- Academic Editor
The microbiome has a major impact on human physiology and plays a critical role in enhancing or impairing various physiological functions such as regulation of the immune system, metabolic activities, and biosynthesis of vitamins and hormones. Variations in the gut microbial community play a critical role in both health and disease. Regulation of calcium and bone metabolism, as well as cellular functions such as proliferation, apoptosis, differentiation, and immune modulation, are among the known effects of vitamin D. These biological functions are primarily carried out through the binding of vitamin D to the vitamin D receptor (VDR), a member of the nuclear receptor superfamily. The immunomodulatory properties of vitamin D suggest that this molecule plays an important role in various diseases. Maintenance of immune homeostasis appears to occur in part through the interaction of the gut microbiota with vitamin D. Increasing evidence points to the central role of vitamin D in maintaining mucosal barrier function, as vitamin D deficiency has been associated with disruption of gut barrier integrity, translocation of bacteria into the bloodstream, and systemic inflammation. In parallel, a bidirectional interaction between vitamin D and the gut microbiota has been demonstrated as data show upregulation of intestinal VDR expression and downregulation of inflammatory markers in response to fermentation products. The aim of this review is to provide an overview of the evidence of a link between the gut microbiome and vitamin D, with a focus on data from experimental models and translational data from human studies related to vitamin D-induced changes in gut microbiota composition.
The gut microbiota, also known as the intestinal flora, is a complex ecosystem of microorganisms such as bacteria, fungi, viruses, parasites, and archaea that live in symbiosis in the human intestinal lumen [1, 2]. These microorganisms are mainly host–specific, and their composition and numbers vary depending on the host species and microenvironment [3]. The gut microflora plays an important direct and/or indirect role in a variety of physiological processes, including regulation of the immune system, modulation of neurotransmitters and hormones, and production of metabolites and antioxidants. Emerging evidence suggests that the gut microbiota plays a critical role in alleviating various systemic diseases [3]. The gut microbiome is considered a new active organ due to its ability to interact with host biology and integrate systemically [4, 5]. Changes in the composition or number of the gut microbiota disrupt the commensal relationship with the host and have negative consequences for human health [6, 7, 8].
Vitamin D/vitamin D receptor (VDR) signaling contributes significantly to the immunological, genetic, environmental, and microbial aspects of human health and disease [8, 9]. The human VDR is the first identified gene that functions as an essential host factor and genetically influences the modulation of the gut microbiome [10]. Several studies have demonstrated the crucial function of vitamin D and its receptor in maintaining a healthy gut [11, 12].
The aim of this review is to provide an overview of the data linking the gut microbiome to vitamin D and VDR, with a focus on data from experimental models and translational data from human studies related to changes in gut microbiota composition caused by vitamin D.
Our review focused on quantitative studies published in peer-reviewed English language journals and available as full text online or through a library. We considered randomized controlled trials, comparative studies, multicenter studies, observational studies, clinical trials, review articles, systematic reviews, meta-analyses, and case reports.
We performed a thorough search of the MEDLINE, COCHRANE, and PubMed databases to
find relevant articles from the beginning of each database to February 28, 2023.
We used Boolean operators (AND, OR, NOT) to combine search terms and narrow the
search results because the topic included multiple keywords. Search terms
included “vitamin D”, “vitamin D receptor”, “VDR”, “25(OH)D”, “25
hydroxyvitamin D”, “1,25(OH)
Vitamin D, a fat-soluble vitamin, occurs in two forms, ergocalciferol or vitamin
D
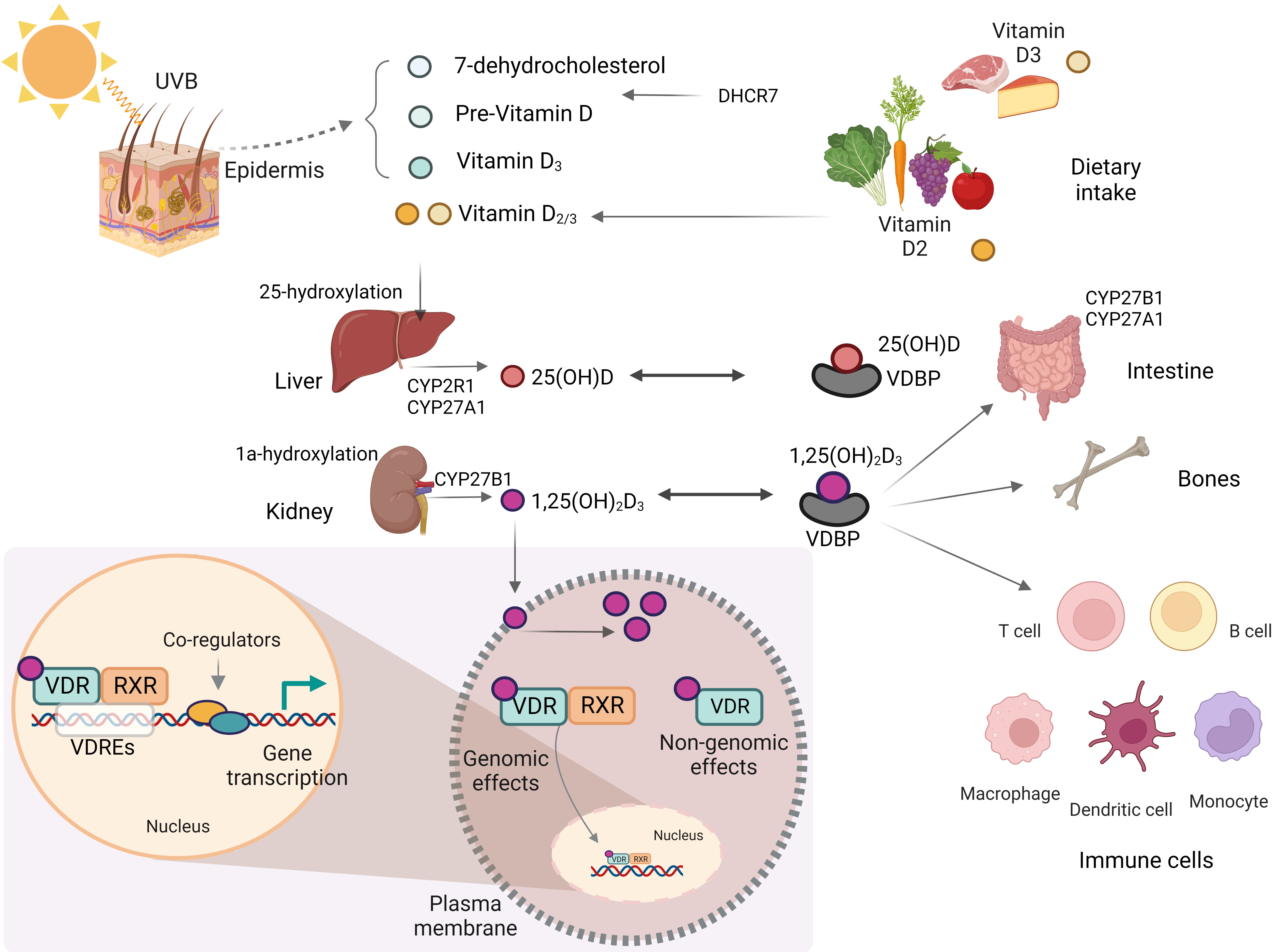
Biosynthesis and metabolism of vitamin D. This figure was created using BioRender (https://biorender.com, accessed February 13, 2023). UVB, ultraviolet B; CYP2R1, cytochrome P450, family 2, subfamily R, polypeptide 1; CYP27A1, cytochrome P450, family 27, subfamily A, polypeptide 1; CYP27B1, cytochrome P450, family 27, subfamily B, polypeptide 1; VDR, vitamin D receptor; VDRE, vitamin D response element; RXR, retinoid X receptor; DHCR7, 7-dehydrocholesterol reductase; VDBP, vitamin D-binding protein.
Until their enzymatic hydroxylation, these forms remain biologically inactive
[16]. After vitamin D
In addition to the classical effects of vitamin D in regulating bone mineralization and maintaining systemic calcium homeostasis, non-classical effects such as regulation of cell proliferation and differentiation, hormone secretion, and immune system function have recently been reported [16, 17].
The VDR acts as a transcription factor and controls the expression of genes
involved in the biological effects of vitamin D. Binding of 1,25(OH)
The protective barriers of the mucus layer and intestinal epithelium prevent bacteria and their products from entering the interstitial space, thus preventing the development of intestinal inflammation [28]. However, disruption of the homeostasis of the gut microbiota and the subsequent entry of immunogenic byproducts into the interstitial space can trigger an inflammatory response by activating the immune system [29].
In the human body, the total number of bacterial cells ranges from 10
Vitamin D has been shown to alter the composition of the gut microbiome in
several experimental animal models (Table 1, Ref. [42, 43, 44]) [45]. Genome-wide
association studies have shown that variations in the human VDR gene are
associated with changes in the gut microbiota [10], whereas, by contrast, the
absence of VDR in the gut results in dysbiosis in mice [46]. Two VDR
polymorphisms were significantly associated with changes in microbiota
composition in a mixed cohort [10]. In this study, VDR genetic variants affected
the genus Parabacterioides (phylum: Bacteroidetes), while examination of
VDR knockout (KO) mice revealed higher levels of Parabacteroides
compared to wild-type (WT) mice [10]. Vitamin D deficiency caused by dietary
restriction, CYP27B1 depletion, or VDR depletion in experimental models resulted
in an increase in the Bacteroidetes population and Proteobacteria phyla [42].
Vitamin D supplementation or hypovitaminosis had little effect on gut microbiota
composition at the phylum level; however, significant differences occurred at
lower taxonomic levels [44]. In CYP27B1 KO mice, deficiency
of 1,25(OH)
Study [ref.] | Model used | Intervention/Parameter | Study duration | Outcomes | |
Microbial alterations | Other | ||||
Assa et al., 2014 [42] | Weaned C57BL/6 mice | Vitamin D dietary intake: sufficient vs. deficient | 5 weeks | Vitamin D deficiency |
1,25(OH) |
- Increase in Bacteroidetes, Firmicutes, Actinobacteria, and Gammaproteobacteria | - Reduction of Escherichia coli O157:H7-induced decrease in transepithelial electrical resistance | ||||
- Reduction of permeability | |||||
- Barrier integrity preservation | |||||
Vitamin D-deficient mice challenged with Citrobacter rodentium | |||||
- Increase in colonic hyperplasia | |||||
- Epithelial barrier dysfunction | |||||
Jin et al., 2015 [43] | VDR KO and WT C57BL/6 mice | VDR KO vs. WT mice | NA | VDR KO mice - fecal stool |
VDR status affected NLR signaling |
- Increase in Clostridium and Bacteroides Sphingobacteria-to-Sphingobacteriaceae Decrease in Lactobacillus | |||||
VDR KO mice - cecal stool |
|||||
- Increase in Eggerthella | |||||
- Decrease in Alistipes and Odoribacter | |||||
Zhu et al., 2019 [44] | WT and Cyp27b1 KO mice | Cyp27b1 KO mice fed a rescue diet (1.25% phosphorus, 2% calcium, 20% lactose) vs. CYP27B1 KO mice subcutaneously injected with 1,25(OH) |
Rescue diet or 1,25(OH) |
1,25(OH) |
1,25(OH) |
- For 10–12 weeks | - Increase in Akkermansia muciniphila, Solitalea Canadensis | - Decrease in the colonic mucus layer | |||
or | - Decrease in Bacteroides acidifaciens, Bacteroides plebeius | - Increase in bacterial translocation to mesenteric lymph nodes | |||
- Until mice reached 8–10 months of age | 1,25(OH) |
VDR, vitamin D receptor; KO, knockout; WT, wild-type; NA, not applicable; NLR, nucleotide-binding oligomerization domain-like receptor.
A significant correlation between gut microbiota composition and vitamin D has also been demonstrated in human studies (Table 2, Ref. [10, 49, 50, 51, 52, 53, 54, 55, 56]). In healthy individuals, high vitamin D intake resulted in increased levels of Prevotella and lower levels of Haemophilus and Veillonella in stool samples [52]. In addition, bacterial enrichment varied in individuals with high or low serum 25(OH)D concentrations, as higher vitamin D levels were associated with increased Megasphaera concentrations and lower Veillonella and Haemophilus concentrations [52]. By contrast, another study found that vitamin D supplementation was inversely related to Prevotella concentrations and strongly associated with Bacteroides in healthy individuals [49]. Singh et al. [55] demonstrated that vitamin D supplementation significantly increased the diversity of the gut microbiota, resulting in a higher proportion of Bacteroidetes compared to Firmicutes, in addition to an increase in the abundance of beneficial microorganisms such as Bifidobacterium and Akkermansia.
Study [ref.] | Patients included (n) | Study design | Intervention/Parameter | Study duration | Outcomes | |
Microbial alterations | Other | |||||
Wu et al., 2012 [49] | 98 | Cross-sectional study | Vitamin D dietary intake | NR | Long-term vitamin D intake → | NR |
- Increase in Bacteroides | ||||||
- Decrease in Prevotella | ||||||
Wang et al., 2016 [10] | 1812 | Genome-wide association cross-sectional | Genetic variants | NR | VDR gene and POMC gene were associated with |
NR |
Taxa significantly associated with VDR → | ||||||
- Parabacteroides | ||||||
- Unclassified Enterococcaceae | ||||||
- Unclassified Ruminococcaceae | ||||||
- Bacteroides | ||||||
Tabatabaeizadeh et al., 2016 [50] | 50 | Intervention study | Pre- vs. post-supplementation with 9 doses of 50,000 IU cholecalciferol pearls (1 dose/week) | 9 weeks | Post-supplementation → | NR |
- Increase in Bifidobacterium, Firmicutes | ||||||
- Decrease in Lactobacillus, Bacteroidetes | ||||||
Bashir et al., 2016 [51] | 16 | Interventional, open-label, pilot study | Pre- vs. post- supplementation → Weeks 1–4: Weekly vitamin D3 dose of 980 IU/kg body weight | 8 weeks | Post-supplementation → | Post-supplementation → |
Weeks 5–8: Weekly vitamin D3 dose of 490 IU/kg body weight | - Increase in Bacteroidetes (gastric antrum & duodenum) | - Increase in phylotype richness in the gastric antrum | ||||
- Decrease in Proteobacteria (gastric antrum & gastric corpus) and Gammaproteobacteria (upper GI tract) | - Trend toward an increase in phylotype richness in the duodenum | |||||
- Significant change in microbial composition in the upper GI tract | ||||||
Luthold et al., 2017 [52] | 150 | Cross-sectional study | Vitamin D dietary intake (highest tertile vs. 1st & 2nd tertile combined) | NR | Highest vitamin D intake tertile → | - Inverse correlation lipopolysaccharides with 25(OH)D |
- Prevotella is more abundant | - Inverse correlation of CRP & E-selectin with 25(OH)D | |||||
- Haemophilus & Veillonella aer less abundant | ||||||
Serum 25(OH)D (highest tertile vs. 1st & 2nd tertile combined) | Highest serum 25(OH)D → | |||||
- Megasphaera is more abundant | ||||||
- Haemophilus & Veillonella are less abundant | ||||||
Seura et al., 2017 [53] | 28 | Cross-sectional study | Vitamin D dietary intake | 3 days | No association with Bacteroides, Bifidobacterium, and Lactobacillales | NR |
Naderpoor et al., 2019 [54] | 26 | Double-blind, randomized clinical trial | Pre- vs. post-supplementation with cholecalciferol 100,000 IU dose at baseline followed by 4000 IU daily or matching placebo | 16 weeks | Post-supplementation → | NR |
- Increase in Lachnospira | ||||||
- Decrease in Blautia | ||||||
Individuals with 25(OH)D | ||||||
- Increase in Coprococcus | ||||||
- Decrease in Ruminococcus | ||||||
Singh et al., 2020 [55] | 80 | Intervention study | Vitamin D responders vs. non-responders post-supplementation of a weekly oral dose of 50,000 IU vitamin D3 | 12 weeks | Vitamin D supplementation → | NR |
- Increase in Bacteroidetes to Firmicutes ratio | ||||||
- Increase in Akkermansia and Bifidobacterium | ||||||
- Increase in Bacteroides/Prevotella ratio | ||||||
- Decrease in Ruminoccoccus | ||||||
- Increase in Bacteroides acidifaciens, Ruminococcus bromii, Bacteroides eggerthii, and Barnesiella intestinihominis in responders | ||||||
- Decrease in Bacteroides acidifaciens in non-responders | ||||||
Singh et al., 2022 [56] | 112 | Pilot study | Vitamin D deficient vs. non-deficient children | Vitamin D deficient children → | NR | |
- Increase in Bacteroidetes / Firmicutes ratio | ||||||
Vitamin D deficient children → | ||||||
- Gut enterotypes dominated by Prevotella as opposed to Bacteroides |
NR, not reported; VDR, vitamin D receptor; POMC, proopiomelanocortin; GI, gastrointestinal; CRP, c-reactive protein.
Administration of high-dose vitamin D supplements caused changes in the gut
microbiome of female adolescents; specifically, a weekly dose of 50,000 IU
cholecalciferol over a 9-week period resulted in a decrease in populations of
Bacteroidetes and Lactobacillus and an increase in populations of
Firmicutes and Bifidobacterium [50]. In parallel, vitamin D
supplementation caused significant changes in Bacteroides and
Prevotella, which showed a variation in enterotypes [55]. In a study by
Bashir et al. [51], analysis of stool samples and endoscopy and
colonoscopy biopsies demonstrated that 8 weeks of vitamin D
A study conducted in a pediatric population in Qatar showed that the gut microbial community differed significantly between groups with and without vitamin D deficiency, with individuals with deficiency having lower microbial diversity [56]. In addition, significant differences were observed in phyla Firmicutes and Bacteroidetes, with individuals with deficiency having a higher ratio of Bacteroidetes to Firmicutes than those without deficiency [56]. The same study showed that children with vitamin D deficiency had a higher concentration of the genus Prevotella than Bacteroides in the gut [56].
On the other hand, a retrospective case-control study of adult women with irritable bowel syndrome compared with healthy controls examined plasma 25(OH)D levels and gut microbiome composition and found no association between plasma 25(OH)D levels and bacterial richness, measures of alpha diversity including Shannon and Simpson indices or bacterial abundance in either group [57].
A systematic review examined the role of vitamin D on the gut microbiome in animals and humans [45]. The results of animal studies showed an increase in Bacteroidetes in the vitamin D-deficient and VDR KO groups [42]. Human studies have mainly been observational; 12 of 14 studies reported an association between vitamin D and the gut microbiome but with differences among studies [45]. A recent systematic review of 25 human studies examining the role of vitamin D on gut microbiota composition found that vitamin D supplementation was associated with greater changes in the composition of phyla Firmicutes, Actinobacteria, and Bacteroidetes [58]. Regarding alpha and beta diversity, high dietary vitamin D intake promoted changes in bacterial composition and/or influenced the richness of different species [58]. Vitamin D supplementation and an increase in 25(OH)D levels led to a decrease in the families of Veillonellaceae and Oscillospiraceae [58]. Functional profiling of the bacterial communities in the gut using Phylogenetic Investigation of Communities by Reconstruction of Unobserved States (PICRUST) analysis showed that there are metabolic pathways involved in lipid metabolism and fatty acid biosynthesis that play an important role in the uptake of vitamin D in the gut lumen [55].
These results suggest that vitamin D has a major impact on the distribution of intestinal flora, considering that the effects depend on the dosage and duration of vitamin D supplementation, as well as on the origin of the tissue for microbiome analysis [51, 54]. The question arises whether vitamin D has an effect on microbial richness either in the gastrointestinal tract or in stool samples, and caution should be exercised when collecting stool samples. In addition, methodological differences in the assessment of vitamin D origin (ultraviolet B radiation, dietary intake, serum 25(OH)D, dietary supplements) may lead to conflicting results.
There is increasing evidence that bacteria play an important role in altering
vitamin D metabolism. Several enzymes expressed by bacteria are involved in the
hydroxylation of steroids; consequently, these enzymes can induce vitamin D in a
similar manner as in humans [59]. The bacterial CYP105A1 (Streptomyces
griseolus) is responsible for the conversion of vitamin D
The presence of dysbiosis and alterations in the gut microbiota in autoimmune diseases is becoming increasingly clear [63]. Nevertheless, the exact way in which the immune system and the gut microbiota interact in the development of disease is not yet fully understood. Although the extent of dysbiosis may vary among autoimmune diseases, there is evidence that certain bacteria may promote or inhibit immune responses in different ways, suggesting that the microbiota may have a multifaceted influence on inflammatory diseases [63].
Commensal bacteria, toxins, and food antigens are involved in stimulating the immune response, which in turn leads to the initiation of inflammatory response; persistent inflammation can lead to the development of autoimmune diseases [64]. Recent evidence has shown that vitamin D signaling has a major impact on a variety of aspects of intestinal physiology and plays a critical role in maintaining intestinal homeostasis [65]. Vitamin D contributes to the activation and synthesis of pattern recognition receptors, various cytokines, and antimicrobial peptides (AMPs). It also plays an important role in modulating the gut microbiota, preventing bacterial overgrowth, and strengthening the integrity of the gut barrier [65]. In parallel, vitamin D positively affects intestinal tissues by promoting innate immune responses and attenuating T-cell-mediated inflammatory adaptive immunity, functions that are closely associated with the development of autoimmunity [66].
The intestinal mucosa serves as both a physical and functional barrier separating host cells from the microenvironment. It consists of several components that work together to fulfill its function, such as the commensal gut microbiota, an outer mucus layer, secretory immunoglobulin A (sIgA), and AMPs secreted into the mucus layer as immune-sensing molecules and regulatory proteins. The specialized epithelial cell layer and inner lamina propria also contribute to this function (Fig. 2) [67].
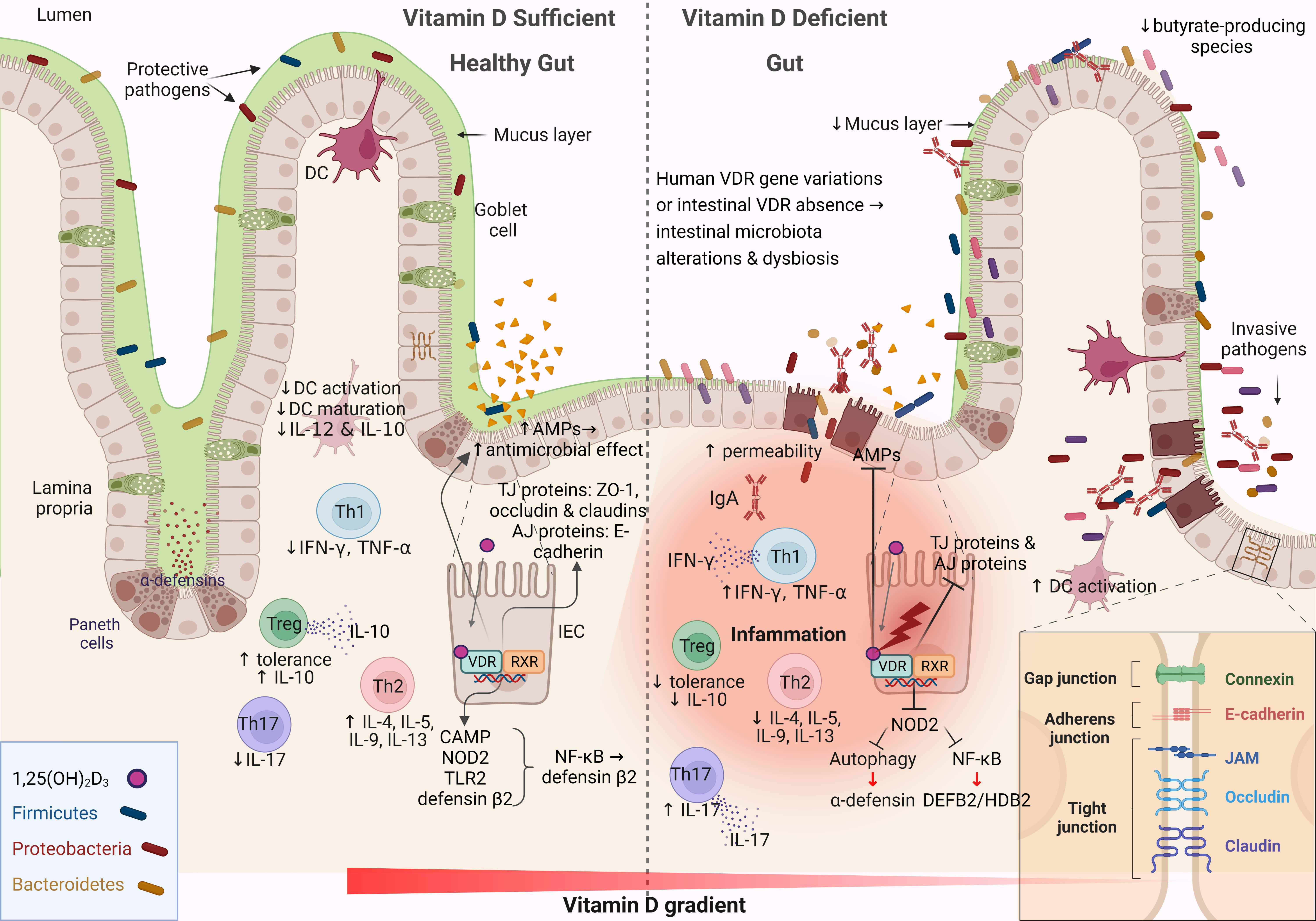
Schematic representation of the changes associated with the
intestinal barrier between a vitamin D-sufficient and vitamin D-deficient
dysbiotic gut. This figure was created using BioRender (https://biorender.com,
accessed February 13, 2023). DC, dendritic cell; IL-12, interleukin 12; Th, T
helper; TNF-
The IECs form a monolayer and are tightly connected by symmetrical structures called junctional complexes. The tight junctions (TJs) are located on the apical side of the cells and are responsible for ion transport and small molecule regulation (Fig. 2). The gut-associated lymphoid tissue is involved in both adaptive and innate immunity and consists of B cells, T cells, macrophages, and dendritic cells, which contribute critically to the immunological defense mechanisms of the gut barrier [68]. Immunoregulators such as sIgA and AMPs are secreted into the mucus layer to enhance the gradient separation of the microbiota from the epithelium to the lumen [69]. The sIgAs are produced by plasma cells, and their functions are diverse: they coat bacteria to facilitate their interaction with host immune cells, downregulate inflammation-associated epitopes, or neutralize toxins [70]. The composition of the mucus layer can influence the gut microbiota, and in parallel, the gut microbiota determines the properties of the mucus layer [71]. The IEC layer preserves the property of not being penetrated by pathogens and toxins through the function of TJs. Impairment of any aspect of the intestinal barrier, whether physical or functional, renders the host vulnerable to pathogen invasion, triggers a local immune response, and stimulates an inflammatory response in the gut (Fig. 2) [72].
Vitamin D–VDR signaling is critical for maintaining the integrity of the intestinal barrier by regulating apical junctions, adherent junctions, and proteins associated with TJs [73]. Defensin beta 4/human beta-defensin-2 (HBD-2) and cathelicidin antimicrobial peptide are stimulated directly and indirectly by vitamin D in human intestinal and monocyte cell lines through stimulation of the intracellular pattern recognition receptor nucleotide-binding oligomerization domain protein 2 (NOD2) [74, 75]. Stimulation of NOD2 by its ligand muramyl dipeptide induces HBD-2 gene expression (Fig. 2) [76]. These data support the hypothesis that AMPs have a major impact on the composition of the gut microbiota, whereas AMPs, in combination with IgAs, exert protective effects on the mucus layer [77].
Vitamin D–VDR signaling helps maintain intestinal barrier integrity by
regulating myosin light chain kinase (MLCK) and provides protection by preventing
nuclear factor kappa-light-chain-enhancer of activated B cells (NF-
There is growing evidence that the microbiome plays an important role in both health and disease. Variations in the human microbiome are closely related to a variety of physiological functions. In parallel, new data show that the effect of vitamin D on health is mediated in part by the microbiome. Vitamin D exerts its modulatory effects on gastrointestinal health by either regulating the composition and metabolic activity of the gut microbiome or modulating the functions of the physiological gut barrier and immune system. However, the effects of vitamin D on the gut microbiome are also closely related to VDR activation, as VDR is a crucial host factor that can influence the gut microbiome at the genetic level [82]. Several studies have demonstrated the association between vitamin D deficiency and the development of dysbiosis, while intervention studies have demonstrated vitamin D-induced changes in the composition of the microbiome, mainly through the induction of a health-promoting microbiota and the reduction of the Firmicutes/Bacteroides ratio.
Future research should focus on elucidating the mechanisms involved in the changes in gut microbiota composition associated with vitamin D status or supplementation and/or genetic impairment of VDR expression/activity. Thus, there is a great need for well-designed animal and human studies that allow consistent analyses of gut microbiota and assessment of vitamin D or VDR. In addition, the question of what dose of vitamin D supplementation results in specific changes in the gut microbiome that affect host health remains unresolved. Considering that the gut microbiome can provide valuable information to assess individual responses to vitamin D supplementation, future research should focus on intra- and inter-individual variability using multi-omics strategies. In parallel, the design of experimental animal studies is necessary to understand causality, as it is possible that microbial changes precede disease onset, suggesting a potential causal relationship or passive response to disease.
VDR, vitamin D receptor; VDBP, vitamin D binding protein; CYP2R1, cytochrome
P450, family 2, subfamily R, polypeptide 1; CYP27B1, cytochrome P450, family 27,
subfamily B, polypeptide 1; FGF23, fibroblast growth factor 23; VDRE, vitamin D
response elements; IEC, intestinal epithelial cell; KO, knock out; WT, wild type;
ZO-1, zonula occluden-1; PICRUST, phylogenetic investigation of communities by
reconstruction of unobserved states; AMP, antimicrobial peptide; sIgA, secretory
immunoglobulin A; TJ, tight junction; NOD2, nucleotide-binding oligomerization
domain protein 2; MLCK, myosin light chain kinase; NF-
Not applicable.
IA and ET: conducting research and investigation process, specifically performing the data/evidence collection; contributing to the visualization preparation, creation and presentation of the published work; contributing to the preparation, creation and presentation of the published work, specifically writing the initial draft (including substantive translation) and AM and CT: contributing to conceptualization ideas; formulation and evolution of review aims; contributing to management and coordination responsibility for the research activity planning and execution; contributing to preparation, creation and presentation of the published work, specifically critical review, commentary and revision—including pre- or post-publication stages. All authors read and approved the final manuscript. All authors have participated sufficiently in the work and agreed to be accountable for all aspects of the work.
Not applicable.
Not applicable.
This research received no external funding.
The authors declare no conflict of interest.
Publisher’s Note: IMR Press stays neutral with regard to jurisdictional claims in published maps and institutional affiliations.