- Academic Editor
Background: Hydroxychloroquine (HCQ) toxicity can adversely affect vital organs, cause pathologic ocular damage, and can have direct cardiovascular effects. This study aims to identify the biochemical, hematological, and histological alterations of the vital organs associated with the effects of HCQ. Methods: Male albino rats were exposed to the equivalent of HCQ therapeutic doses given to human patients being affected by malaria, lupus erythematosus, and COVID-19. The animal blood samples were subjected to hematological analysis, biochemical analysis, liver function tests, kidney function tests, and cardiac biomarkers. Liver, kidney, heart, spleen, and testis biopsies were subjected to histological examination. Results: HCQ significantly lowered the values of erythrocytes, hemoglobin, hematocrit, platelets, leucocytes, and lymphocytes but significantly increased the values of aspartate aminotransferase (AST), alanine aminotransferase (ALT), amylase, alkaline phosphatase, lactate dehydrogenase, cholesterol, and chlorine ions. The renal tissues of HCQ-treated animals demonstrated glomerular fragmentation, partial atrophy degeneration, renal tubules hydropic degeneration, hyaline cast formation, and interstitial edema formation. Additionally, the heart exhibited myofiber necrosis, myolysis, wavy appearance, disorganization, and disarray. The testicular tissues also demonstrated spermatocyte degeneration, spermatogenic cell sloughing, testicular interstitial edema, and occasional spermatogenic arrest. Additionally, the spleen showed a decrease in the number and size of the white pulp follicles, a decrease in the number of apoptotic activity, and a decline in the number of T-rich cells. However, the red pulp demonstrated a diffuse decline in B rich-lymphocytes and macrophages. The liver was also the least affected but showed Kupffer cell hyperplasia and occasional hepatocyte dysplasia. Conclusions: The results indicate that chronic exposure to HCQ could alter the structures and functions of the vital organs.
Hydroxychloroquine (HCQ) is an anti-inflammatory and anti-apoptotic agent widely used to treat malaria and autoimmune diseases, such as systemic lupus erythematosus, rheumatoid arthritis, endometriosis, and porphyria cutanae tarda [1, 2, 3, 4]. HCQ is also a novel pharmaceutical therapy for managing type-2 diabetes, lowering cardiovascular risk, guarding against myocardial injury, attenuating renal ischemia, and inhibiting cathepsin inflammasome activation [5, 6]. The outcomes of some medical investigations showed that HCQ could improve lipotoxicity related-obesity and reduce insulin resistance through the proliferation of the peroxisome gamma pathway activation receptor [7]. During the height of the COVID-19 pandemic, global medical professionals have not invested in HCQ drugs for COVID-19 treatment because of the major concerns about its potential side effects [8, 9, 10]. Some studies indicated that this drug had impacts on the cellular receptor of SARS-CoV-2 entry in the pulmonary cells [11].
HCQ is absorbed after oral administration through the alimentary canal, distributed to various organs, and eliminated through urine [12]. This drug can easily cross the plasma membrane and accumulate in the cytoplasm; its free base also accumulates in the lysosomes, and an increase in its PH inhibits organelles. Studies have shown that 3A4, 3A5, CYP2D6, and 2C8 cytochrome genes metabolize this HCQ drug to N-desethylhydroxychloroquine derivative with possible interference with the immune system [13]. The clinical reports also revealed that HCQ could cause retinopathy, cardiotoxicity, bone-marrow toxicity, alimentary canal disturbances, hypoglycemia, itching, acne, hemolytic anemia, loss of hair, and urinary incontinence [14]. Some studies have indicated that HCQ could induce sciatic nerve oxidative stress and muscle tissues in correlation with sciatic nerve axonal atrophy and HCQ neuromyo-toxicity-induced myopathy, dysphagia, polyradiculoneuropathy, and neuropathy [15, 16, 17].
With the outbreak of the COVID-19 pandemic, a significant investment has been made in HCQ for COVID-19 treatment. However, the international medical circles failed to provide significant evidence to back up the use of HCQ for COVID-19 therapy. In March 2020, USA Food and Drug Administration (FDA) permitted the distribution of HCQ to public health authorities for hospitalized patients with COVID-19 [18]. However, HCQ is available for treating malaria and several autoimmune diseases in some African countries and the Middle East [19, 20].
The toxicities associated with HCQ mechanisms are unknown. The clinical symptoms in patients who experience an overdose or accidental poisoning of HCQ are unknown. Moreover, little is known about the chronic alterations in gene expression, oxidative stress, psychiatric behavior, interaction with macromolecules, and ultrastructural injury in the vital organs, based on chronic exposure to HCQ. This study investigates the hematological, biochemical, and histological changes, resulting from chronic subjection to HCQ therapeutic doses.
Hydroxychloroquine sulfate (99.90% purity) was obtained from Hikma Pharmaceutical Company in Jordan.
Male albino rats (Rattus norvegicus), weighing 210–225 gm, obtained from the breeding of Jerash University, were used for the experiment. The rats were grouped into four (8 rats each).
The animals were housed in standard proper conditions (24
The invested experimental protocol was authorized by the Jerash University Ethical Committee (Approval Number JU/14/12/2020). All experiments were performed according to the Canadian Council Guide.
All animals were observed daily to monitor their allergy to HCQ treatment, general well-being, morbidity, and mortality.
Blood samples were collected from all rats through the heart puncture technique. Hematological tests were conducted, following the coulter counters principle for a complete blood analysis profile. The following hematological parameters were determined using Beckman-Coulter AU 5800 (Version 3, Califonia, USA): Hemoglobin (Hb), red blood cell (RBCs) count, white blood cell (WBCs) count, thrombocytes, hematocrit (HCT), mean corpuscular volume (MCV), mean corpuscular hemoglobin (MCH), and mean corpuscular hemoglobin concentration (MCHC).
A single blood sample of each anesthetized rat (given 87 mg ketamine/kg of body weight and 13 mg xylazine/kg, IM) was collected at the terminal stage of the study to collect a sufficient blood volume (15 mL/kg). Blood samples were collected from the cardiac puncture of each rat in all groups and drawn in vacutainers tubes. Analysis of the entire blood, plasma, and serum samples of all rats was performed using an Auto-chemistry analyzer to conduct the following biochemical tests: albumin, total protein, glucose, alkaline phosphatase, amylase, creatine phosphate kinase (cpk), lactate dehydrogenase, alanine aminotransferase (ALT), aspartate aminotransferase (AST), urea, creatinine, uric acid, total cholesterol, total bilirubin, and triglyceride. Various biochemical parameters were measured using the Beckman Coulter LH 780 analyzers.
After the dissection, each rat in all groups was inspected with the naked eye and microscopic lenses to identify any anatomical abnormalities in the spleen, testes, heart, liver, and kidneys.
The tissue blocks from the spleen, tstis, heart, liver, and kidneys were taken and processed for further histological examination, including fixation, dehydration, clearing, wax impregnation, embedding, and sectioning. Paraffin sections (4–5 µm) of all experimental rats in all groups were applied, using the following stains: hematoxylin and eosin stain for general structure, trichrome stain, Prussian blue reaction for hemosiderin, reticulin stain for reticular fibers, and Periodic acid-Schiff (PAS) for glycogen [23, 24, 25]. Histological sections were examined using an Olympus BX40 Polarized Light Microscope. However, a digital microscopic camera was used to take the photography of the sections.
The hematological and biochemical data changes, induced by chronic exposure to
HCQ, were subjected to statistical analysis. The obtained data were tested using
by student t-test, and p
No mortality was recorded in any HCQ-treated rat. However, evidence of back arching was noted, an indication of visceral pain and nose bleeding, during the clasping of injection-related to thrombocytopenia.
In comparison with the hematological references, the control rats showed normal hematological characterization values [26]. As shown in Table 1, animals subjected to HCQ showed a lowered significant value in erythrocytes, hemoglobin, hematocrit, platelets, leucocytes, and lymphocytes than those in the control group. A reduction in the value of all these blood components was dose-dependent. However, the values of erythrocytes MCV, MCH, MCHC, and RDW-SD did not show a significant change in all rats subjected to HCQ compared with the control rats.
Tests | Experimental groups | |||
Control | 40 mg/kg HCQ | 60 mg/kg HCQ | 120 mg/kg HCQ | |
Thrombocytes (10 |
295.5 |
100 |
42 |
34 |
Hb (g/dL) | 13.25 |
9.6 |
6.4 |
5.5 |
WBCs (10 |
8.25 |
3 |
1.7 |
1.4 |
RBCs (Millions/mm |
7.4 |
5.3 |
3.6 |
2.9 |
HCT (PCV) (%) | 40.95 |
28.9 |
18 |
15 |
MCV (πm |
17.95 |
18.3 |
18.8 |
19.25 |
MCH (Pg) | 55.2 |
54.7 |
53 |
54 |
MCHC (g/dL) | 32.5 |
32.7 |
34 |
33.83 |
LYM (%) | 79 |
51.85 |
56.8 |
60.65 |
LYM (#) | 6.55 |
1.55 |
0.94 |
0.76 |
RDW-SD (%) | 30.15 |
31.45 |
29.2 |
29.25 |
RDW-CV (%) | 13.85 |
14.95 |
13.1 |
12.75 |
Abbreviations: HCQ, hydroxychloroquine; Hb, hemoglobin; WBCs, white blood cells; RBCs, red blood cells; HCT, hematocrit; LYM (%), lymphocytes percent; LYM (#), lymphocytes number; MCH, mean corpuscular hemoglobin; MCHC, mean corpuscular hemoglobin concentration; MCV, mean corpuscular volume; RDW-SD, relative distribution width of RBC by volume-standard deviation; RDW-CV, relative distribution width of RBC by volume-coefficient of variation.
(*) means significant difference (p
A significant decline in the hematological indices (p
Compared with the control animals, HCQ elevated significantly the values of AST
and ALT, amylase, alkaline phosphatase, lactate dehydrogenase, cholesterol, and
chlorine ions (p-value
The test | Experimental groups | |||
Control | 40 mg/kg HCQ | 60 mg/kg HCQ | 120 mg/kg HCQ | |
Albumin (g/L) | 28.23 |
29.93 |
28.33 |
28.47 |
Total protein (g/L) | 68.25 |
76.55 |
75.15 |
78.63 |
Creatinine (mg/dL) | 0.485 |
0.43 |
0.31 |
0.29 |
ALT (IU/L) | 281 |
315 |
380.5 |
402.3 |
AST (IU/L) | 325.3 |
723.33 |
741 |
792.5 |
Amylase (IU/L) | 1957.5 |
1891 |
2488 |
3568.7 |
Glucose (mg/dL) | 117.65 |
93.35 |
88.95 |
75.4 |
Cholesterol (mg/dL) | 58.85 |
83.1 |
72.75 |
71.7 |
Total bilirubin (mg/dL) | 0.4 |
0.5 |
0.27 |
0.2 |
Direct bilirubin (mg/dL) | 0.02 |
0.01 |
0.01 |
0.2 |
GGT (IU/L) | 5.95 |
5.45 |
5.75 |
2.6 |
Alkaline phosphatase (IU/L) | 175.5 |
355 |
427 |
516 |
High-density lipids (HDL) (mg/dL) | 20.4 |
23.3 |
27.35 |
26.27 |
Low-density lipids (LDL) (mg/dL) | 13.65 |
18.05 |
19 |
20.87 |
Phosphate (mg/dL) | 13.76 |
11.68 |
9.15 |
10.82 |
Iron (mg/dL) | 164 |
170.5 |
180.5 |
178.3 |
LDH28 (IU/L) | 1666.7 |
3305.5 |
3087.5 |
3115.5 |
Triglyceride (mg/dL) | 89 |
70.85 |
69.6 |
59.7 |
Urea (mg/dL) | 45.5 |
52.27 |
45.8 |
46.76 |
Uric acid (mg/dL) | 10.65 |
3.35 |
3.22.36 |
3.5 |
BUN (mg/dL) | 21 |
23.7 |
22.3 |
22 |
LDL-C | 97 |
108 |
109.3 |
110 |
Calcium (mg/dL) | 10.5 |
9.2 |
9.2 |
9.6 |
Sodium (mmol/L) | 145.27 |
142.67 |
147.15 |
155.16 |
Potassium (mmol/L) | 13.86 |
11.5 |
11.7 |
9 |
Chlorine (mmol/L) | 79.225 |
98.61 |
98.89 |
101.5 |
(*) indicates a significant difference (p
HCQ-dose rats also demonstrated no significant alteration in the value of albumin, urea, Blood urea nitrogen (BUN), HDL, sodium ions, and iron. However, they demonstrated a significant reduction in the values of glucose, total protein, creatinine, triglycerides, and uric acid. Additionally, HCQ exposure did not significantly affect the serum levels of potassium, phosphorus, and sodium but significantly elevated the level of chlorine ions. The stability of serum phosphoremia and a drastic lowering of blood cells may not be associated with the hemolytic effect of HCQ, due to its effects on hemopoiesis. However, the biochemical findings of the current study are in agreement with that of other reports [28]. Moreover, the findings are in agreement with several case studies where hypoglycemia was caused by chronic exposure to HCQ [28, 34]. However, the biochemical alterations shown in this study agreed with previous studies, in which the HCQ-treated group showed significant elevations in serum AST, ALT, alkaline phosphatase, creatinine, and uric acid [28, 35].
The kidneys of the control animals showed normal renal histological components and normal nephrotic structures of the glomeruli, renal tubules, collecting tubules, and interstitial tissue (Fig. 1a,b). Compared with the control kidneys, the renal tissues of the rats exposed to HCQ demonstrated glomerular fragmentation, partial atrophy (Fig. 1c), proximal convoluted renal tubules (PCTs) hydropic degeneration, cloudy swelling (Fig. 1d), hyaline cast formation in the distal convoluted tubules (DCTs), and occasional interstitial edema next to the renal arteries (Fig. 1e). Some histopathological reports indicate that HCQ toxicity is responsible for the glomeruli size reduction, dilatation of Bowman’s space, and degeneration of renal tubules [28].
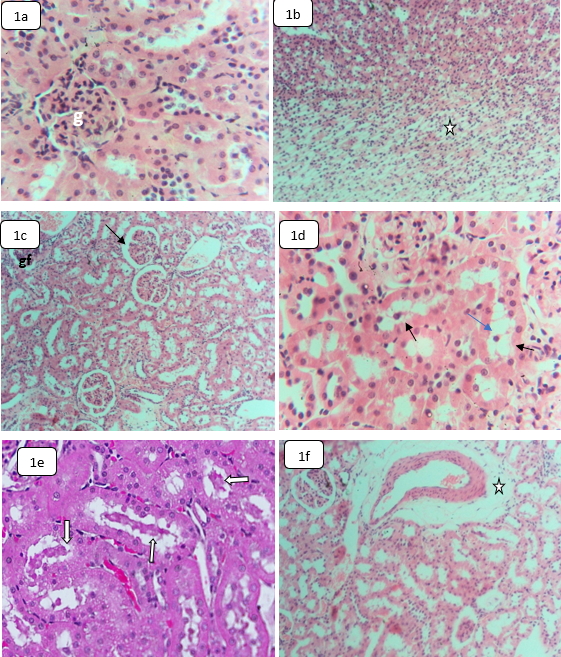
Light microphotographs sections in the kidneys of control and
HCQ-treated rats stained by H&E showing: (a,b) Control kidneys, (a) Normal
glomerulus (g) and renal tubules,
Treated rats’ renal cells demonstrated swelling and cytolysis with occasional brush border detachment of the PCTs’ epithelial lining. Previous studies indicated that HCQ was a potential risk to the function and structure of the kidney [6, 36]. Our findings show that the PCTs cause more injury than the distal convoluted tubule. This might be associated with the high blood volume received by the cortex and the proximal tubules compared with the medulla and the distal parts, respectively [37]. However, the hyaline casts’ appearance in the renal tubules of HCQ-treated rats may suggest subsequent glomerulonephritis caused by HCQ because of the ion pump disturbance across the renal tubules [6, 36].
Some studies reported a low risk of renal disorders among patients with rheumatoid arthritis and lupus nephritis, treated with HCQ [38, 39, 40]. An et al. [39] concluded that HCQ could protect patients from lupus erythematosus immune inflammatory lesions that disrupted the lysosomal-autophagy pathway and promoted renal cell senescence [39]. Catfish exposed to HCQ also showed a reduction in glomeruli, dilatation in Bowman’s space and deterioration in renal tubules [28]. However, Pari and Murugan [36] reported renal tubule hemorrhage and necrosis with cloudy swelling, resulting in reactive free radicals induced by oxidative stress, caused by HCQ treatment [40]. Additionally, El-Shishtawy et al. [35] found that rats treated with HCQ had glomeruli hypercellularity, hydropic degeneration, hemorrhage, inflammation, tubular necrosis, and hazy swelling. Ramesh et al. [32] also reported renal cell necrosis in the renal tissues of rats subjected to HCQ. Furthermore, HCQ attenuated renal damage by CTSB downregulation and the activation of CTSL-mediated NLRP3 inflammasomes showed significant attenuation of the renal dysfunction, based on a reduction in serum creatinine [6].
The liver of the control animals demonstrated normal intact hepatic tissues with preserved zonal accentuation. The hepatic lobular architecture exhibited normal hepatocytes, hepatic portal triads, Kupffer cells, and sinusoids (Fig. 2a). The liver of HCQ-treated rats has demonstrated little hepatic histological and histochemical alteration, leading to Kupffer cells hyperplasia and occasional hepatocytes injury in form of dysplasia and pyknosis (Fig. 2b). Additionally, the hepatic tissue of HCQ-treated rats showed inter-hepatic bile duct hyperplasia (Fig. 2c). Some hepatocytes exhibited nucleomorphism, vesiculation, binucleation, multinucleation, and irregularly clumped chromatin. These findings may demonstrate DNA fragmentation and apoptosis potential [41, 42]. However, some recent studies reported that HCQ could induce dilation of the hepatic central vein, vacuolization, hepatocyte degeneration, and vacuolization with pyknotic nuclei [28]. The liver of HCQ-exposed catfish demonstrated dilation of the lobular central vein, vacuolization of hepatocytes nuclear, and degeneration of pyknotic nuclei [28]. Previous case studies also showed hepatic failure after prolonged use of HCQ [43]. However, no necrotic or degenerative alterations were noted; no glycogen storage was affected, and no hemosiderin precipitate was observed in the liver of rats subjected to HCQ. Moreover, portal vessels and central veins were not affected.
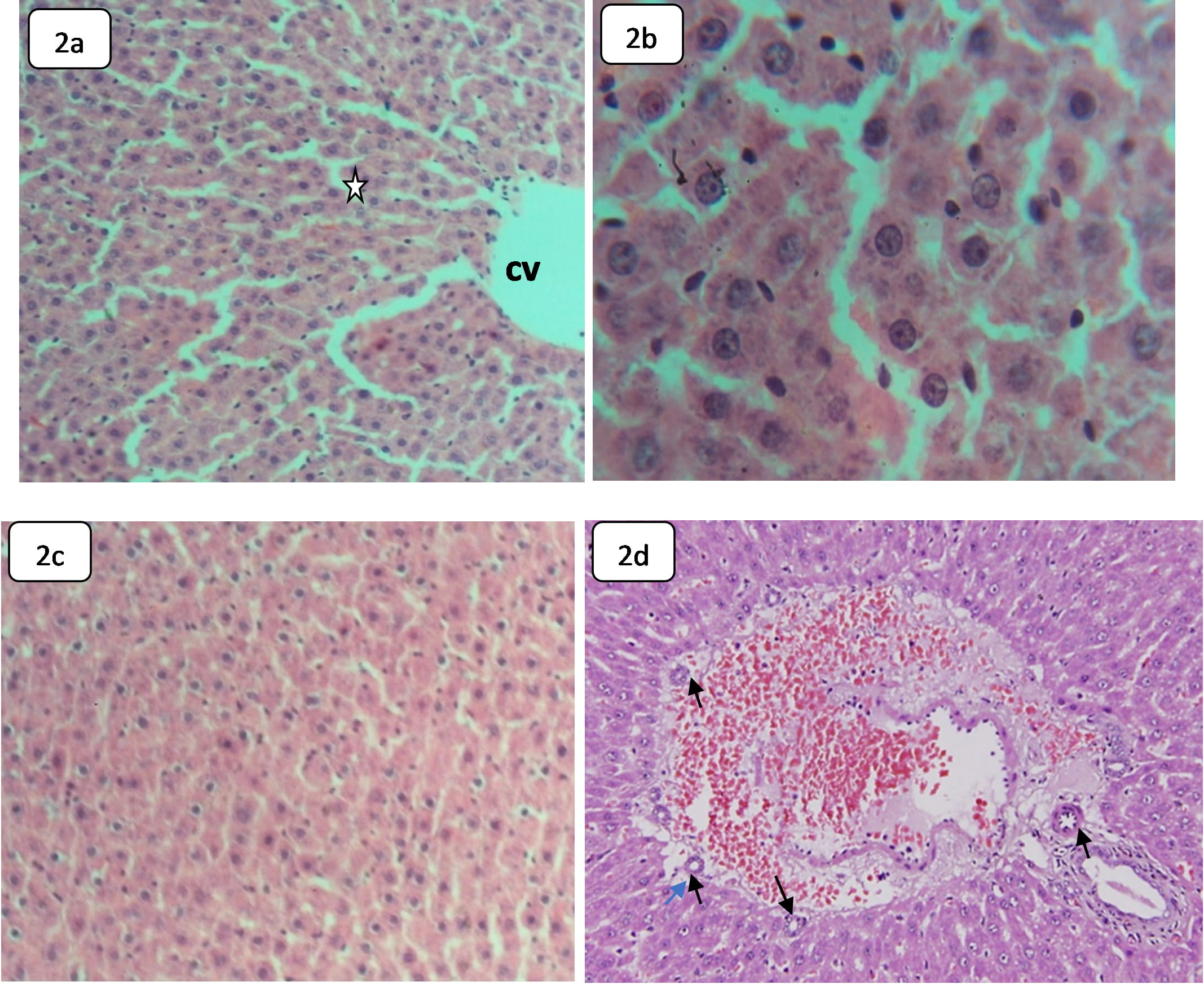
Light microphotographs of H&E stained sections in the liver of
the control and HCQ treated rats demonstrating: (a,b) Sections of control rats, (a) Normal hepatic architecture and normal hepatic strands (star),
The liver performs a vital role in the metabolism, excretion, and detoxification of chemicals, drugs, and other xenobiotic materials that can make the hepatic tissues susceptible to potential toxic effects [33]. The absence of serious abnormality in the liver of HCQ-treated rats may indicate that the toxicity of this drug does not target the liver. However, hepatocerebral degeneration, feathery degeneration, sinusoidal dilation, hydropic degeneration, and hepatocyte pyknosis have been reported in HCQ-treated albino rats [36, 41].
Control rats showed normal cardiac branched myofibers, arranged in bundles. The myocardial fibers are attached via well-preserved intercalated discs with normal striation and central oval nuclei (Fig. 3a). Moreover, the control hearts demonstrated normal cardiac interstitial tissue rich with blood capillaries.
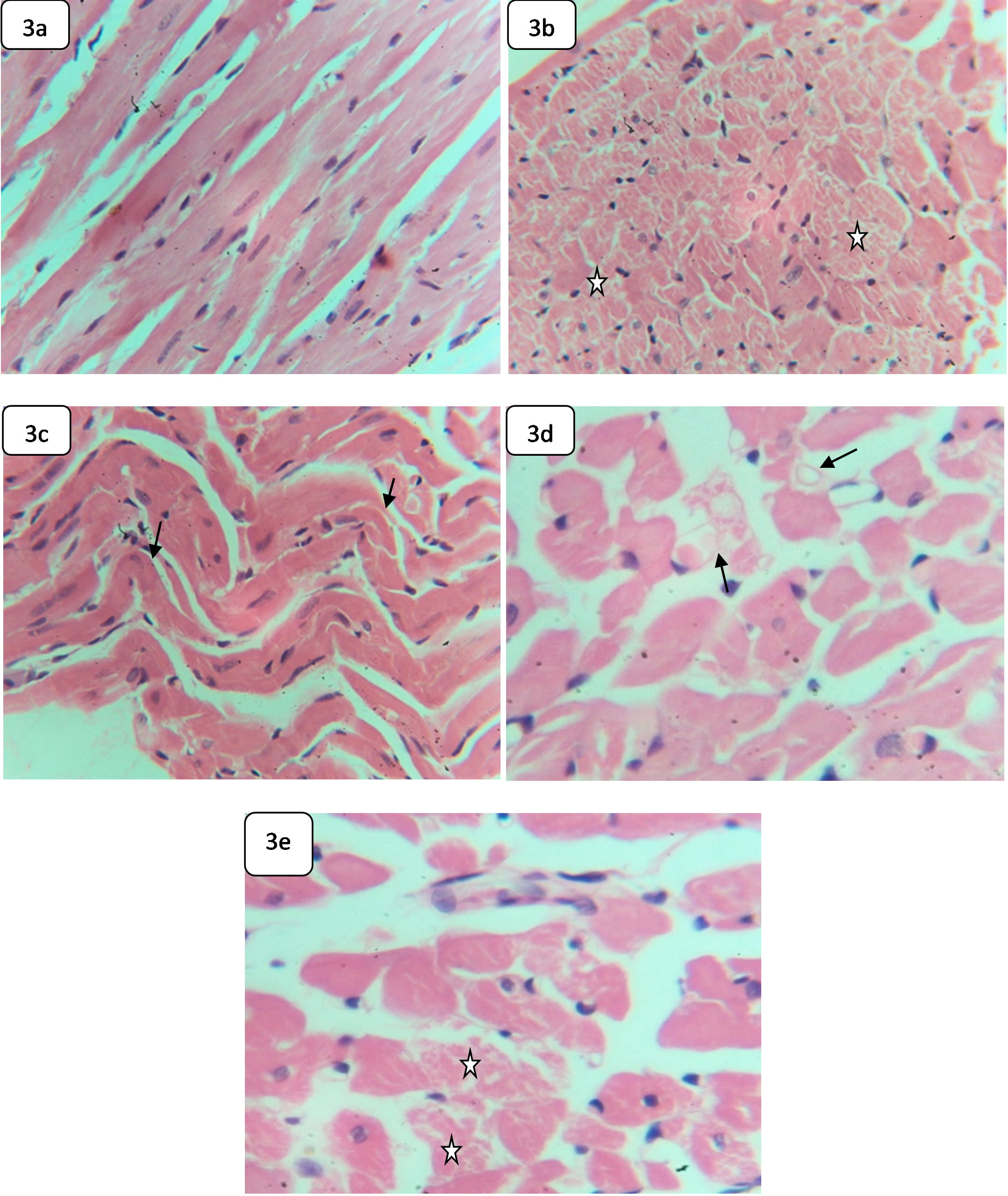
Light microphotographs of H&E-stained sections in the heart of
control and HCQ-rats demonstrate: (a) Hepatic tissue of control rat, showing
normal bundles of branched myofibres,
The heart of rats exposed to HCQ exhibited myofiber necrosis (Fig. 3b), a wavy appearance (Fig. 3c), disorganization, and disarray (Fig. 3d). Rats exposed to HCQ also exhibited occasional cardiac myolysis and nonparallel arrangement myofibers (Fig. 3e).
The induced cardiac alterations may reveal that the cardiac myofibers are affected by exposure to HCQ. The induced wavy appearance in the cardiac tissues may reveal myocardial degeneration, indicating an impact on the pump of the heart, proceeding toward a heart attack [44]. Additionally, the induced myofiber disarray might be associated with non-contractile scar tissue formation and heart rate reduction, which are features of hypertrophic cardiomyopathy [45]. Cardiomyocyte disorganization has also been reported, indicating hypertrophic cardiomyopathy [46]. Case studies have shown that prolonged use of HCQ could induce cardiomyopathy and heart failure [44]. Some studies have demonstrated hydropic degeneration, necrosis, interstitial hemorrhage, and vascular congestion in the vital organs of HCQ-treated rats [35].
The testes of the control rats showed normal seminiferous tubule histological structures, demonstrating normal spermatogenic cells and interstitial tissues (Fig. 4a,b). In comparison with the control testes, the following histological changes were observed in the testicular tissues of HCQ-treated rats comprising, spermatogenic cell sloughing, spermatocytes degeneration, testicular interstitial edema, and occasional spermatogenic arrest (Fig. 4c–e).
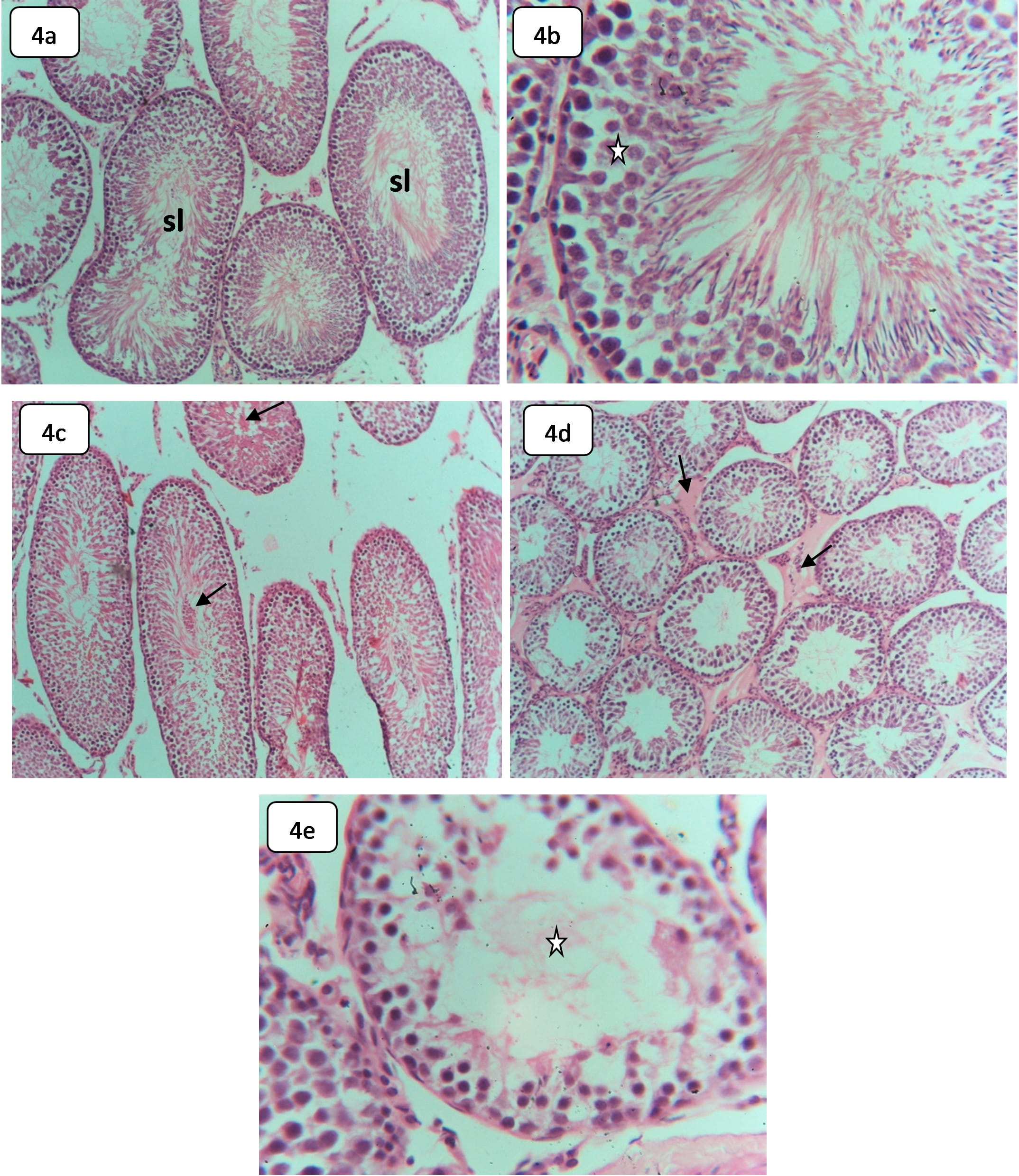
Light microphotographs of H&E-stained sections in the testes of
control and HCQ-treated rats showing: (a,b) Sections in the control testes, (a) demonstrating normal seminiferous tubules (sl),
Spermatid sloughing may indicate spermatocyte disruption of the testicular tissue skeleton induced by HCQ toxicity [47]. However, spermatogenesis disturbance might have resulted from spermatocyte degeneration or Leydeg cell population abnormality [46].
This finding agrees with that of Kavanagh et al. [48] where chronic exposure to HCQ-depressed testosterone decreases sperm count, increases spermatozoon morphological abnormality, and increases the organ index of the testes and epididymis, seminal vesicle, and prostate. The testicular toxicity of HCQ might be related to several enzymatic activities, needed in spermatogenesis, such as succinate dehydrogenase, glucose 6-phosphate dehydrogenase, and alteration in mitochondrial NADH and cytochrome C. However, Vawda and Saade [49] reported that HCQ could be harmful at the testicular and epididymal levels.
The control spleen demonstrated normal lymphoid white pulp and hematogenous red pulp, separated by prominent marginal zones. The white pulp showed periarteriolar lymphocyte sheaths (PALS), surrounding the follicles (Fig. 5a). The splenic red pulp showed prominent RBCs and venous sinuses, associated with B cells-rich PALS, macrophages, and plasma cells. Furthermore, the white pulp of the control spleens demonstrated normal follicles with central arterioles, surrounded by T cells-rich PALS and fibrous concentric layers (Fig. 5b).
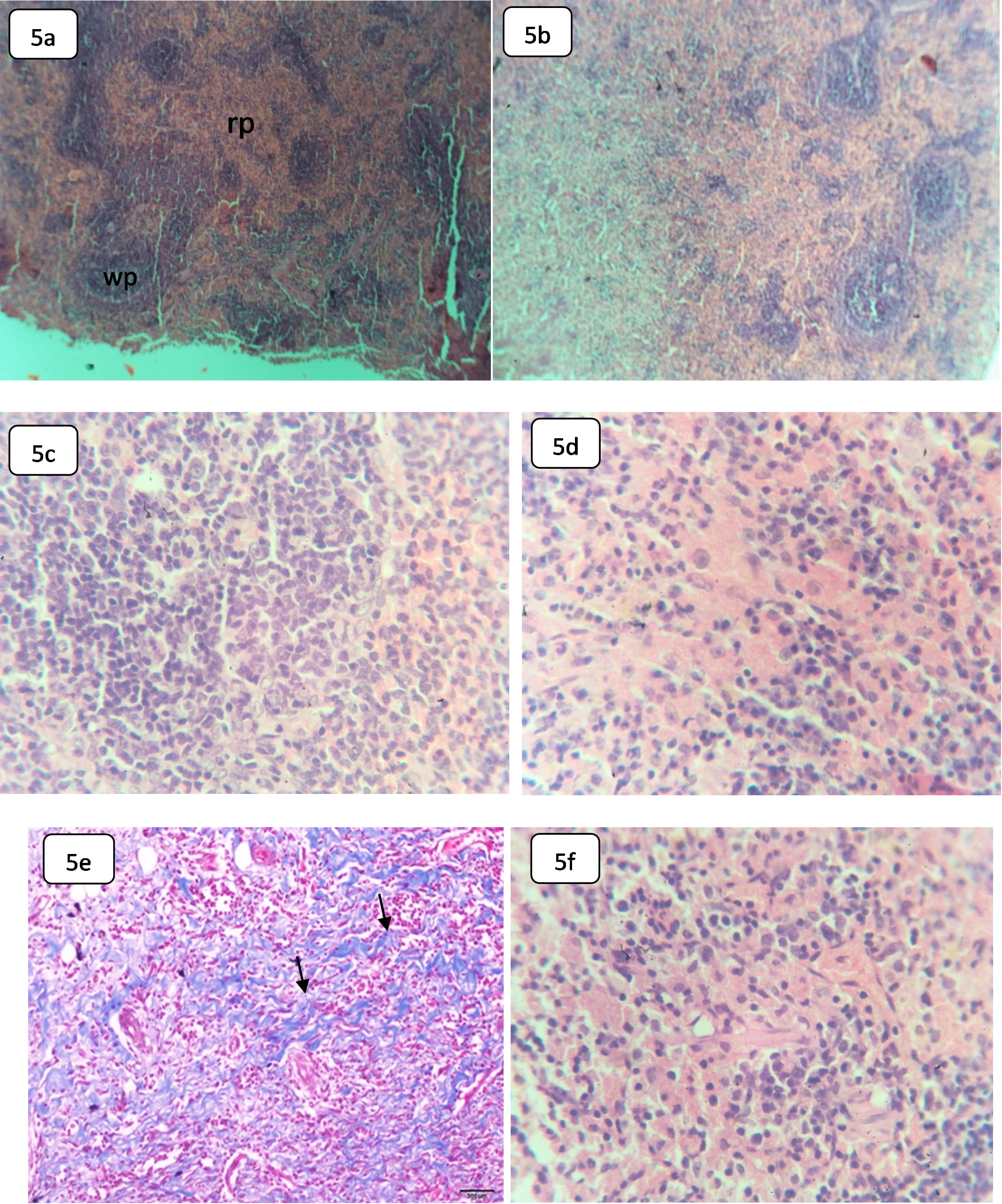
Light microphotographs of H&E stained sections in the spleen of
control and HCQ-rats show: (a) Splenic tissue of control rat, showing normal red
pulp (rp) and the lymphoid white pulp (wp),
The spleen of rats, subjected to HCQ, exhibited histological alterations in both red pulp and white pulp. The white pulp showed a decline in the size, the number of follicles, and a decline in T-rich-cells PALS number with apoptotic activity (Fig. 5b). Additionally, the germinal center of the follicles showed a decrease in size compared with the control rats. However, the red pulp demonstrated diffuse and a decline in B-rich-lymphocytes with macrophage infiltration and occasional multinucleated giant cells (Fig. 5d).
Collectively, the alterations in the white pulp may indicate the suppression of T-independent humoral immune response [6]. A decrease in cellularity at both the follicles and the marginal zone might have resulted from apoptosis at both T-cells and B-cells [50]. The spleen is the largest immune organ in the human body with hematopoietic and lymphatic components and is being targeted by several chemicals, toxins, and drugs, in which injury may be in both red and white pulps. This immune organ performs a critical role in removing ruptured and degenerated red blood cells.
Hydroxychloroquine has demonstrated efficacy in treating some autoimmune diseases and in decreasing immune system activity. This drug promotes CD8 T-cell stimulation but inhibits CD4 T-cell stimulation [34]. This combined effect has a beneficial action in autoimmunity and in reducing the infections opportunity.
The rise in the use of hydroxychloroquine has raised concerns about its potential risks to human health. Our findings show that chronic subjection to HCQ could alter the hematological, biochemical, and histological components and the function of the body’s vital organs. HCQ toxicity might be related to the formation of some oxidative materials that lead to the production of reactive oxygen species. Further studies must better understand the potential risk of hydroxychloroquine.
ALT, Alanine aminotransferase; AST, Aspartate aminotransferase; BUN, Blood urea nitrogen; cpk, creatine phosphate kinase; DCTs, Distal convoluted tubules; Hb, Hemoglobin; HCQ, Hydroxychloroquine; HCT, Hematocrit; HDL, High-density lipids; H&E, Hematoxylin and eosin; MCH, Mean corpuscular hemoglobin; MCHC, Mean corpuscular hemoglobin concentration; LDL, Low-density lipids; LDL-C, Low-density lipoprotein cholesterol; LYM, Lymphocytes; PAS, Periodic acid-Schiff; PCTs, Proximal convoluted tubules; PALS, periarteriolar lymphocytes sheaths; PCV, Packed cell volume; RBCs, Red blood cells; RDW-CV, Relative distribution width of RBC by volume-coefficient of variation; RDW-SD, Relative distribution width of RBC by standard deviation; WBCs, White blood cells.
The datasets used and/or analyzed during the current study are available from the corresponding author on reasonable request.
Conceptualization—MeA, BJ, QJ, LCM; Data curation—BJ, QJ, KWG, SM; Formal analysis—MeA, BJ, QJ, LCM; Funding acquisition—MeA, MaA, KWG, CA, LCM; Investigation—MeA, BJ, QJ, LCM; Methodology—BJ, QJ; Project administration—MeA, BJ, LCM; Supervision—MeA, BJ, MaA, KWG; Validation—BJ, QJ, CA, LCM; Writing—original draft—BJ, QJ, CA, LCM; Writing—review and editing—MeA, MaA, KWG, SM, CA, LCM. All authors have read and agreed to the published version of the manuscript.
The invested experimental protocol was authorized by the Jerash University Ethical Committee (Approval Number JU/14/12/2020). All experiments were performed according to the Canadian Council Guide.
The support of Isra University is highly appreciated during the conduction of the present study. Moreover, the authors are thankful for Jerash University for putting all the facilities under their disposal to the achieve the present work.
This work was funded by the Deanship of Scientific Research of Jouf University under grand NO (DSR-2021- 01-03179).
The authors declare no conflict of interest.
Publisher’s Note: IMR Press stays neutral with regard to jurisdictional claims in published maps and institutional affiliations.