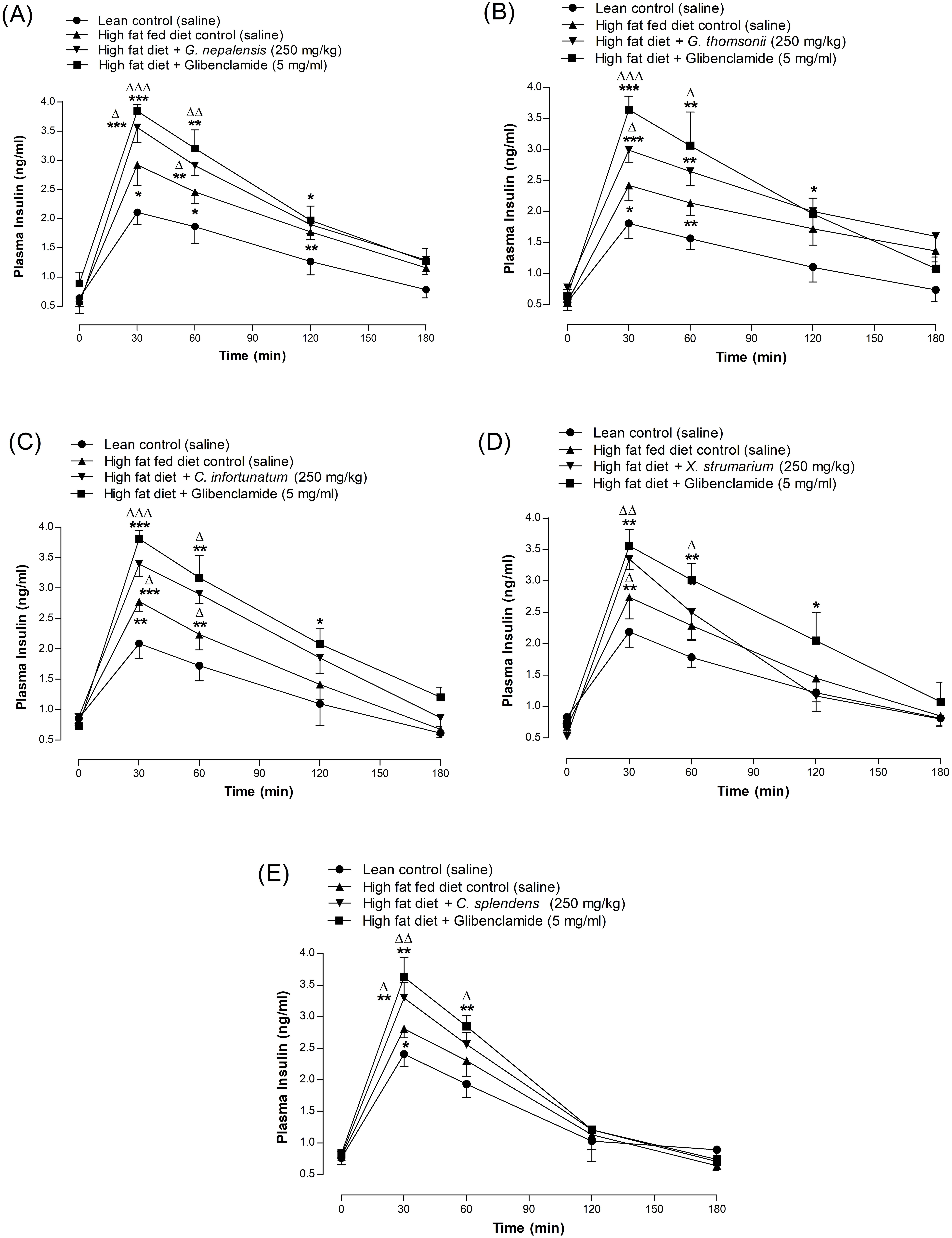
Effects of ethanol extracts of (A) G. nepalensis, (B)
G. thomsonii, (C) C. infortunatum, (D) X. strumarium
and (E) C. splendens on plasma insulin levels in HFF rats.
Plasma insulin was monitored in overnight fasted rats before and after oral
gavage of glucose (2.5 gm/5 mL/kg, body weight, control), with or without a plant
extracts (250 mg/kg, body weight) or glibenclamide (5 mg/kg). Values n = 6 are
mean
G. nepalensis (250 mg/kg), caused a significant decrease in food intake
at 120 and 180 min (p
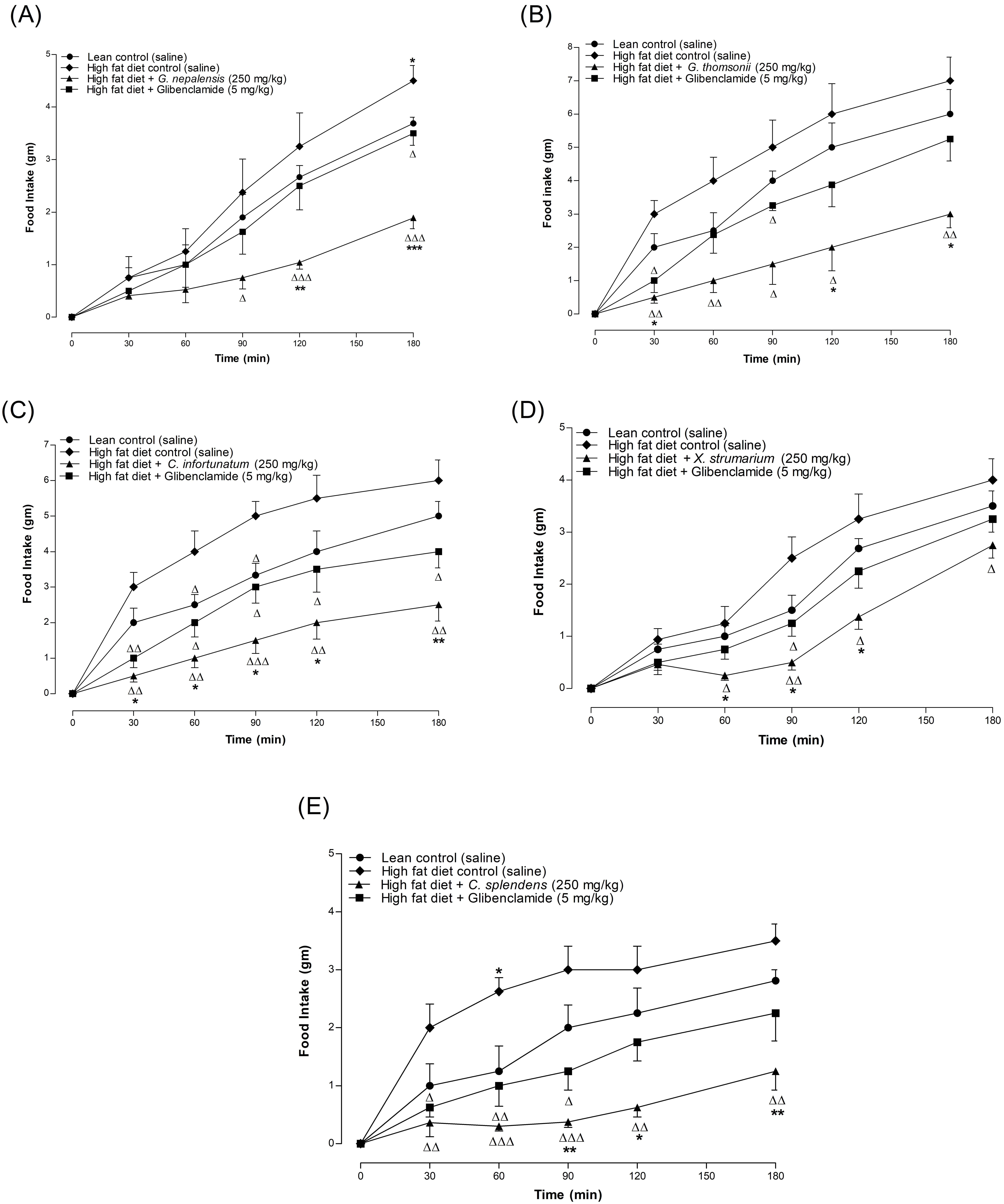
Effects of ethanol extracts of (A) G. nepalensis, (B)
G. thomsonii, (C) C. infortunatum, (D) X. strumarium
and (E) C. splendens on food intake in HFF rats. Food intake was
assessed in 12 h fasted rats with or without oral administration of plant extracts
(250 mg/kg, body weight) or glibenclamide (5 mg/kg). Values n = 6 are mean
Although ethanol extracts of G. nepalensis, G. thomsonii, C. infortunatum, X. strumarium and C. splendens (250 and 500 mg/kg, b.w.) decreased food and fluid intake in HFF rats, over the period of 36 h, was not significant in comparison to the control (Figs. 4A–E,5A–E). However, extracts, at 500 mg/kg, b.w. was more effective than at 250 mg/kg, b.w. (Figs. 4A–E,5A–E).
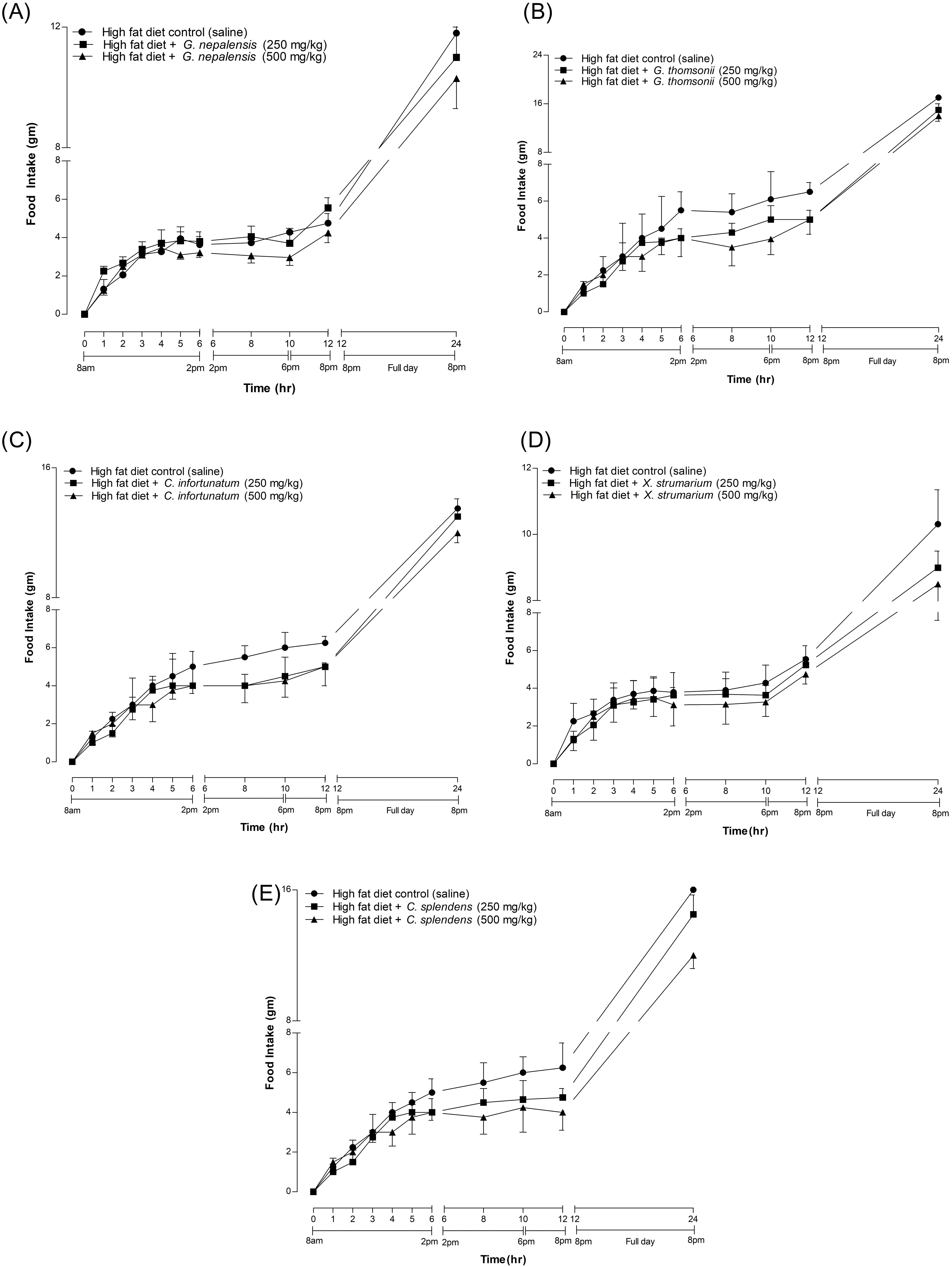
Effects of ethanol extracts of (A) G. nepalensis, (B)
G. thomsonii, (C) C. infortunatum, (D) X. strumarium
and (E) C. splendens on food intake after 36 h of metabolic study. Food
intake was measured at 1, 2, 3, 4, 5, 6, 8, 10, 12 & 24 h interval under metabolic cage
along with or without either receiving a plant extracts (250 & 500 mg/kg, body
weight). Values n = 6 are mean
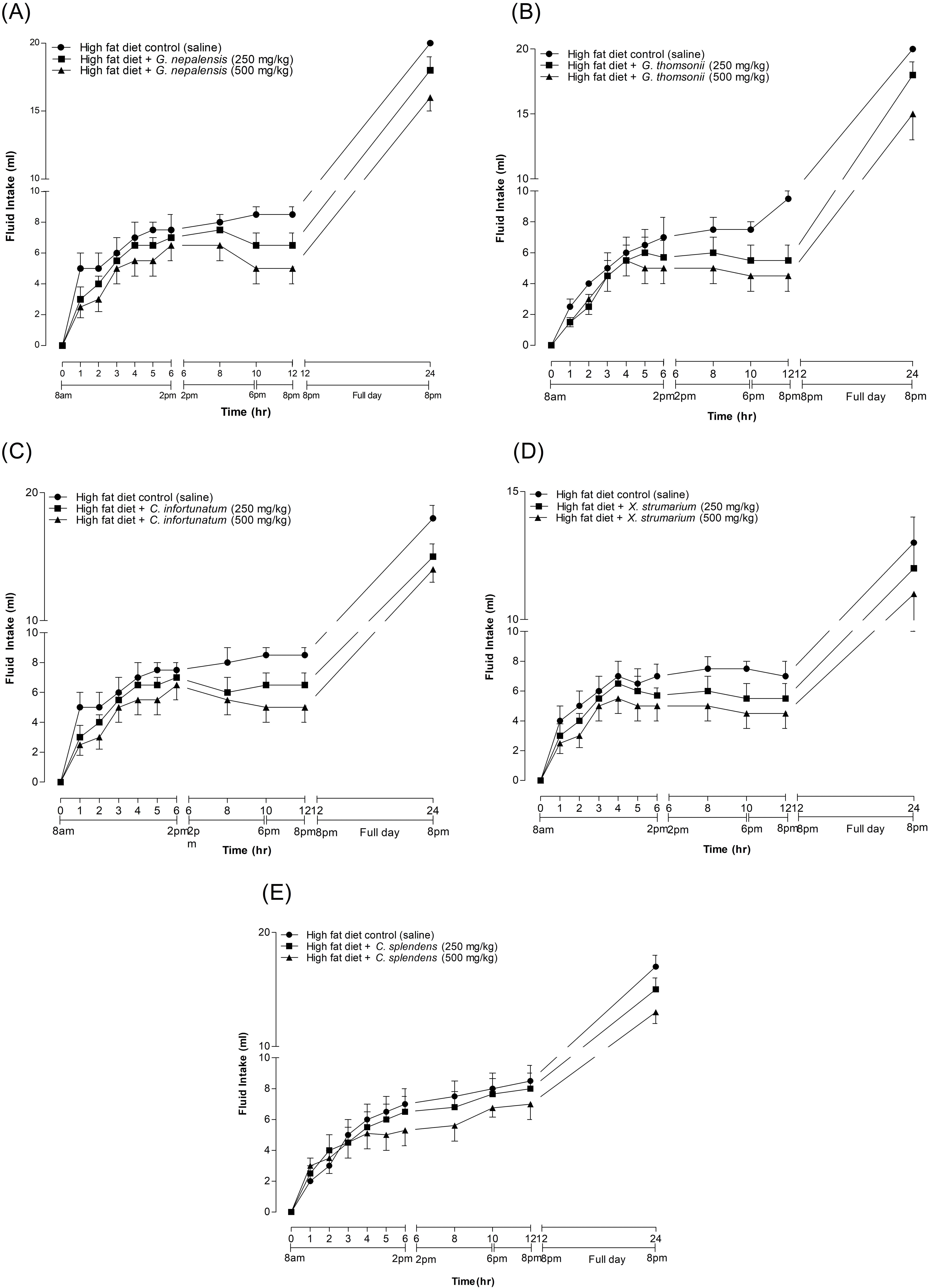
Effects of ethanol extracts of (A) G. nepalensis, (B)
G. thomsonii, (C) C. infortunatum, (D) X. strumarium
and (E) C. splendens on fluid intake after 36 h of metabolic study.
Fluid consumption was assessed at 1, 2, 3, 4, 5, 6, 8, 10, 12 and 24 h intervals using
metabolic cage along with or without a plant extracts (250 & 500 mg/kg, body
weight). Values n = 6 are mean
The ethanol extract of plants, G. nepalensis, G. thomsonii, C.
infortunatum, X. strumarium and C. splendens (250 mg/kg) markedly
enhanced gut motility (p
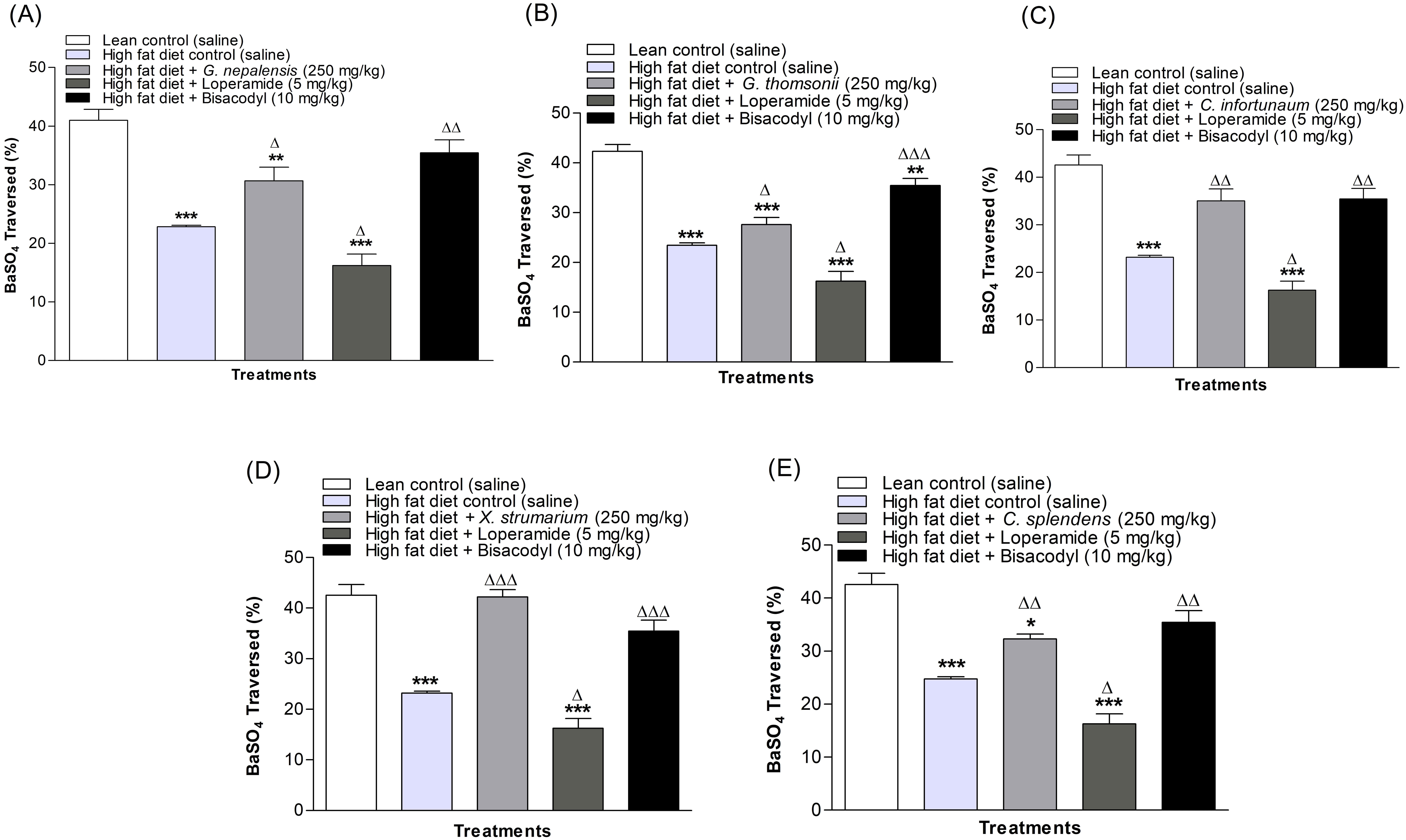
Effects of ethanol extracts of (A) G. nepalensis, (B)
G. thomsonii, (C) C. infortunatum, (D) X. strumarium
and (E) C. splendens on gastrointestinal motility. BaSO4 milk solution
was used to evaluate gastrointestinal motility by measuring BaSO4 travel length
in 20 h fasted rats after oral gavage of BaSO4 solution with or without a plant
extracts (250 mg/kg, body weight), loperamide (5 mg/kg) or bisacodyl (10 mg/kg).
Values n = 6 are mean
Saponins and flavonoids were detected in all five plants in phytochemical screening of crude extract, as well as tannins in G. nepalensis, G. thomsonii, C. splendens, and X. strumarium (Table 1). Reducing sugar was found in G. nepalensis and G. thomsonii and alkaloids were only present in G. thomsonii (Table 1). Additionally, G. nepalensis also contained steroids (Table 1).
Alkaloids | Tannins | Saponins | Steroids | Glycoside | Flavonoids | Reducing Sugar | |
---|---|---|---|---|---|---|---|
Gynura nepalensis | – | + | + | + | – | + | + |
Glochidion thomsonii | + | + | + | – | – | + | + |
Clerodendrum infortunatum | – | – | + | – | – | + | – |
Xanthium strumarium | – | + | + | – | – | + | – |
Clerodendrum splendens | – | + | + | – | – | + | – |
(+) = present, (–) = absent.
Diabetes, one of the most prominent and severe metabolic disorders, has greatly
affected many individuals all over the world [27]. Obesity, commonly
characterized to be an excess of body fat, is a major risk factor for T2DM. In
people who are obese, adipose tissues produce nonesterified fatty acids (NEFAs),
which may result in insulin resistance and
In acute in vivo studies, the ethanol extracts of G.
nepalensis, G. thomsonii, C. splendens, C. infortunatum and X.
strumarium were found to substantially ameliorate glucose tolerance and plasma
insulin level in HFF rats, suggesting that all five plants have glucose lowering
properties. Recent reports have demonstrated that C. infortunatum
improves glucose tolerance and reduces fasting blood glucose in streptozotocin
induced diabetic rats [30]. Previous research on G. nepalensis and
X. strumarium showed that they possess
Additional in vivo studies on HFF rats comprised feeding tests and metabolic studies to investigate the effect of plant extracts on food and fluid consumption. The plant extracts reduced both fluid and food consumption but was not significant as compared to HFF control. Plant extracts with high dose (500 mg/kg, b.w.) shown more efficacy in decreasing these parameters, particularly at night (between 6 to 8 PM). Rats are known to be more active during night-time and the blood sugar levels in diabetic rats tend to be at peak at night [36]. Thus, reduction in food intake caused by medicinal plant extracts may be responsible for maintaining healthy blood glucose levels.
Gastrointestinal motility was observed using BaSO4 milk solution. The present studies showed that plant extracts promote gut motility, indicating that the plants may shorten the time available for the digestion and absorption of carbohydrates in the gut, leading to decreased glucose absorption and plasma sugar levels [37]. The presence of saponins in these plants may contribute to improve gut motility, as saponins are known to inhibit disaccharidase activity and intestinal glucose absorption in streptozotocin induced rats [38].
Phytochemical screening of the five plant extracts confirmed the presence of
flavonoids and saponins, which is consistent with previous studies on these
plants [39, 40, 41, 42, 43]. Flavonoids, such as kaempferol, rutin and quercetin, have
previously been demonstrated to have glucose lowering, insulin secreting, and
Traditional medicinal plants, G. nepalensis, G. thomsonii, C. splendens, C. infortunatum and X. strumarium, showed improvement in glucose tolerance, plasma insulin level and gastrointestinal motility in HFF rats, suggesting that the extracts may improve diabetes and its complexities by lowering blood sugar levels and inhibiting carbohydrate digestion and absorption in the gut. In addition, the plants were also observed to reduce consumption of food and fluid in HFF rats. The anti-hyperglycaemic properties of these plants may be attributed to the presence of phytochemicals such as flavonoids and saponins. Our findings support the use of these plants as an ethnomedicine for the treatment of T2DM. Additional in-depth research, such as the purification and identification of active components from these plants, could aid in the development of anti-diabetic therapy for T2DM in humans.
The information is not available to the public as a result of certain limitations. The corresponding author, however, is willing to provide the information acquired from this study upon request.
JMAH and PA were equally responsible for the conception and design of the study as well as the supervision of the study; PA, NN, FTT and AT conducted the experiments, analyzed the data, evaluated the results, created the figures, and PA drafted the paper, while PA and JMAH edited the revised manuscript. All authors have participated sufficiently in the work to take public responsibility for appropriate portions of the content and agreed to be accountable for all aspects of the work in ensuring that questions related to its accuracy or integrity. The final manuscript has been read and approved by all authors.
Independent University, Bangladesh (IUB), Institutional Review Board (IRB) approved protocols on 19th December 2019 for experiments to be performed using animals and the experiments were performed in line with the Animal Welfare Act 2019 of Bangladesh. It was ensured that no animals will be injured over the course of this study.
The authors would like to express their appreciation to the Independent University, Bangladesh (IUB), Dhaka 1229, Bangladesh for providing the facilities to conduct the studies.
This study was supported by IUB sponsored research project fund (2019-SESM-09) from Independent University, Bangladesh (IUB), Dhaka 1229, Bangladesh.
The authors declare that there is no conflict of interest.
Publisher’s Note: IMR Press stays neutral with regard to jurisdictional claims in published maps and institutional affiliations.