Academic Editor: Agnieszka Paradowska-Gorycka
Background: To investigate the synergic effect and underlying mechanism
of Endostar, a recombinant human endostatin used for anti-angiogenesis, in
radiotherapy for cervical cancer. Methods: The Cell Counting Kit-8
(CCK-8) assay and plate cloning experiment were first employed to analyze the
proliferation of HeLa and SiHa cervical cancer cells and human umbilical vein
vascular endothelial cells (HUVECs). Flow cytometry was used to detect apoptosis
and cell cycle progression. A tube formation assay was used to assess
angiogenesis in vitro. The expression of gamma H2A histone family member
X (
As the leading cause of death from gynecological tumors worldwide [1], cervical cancer has been found to be strongly associated with persistent infection with high-risk human papillomavirus (HR-HPV) [2]. Although increased awareness and use of HPV screening and vaccination has helped to reduce the incidence and mortality of cervical cancer, the current 5-year survival rate for cervical cancer is only about 60% [3]. At present, surgery is the primary treatment option for patients with stage I–IIa cervical cancer, and radiotherapy can be used as a preoperative neoadjuvant treatment to reduce tumor volume prior to surgery, as well as a postoperative adjuvant treatment to eliminate residual tumor cells. However, because the efficacy of single agents still seems restricted, combination radiotherapies involving different groups of substances have been explored.
The hypervascular nature of the tumor tissue is one of the fundamental causes of
high recurrence and metastasis of cervical cancer. As a highly active vascular
endothelial growth factor, endostatin has been shown to inhibit
the growth of a variety of solid tumors in preclinical studies [4, 5, 6], but its
insolubility, instability, short half-life, and high synthetic cost severely
limit its clinical application. Endostatin is a C-terminal fragment of a XVIII
molecule with a molecular weight of 20 kDa and the most effective endogenous
angiogenesis inhibitor currently available. Endostar was developed by Chinese
scientists applying Professor Folkman’s research to improve the solubility and
stability of recombinant human endostatin. Xu et al. [7] reported that
Endostar inhibits angiogenesis by downregulating the Wnt/
Additionally, the combination of anti-angiogenesis treatment with radiotherapy
has found a broad range of clinical applications for cervical cancer treatment.
Ke et al. [9] discovered that when combined with radiotherapy and
chemotherapy, recombinant human endothelin can improve the short-term efficacy of
advanced cervical cancer without causing serious adverse effects. In addition,
Jia et al. [10] found that the most significant inhibition of HeLa tumor
growth and lymph node metastasis was observed with Endostar plus concurrent
chemoradiotherapy (CCRT) compared with CCRT only, Endostar only, or a control
group. Endostar was also found to inhibit the expression of vascular endothelial
growth factor (VEGF) and hypoxia-inducible factor 1 alpha (HIF-1
Conversely, radiotherapy has been shown in studies to stimulate tumor
angiogenesis, resulting in increased malignancy of tumor cells, which in turn
promotes tumor metastasis and recurrence [12]. Tumor cells secrete a variety of
cytokines in response to radiation stimulation, including VEGF and transforming
growth factor
In the present study, through cell proliferation and plate cloning experiments, we investigated the inhibitory effects of Endostar and radiotherapy on HeLa and SiHa cervical cancer cells as well as human umbilical vein endothelial cells (HUVECs). Additionally, flow cytometry and immunofluorescence were used to assess the effect of Endostar and radiotherapy on apoptosis, cell cycle distribution, and H2A histone family member X (H2AX) expression. Finally, we investigated the synergistic effect of Endostar with radiotherapy using a cervical cancer transplanted tumor model in nude mice. Our findings provide experimental evidence and a theoretical basis for the application of Endostar in anti-cervical cancer treatment in the future.
Human cervical cancer cell lines HeLa (http://www.mjswkj.cn/atcc-list.asp?id=253&lx=small&anid=2&nid=12) and SiHa (http://www.mjswkj.cn/atcc-list.asp?id=2604&lx=small&anid=2&nid=12) were obtained from the Cell Bank of Type Culture Collection of Chinese Academy of Sciences (Shanghai, China). HUVECs (http://www.mjswkj.cn/atcc-list.asp?id=2876&lx=small&anid=2&nid=12) were purchased from MINGJING Biology. Co. Ltd. (Shanghai, China). All cells were cultured in appropriate conditions according to the suppliers’ instructions.
Recombinant human endostatin, Endostar, was provided by Simcere Pharmaceutical
Co., Ltd. (Nanjing, China). For in vitro assays, experimental groups
were set up as follows: control group, radiotherapy (RT) group, Endostar (E)
group, and E+RT group. Cell proliferation rates were determined by Cell Counting
Kit-8 (CCK8) assay (Beyotime Biotechnology, Shanghai, China) following the
manufacturer’s instructions, and six replicates were tested for each group.
Specifically, cells at a density of 4
After HeLa and SiHa cells had adhered to six-well plates at the density of about
500 cells/well, culture medium containing Endostar was added for incubation for 1
hour, and then X-ray irradiation of 0, 2, 4, 6, or 8 Gy was administered once,
followed by a 13-day incubation period with medium exchanged every 5 days. When
cell colonies were visible under the microscope, they were fixed in 4%
paraformaldehyde for 20 minutes and stained with crystal violet for 15 minutes
for counting the cell colonies (
A six-well plate was seeded with a well-growing single-cell suspension to achieve the optimal cell density. After the cells reached 80% confluency, Endostar and/or 12 Gy X-ray was administered for 24 hours. Cells were harvested and stained with propidium iodide (PI) after pretreatment with RNase A for cell cycle analysis. The Annexin-AbflourTM647 Apoptosis Detection Kit (Abbkine Scientific, Beijing, China) was used for cell apoptosis detection according to the manufacturer’s instructions. The FACS Canto II instrument (BD Biosciences, San Diego, CA, USA) was used to perform cytometry experiments, and the software FlowJo (Version 10.0.7) (Ashland, OR) was used to analyze cell cycle and apoptosis.
After addition of about 75
Briefly, after fixation with 4% paraformaldehyde and permeabilization with
0.1–0.5% triton X-100, cells were incubated with primary antibody against
Four- to six-week-old female BALB/C nude mice with a bodyweight of 18–22 g were
obtained from the Beijing Weitong Lihua Experimental Animal Technology Co., Ltd
(Beijing, China). They were bred and housed in specific pathogen-free (SPF)
conditions for this study. For each mouse, 100
All data were analyzed using SPSS 18.0 software (IBM, Armonk, NY, USA). Measured
data are expressed as mean
The CCK-8 assay was used to determine the effect of different concentrations of
Endostar on the proliferation of HeLa cells, SiHa cells, and HUVECs after
treatment for 24, 48 and 72 hours. As shown in Fig. 1C, at each time point,
Endostar inhibited the growth of HUVECs beginning at a concentration of 200
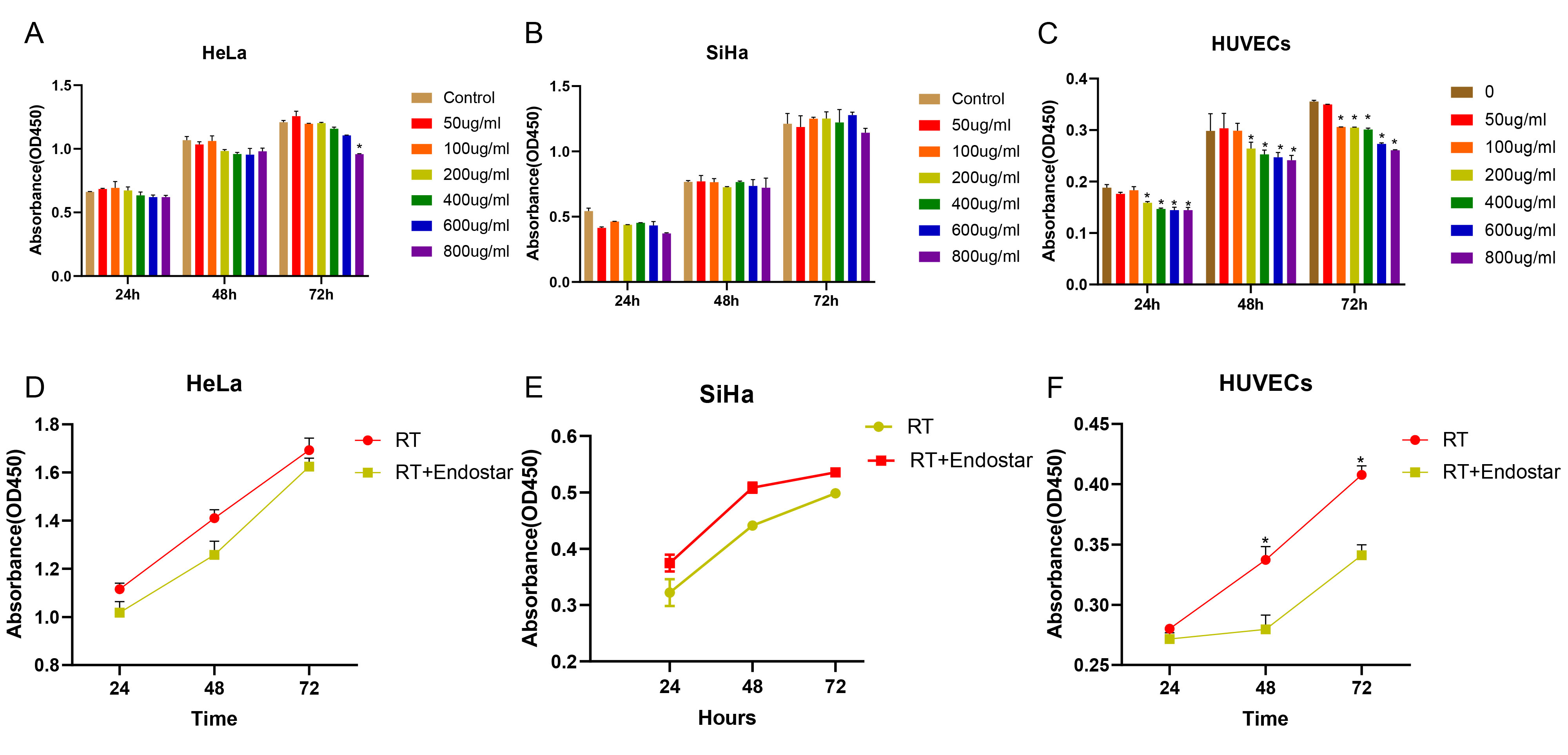
Endostar and irradiation synergistically inhibited the
proliferation of endothelial cells but not cervical cancer cells. HeLa cells
(A), SiHa cells (B) and HUVECs (C) were treated with the different concentrations
of Endostar for 24, 48, or 72 hours. HeLa cells (D), SiHa cells (F) and HUVECs
(F) were further treated with 200
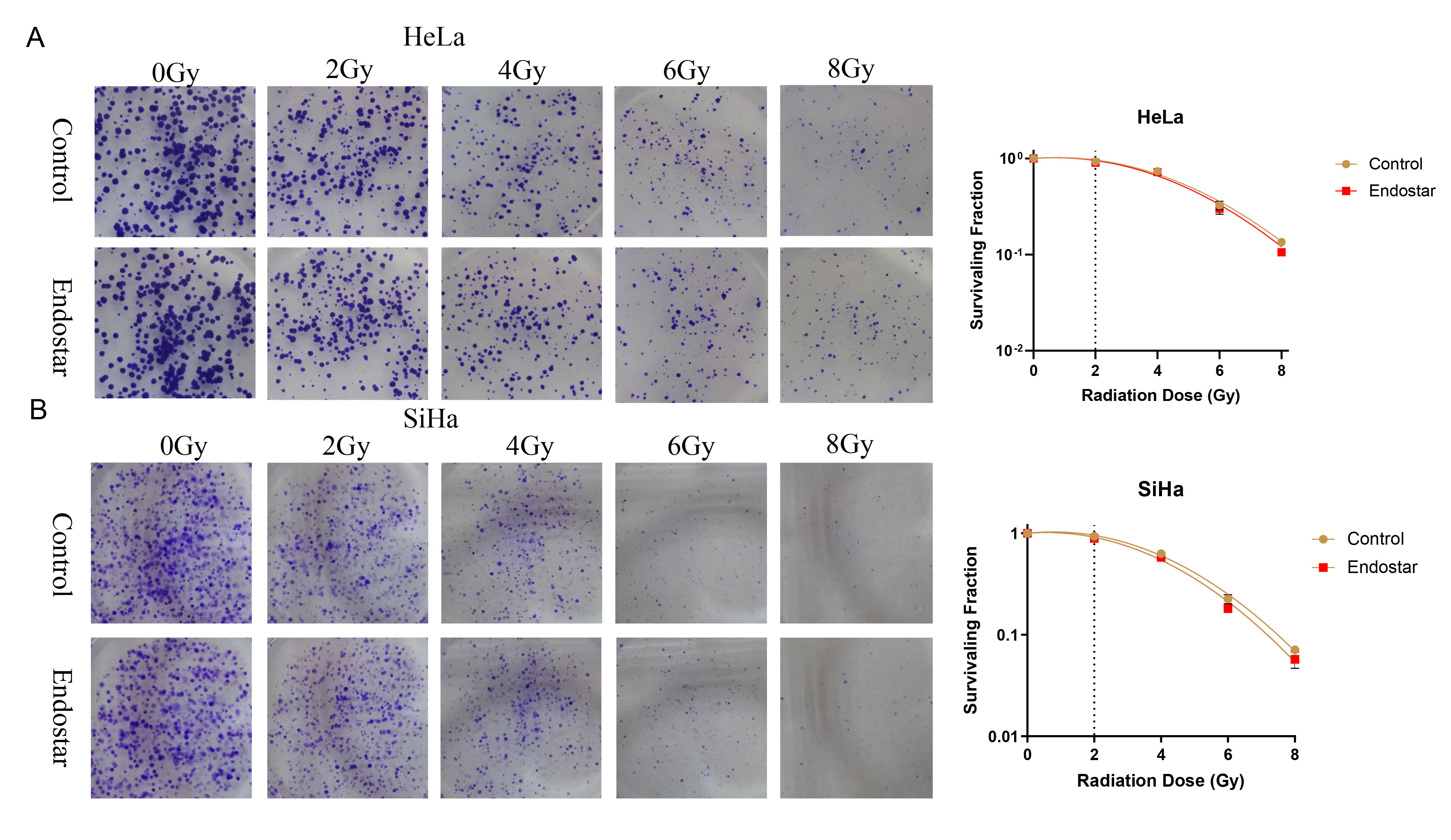
The effects of Endostar and its combination with radiotherapy on the clonogenic ability of HeLa cells (A) and SiHa cells (B). In HeLa or SiHa cells treated with the same RT dose, the addition of Endostar did not significantly change the cell survival score, indicating that Endostar had no measurable impact on the radiotherapy sensitivity of cervical cancer cells.
Annexin-V/PI double staining was performed to assess treatment-induced
apoptosis. The results indicated that compared to those of the untreated groups,
the apoptosis rates of HeLa cells (Fig. 3A), SiHa cells (Fig. 3B), and HUVECs
(Fig. 3C) were significantly higher in the RT group (p
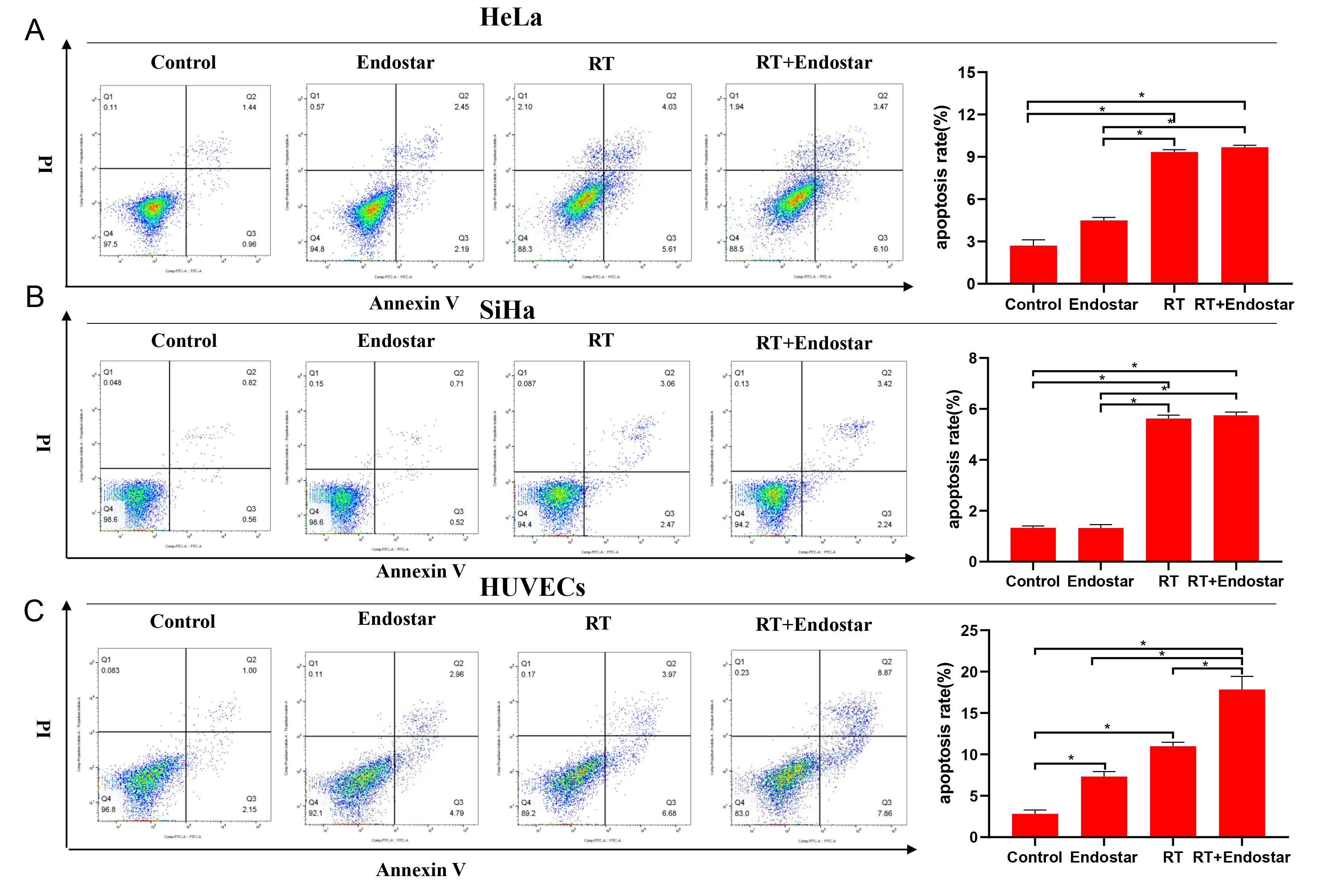
Endostar and radiotherapy synergistically induced apoptosis of
endothelial cells. Flow cytometry indicated that compared to
those in the untreated groups, the apoptosis rates of HeLa cells (A), SiHa cells
(B), and HUVECs (C) were significantly higher in the RT group (p
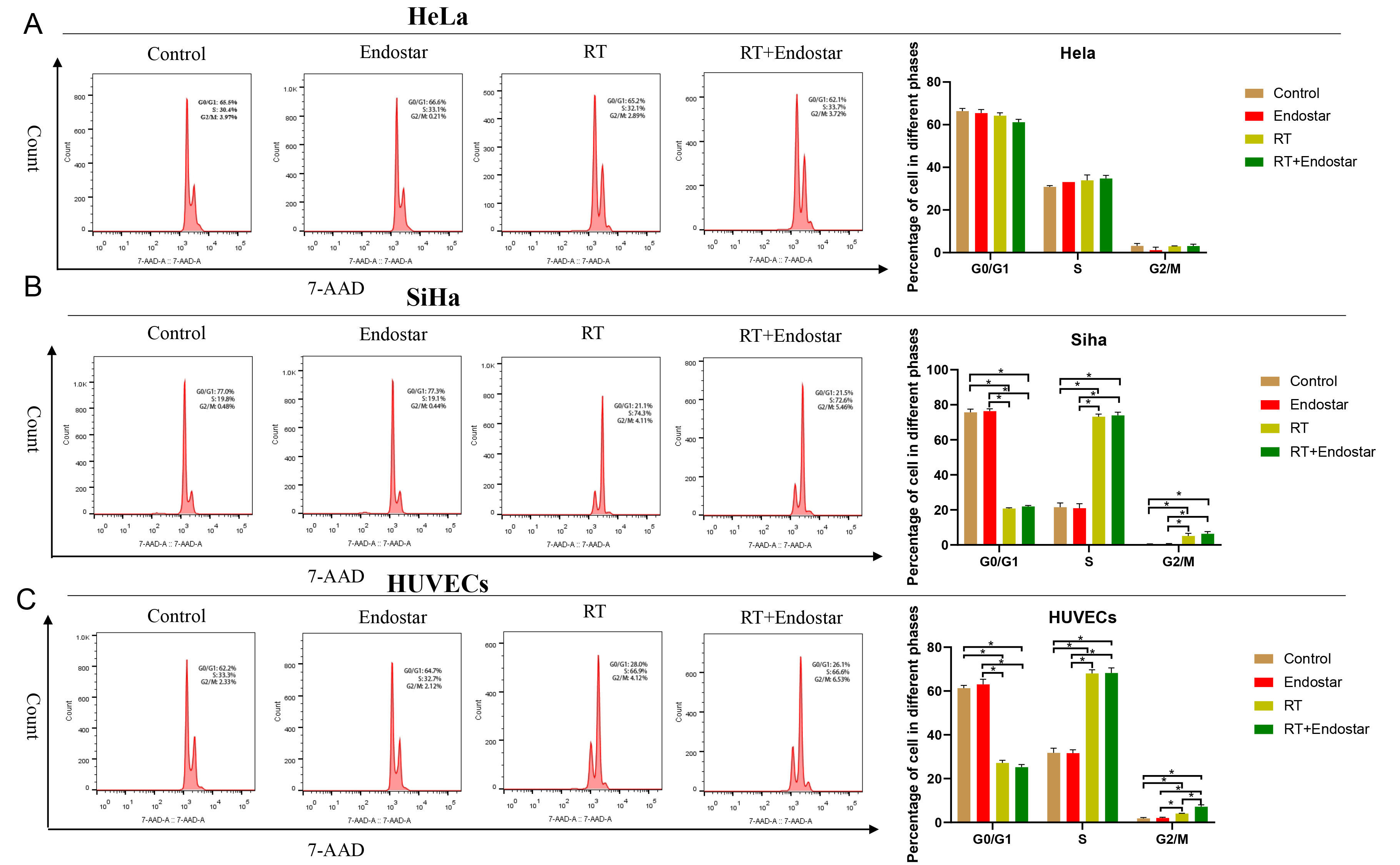
Endostar and radiotherapy synergistically induced cell
cycle arrest of endothelial cells. Neither the Endostar+RT group nor the RT
monotherapy group showed a significant difference in the proportions of HeLa (A)
or SiHa cells (B) in each cell cycle phase. (C) Compared with that in the control
group, the ratio of HUVECs in G0/G1 phase in the Endostar+RT group or RT
monotherapy group was significantly reduced, while the proportions of cells in S
and G2/M phases were increased significantly (p
Angiogenesis is characterized by the formation of capillary tubes and the
sprouting of new capillaries during solid tumor growth. To evaluate the
therapeutic effects of Endostar and radiotherapy on this reorganization stage
during angiogenesis, an in vitro tube formation assay was performed. As
displayed in Fig. 5, microscopic observation showed that the arrangement of the
tubes in the negative control group was essentially complete after 3 hours, while
that in the treatment group was incomplete and had a sparse network. The
quantitative results also showed that compared with that in the control group,
the capillary area was significantly inhibited in each treatment group at each
time point. Additionally, the inhibition rate was noticeably higher in the
Endostar+RT combination group than in the corresponding monotherapy groups
(p
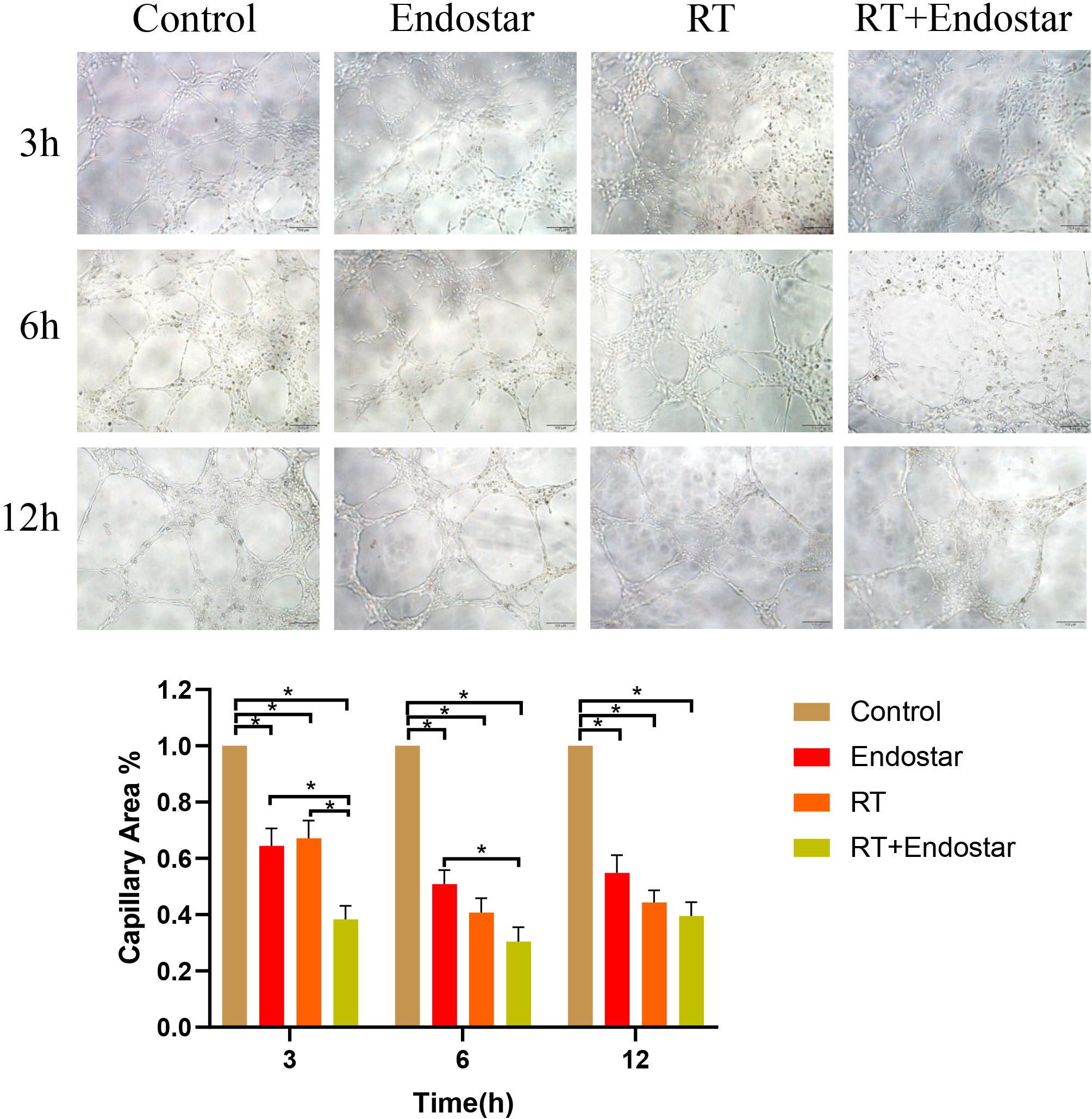
Endostar combined with radiotherapy inhibited tube formation by
endothelial cells in vitro. Microscopic observation showed that the
arrangement of the tubes was incomplete and had a sparse network in the treatment
group. The quantitative results also showed capillary area was significantly
reduced in each treatment group at each time point. Additionally, the inhibition
rate was noticeably higher in the Endostar+RT group than in each monotherapy
group (p
By evaluating the
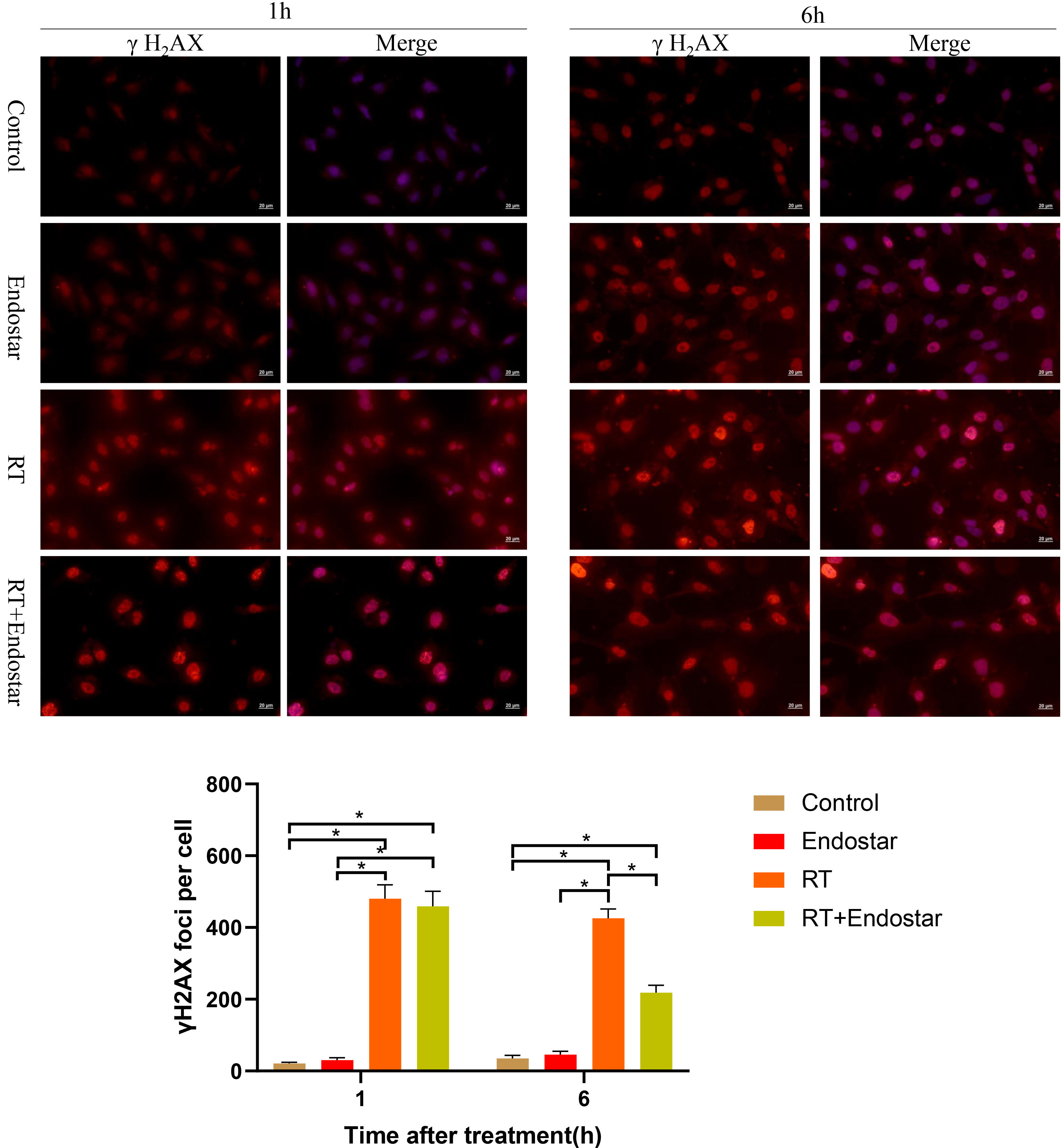
Endostar inhibited RT-induced DNA damage repair in HUVECs
in vitro. One hour after treatment, the expression of
The above results led us to hypothesize that Endostar may enhance HUVEC
radiosensitivity by inhibiting DNA repair via the VEGF receptor (VEGFR) pathway
(Fig. 7). To test this hypothesis, we examined the expression regulation of VEGFR
and its downstream signaling molecules in HUVECs treated with gradient
concentrations of Endostar in combination with RT. Western blotting analysis
revealed that, in comparison to RT monotherapy, Endostar+RT significantly
inhibited the phosphorylation of VEGFR, PI3K, AKT, and DNA-PKcs in HUVECs
(p
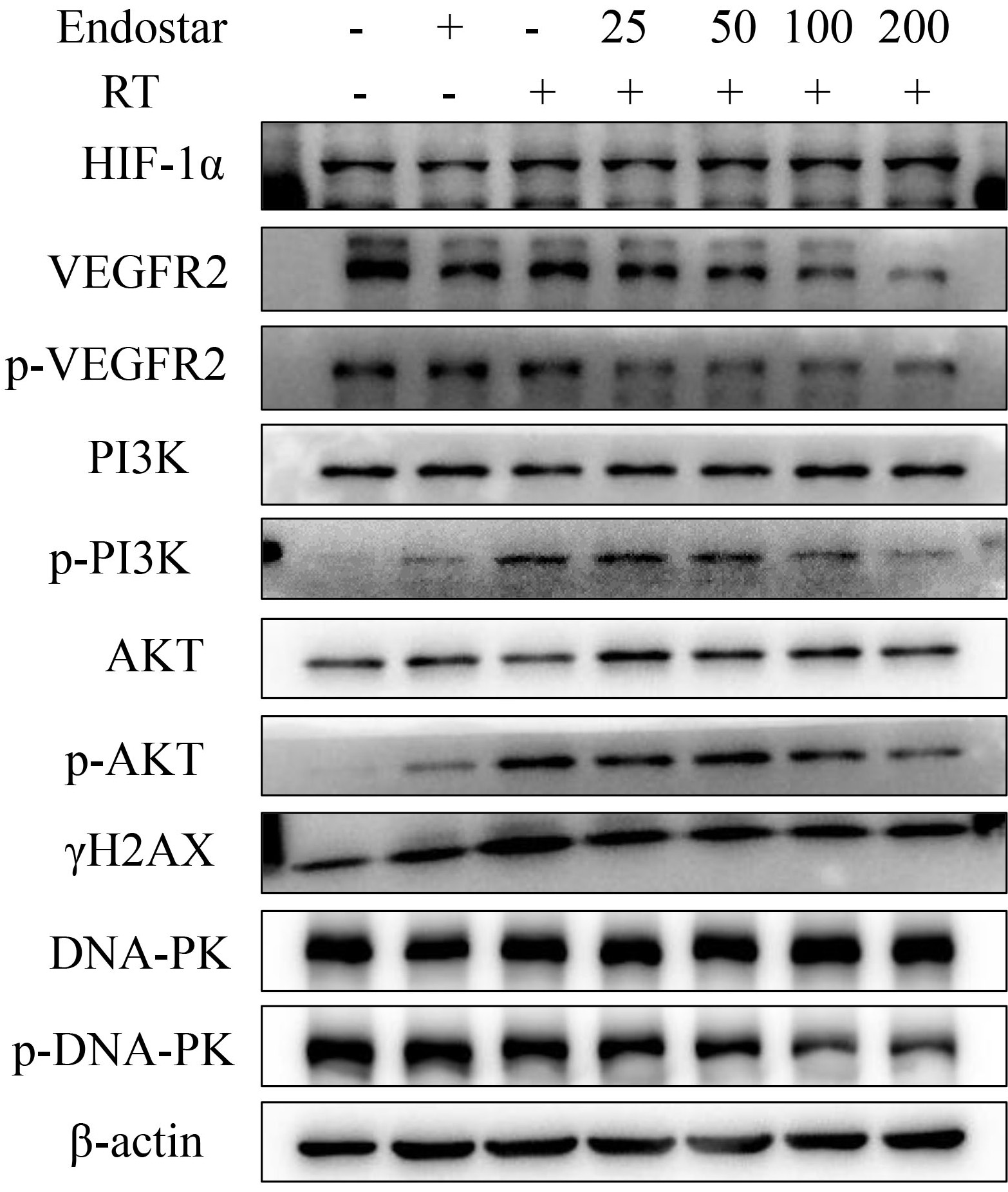
The effect of Endostar on
HIF-1
To study the effect of Endostar on the growth of subcutaneously transplanted
tumors of HeLa cells, we started daily tail vein injection of Endostar when the
tumor volume reached 70–150 mm
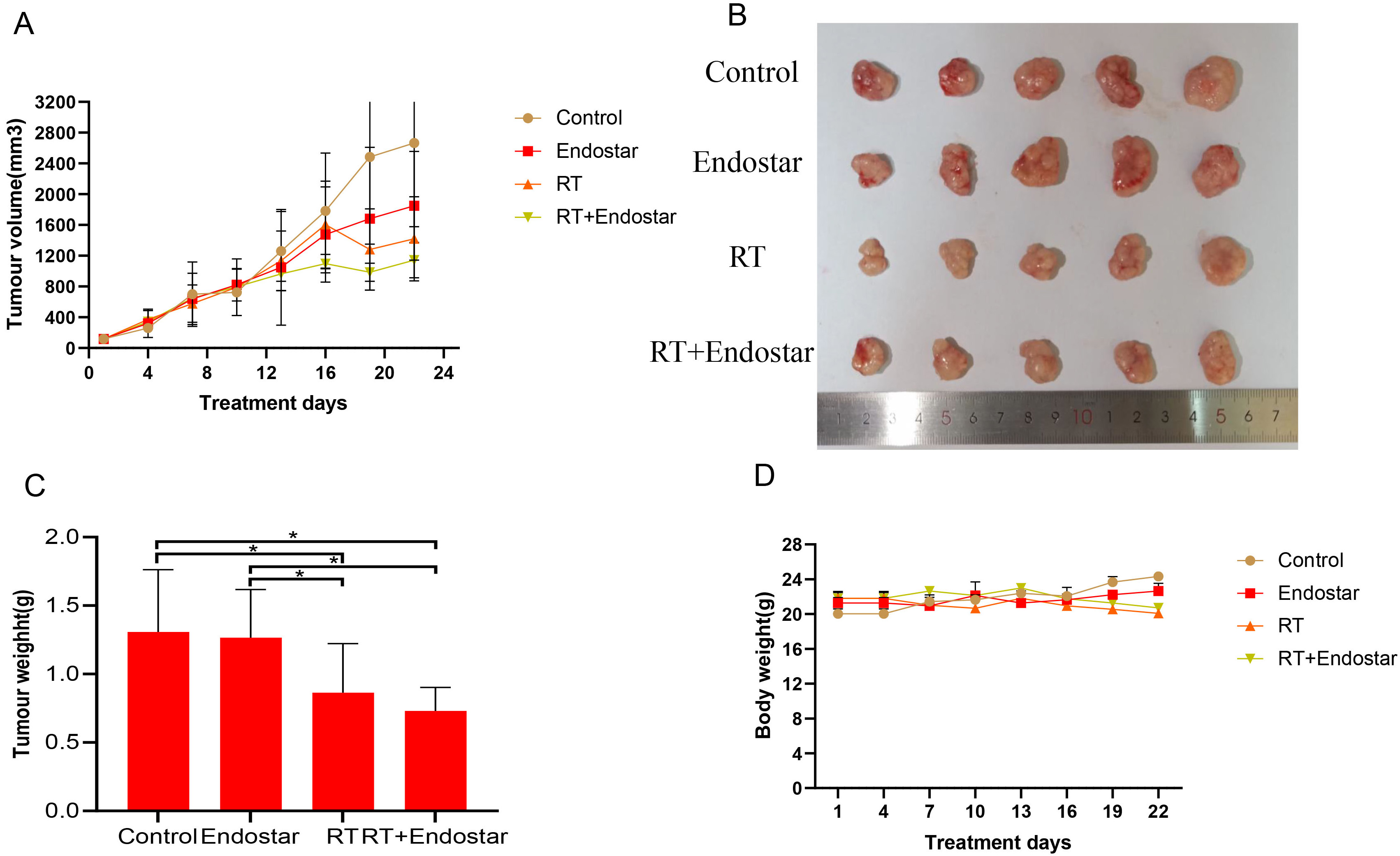
Endostar cannot directly inhibit cervical tumor growth
in vivo. (A,B) Tumor volume did not differ significantly
between the groups prior to radiotherapy. Tumor size in the RT monotherapy and
Endostar+RT groups was significantly decreased on the fourth day following
radiotherapy (p
The density of microvessels in xenografted tumors was quantified using
immunohistochemical staining for the endothelial cell marker CD31. After
treatment, the tumor microvessel density was significantly decreased in all three
treatment groups compared with that in the control group (p
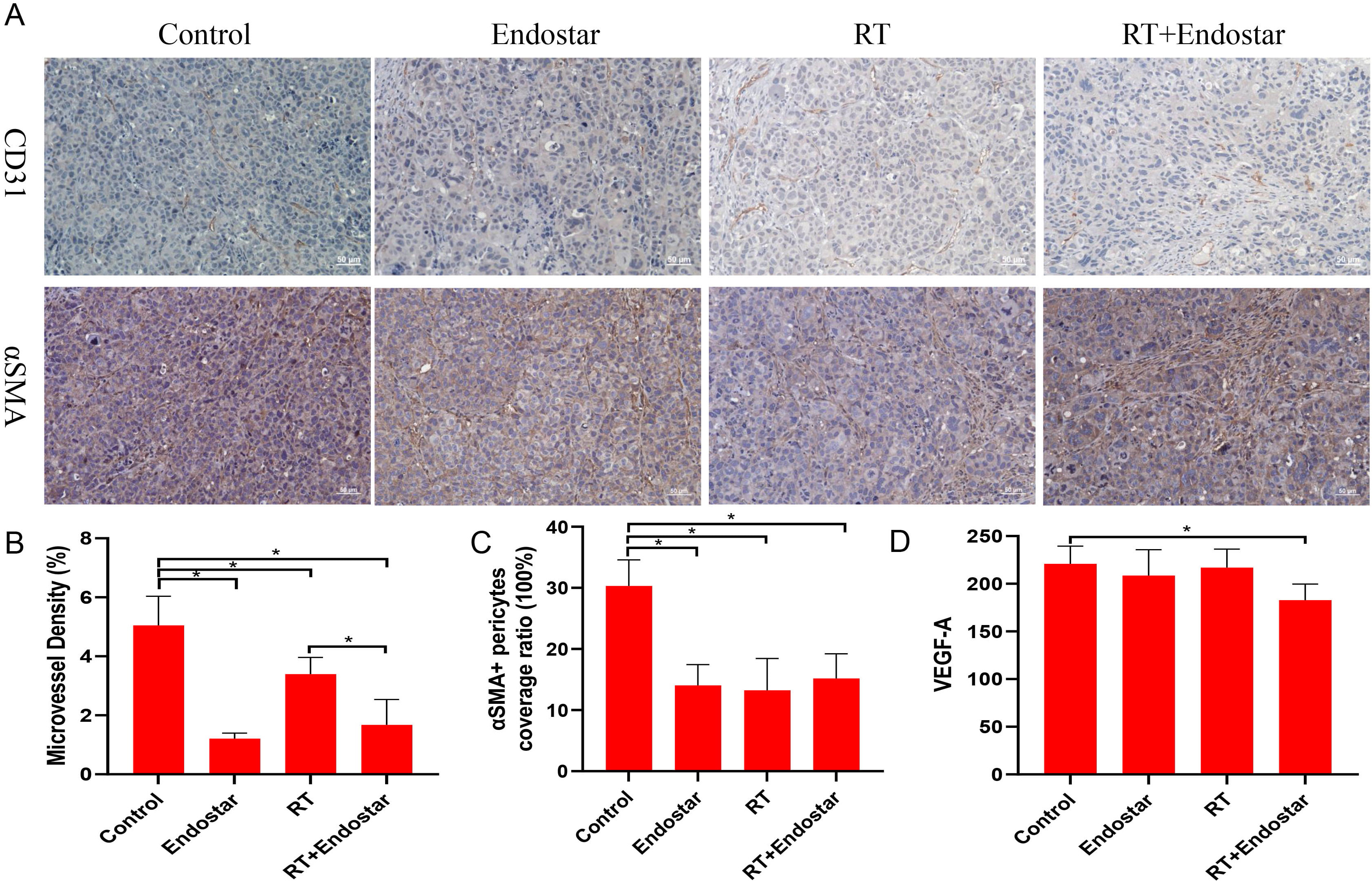
Anti-angiogenic effects of Endostar and radiotherapy in
tumor xenografts. (A,B) The tumor microvessel density was significantly
decreased in all three treatment groups (p
Recent basic research on recombinant endostatin has revealed that it has the ability to inhibit endothelial cell migration and tubule formation, as well as to block tumor nutrient supply, thereby inhibiting tumor proliferation and metastasis [16]. Additionally, studies have demonstrated that recombinant human endostatin can induce apoptosis in tumor cells and vascular endothelial cells [17] as well as inhibit the G2/M phase transition, thereby affecting tumor radiotherapy sensitivity [18, 19]. In the present study, we discovered that Endostar in gradient concentrations inhibited HUVEC proliferation without impairing HeLa or SiHa growth. Additionally, Endostar and its combination with radiotherapy inhibited HUVEC growth but had no apparent synergistic effect on cervical cancer cells. Flow cytometric results revealed that Endostar promoted apoptosis and G2/M phase arrest in HUVECs compared to control treatment, which was further enhanced by combination with radiotherapy, but had no effect on HeLa or SiHa cells. Tubule formation experiments demonstrated that both Endostar and radiotherapy treatments could inhibit tubule formation by HUVECs, and the effect was further strengthened by combination of these therapies.
Endostar has been shown in signaling pathway research to inhibit tumor
angiogenesis by down-regulating the expression of VEGF and VEGFR [8]. Endostar
can also inhibit other key signaling pathways, including HIF-1
Recent research has discovered a link between the inhibition of angiogenesis and
DNA damage repair. Raffaella et al. [21] discovered that siRNA-mediated
silencing of AKT decreased DNA-PKcs (Thr2609) phosphorylation, indicating that
AKT regulates DNA-PKcs expression [22]. Additionally, studies have shown that
when exposed to radiation, epidermal growth factor receptor (EGFR) translocates
to the nucleus to regulate DNA-PKcs activity and promote double-strand break
repair [23], and that VEGF influences radiation-induced changes in EGFR
subcellular localization [24]. Endostatin was found to promote DNA damage in
colon cancer cells by interacting with DNA-PKcs [25]. Additionally, by inhibiting
the DNA-PKcs signaling pathway, bevacizumab was found to improve the radiotherapy
sensitivity of non-small cell lung cancer cells [26]. Anti-VEGF therapy has been
shown to promote radiation-induced apoptosis in glioblastoma cells by inhibiting
ataxia telangiectasia mutated (ATM) and DNA-PKcs activity [27]. In the present
study, we discovered no significant difference in
It was previously discovered that treatment with recombinant human endostatin prior to exposure to 8 Gy radiotherapy significantly inhibits tumor growth in an oral squamous cell carcinoma xenograft tumor model by inhibiting HIF-1 and VEGF expression in the body, thereby inducing remodeling the vascular system and improving hypoxia within the tumor [28]. Wen et al. [29] discovered that recombinant human endostatin combined with radiotherapy can improve the radiosensitivity of human nasopharyngeal carcinoma and lung adenocarcinoma transplanted tumors by increasing tumor cell and endothelial cell apoptosis, decreasing tumor hypoxia, and regulating vascular growth factor expression. Additionally, Jiang et al. [30] demonstrated that combining recombinant human endostatin with radiotherapy can effectively delay tumor growth, which may be related to improved tumor hypoxia and radiotherapy-induced angiogenesis inhibition. Winkler [31] discovered that inhibiting VEGFR2 expression can result in a “normalization window”, or a period during which radiotherapy has the greatest therapeutic effect. It is characterized by an increase in tumor oxygenation, which is thought to be the basis for improved tumor radioresponsiveness. Other studies have also confirmed that recombinant human endostatin can normalize tumor blood vessels in a short period of time [32, 33]. In our xenograft tumor study, Endostar treatment had no effect on tumor volume based on comparison with the control group. Although compared with that in the radiotherapy treatment group, the tumor volume in the combination treatment group was slightly reduced, the difference was not statistically significant. This outcome may be explained by a failure to administer radiotherapy during the normalization window, as well as by the mode, route, and dosage of radiotherapy. Additional research is required to determine the optimal treatment. Additionally, serum VEGF-A levels were significantly lower in the combined group compared to the control group, but not in the single treatment groups, indicating that Endostar can inhibit VEGF-A expression in combination with radiotherapy.
Microvessel density is a well-regarded indicator for determining the blood
vessel density in tumor tissues. CD31 is a vasculature-specific marker expressed
by proliferating endothelial cells in the vasculature. Pericytes support
endothelial cells, and
This study has several imitations. First, this study used a single high-dose irradiation because it has a greater biological effect than segmented irradiation and can shorten the research period for smaller tumors. However, in clinical practice radiotherapy is divided into multiple doses. Second, there were only five mice in each group, making it difficult to determine statistical significance. Third, this study did not investigate hypoxia within tumor tissues or DNA damage repair in endothelial cells in vivo. Furthermore, orthotopic models and survival studies will be more useful for predicting the effectiveness of applying Endostar in clinical treatment than the method used in the present study. We will increase the sample size and improve the related in vivo experiments in future studies.
Endostar can synergize with irradiation to inhibit DNA damage repair and cell proliferation of endothelial cells through the VEGF pathway, thereby reducing the microvessel density in tumor tissues. Our findings provide experimental evidence and a theoretical basis for the application of Endostar in anti-cervical cancer treatment in the future.
The datasets generated and analyzed during the current study are available from the corresponding author on reasonable request.
ZX, XZ, YL and QZ conceived and designed research; ZX, XZ, HS, WL, YD, LX and HZ collected data and conducted research; ZX, YL and XZ analyzed and interpreted data; ZX and YL wrote the initial paper; YL and QZ revised the paper; YL had primary responsibility for final content. All authors read and approved the final manuscript.
The Animal Care and Use Committee approved all experimental procedures for making every effort to minimize animal suffering. All procedures were performed following the relevant guidelines and regulations.
Not applicable.
This study was funded by the China International Medical Foundation (CIMFz-2016-06-19413) and the Major Program of the Anhui Natural Science Foundation (KJ2016A754).
The authors declare no conflict of interest.
Publisher’s Note: IMR Press stays neutral with regard to jurisdictional claims in published maps and institutional affiliations.