†These authors contributed equally.
Academic Editor: Sang Heui Seo
Coronavirus disease 2019 (COVID-19), which broke out at the end of 2019, is a global pandemic and seriously threatens human health. Vaccination is the most effective way to prevent and control COVID-19. At present, more than 13 COVID-19 vaccines have been urgently authorized for use, but the emergence of severe acute respiratory syndrome coronavirus 2 (SARS-CoV-2) variants has brought unprecedented challenges to the protective efficiency of these COVID-19 vaccines. In particular, the recent emergence of Delta and Omicron variants, which are rapidly spreading worldwide, may bring many challenges to the medical systems. Interestingly, previous studies have shown that the Bacillus Calmette-Guerin (BCG) vaccine used to prevent tuberculosis can induce non-specific trained immunity, protecting against infectious diseases caused by respiratory viruses. Therefore, there is a hypothesis that BCG plays an essential role in reducing the incidence, severity, hospitalization, and mortality of COVID-19 and enhancing the protection efficiency of the COVID-19 vaccine. To confirm this hypothesis, 56 clinical trials have been conducted globally to assess BCG’s protective effectiveness against COVID-19 infection. Herein, this review discussed the trained immunity induced by BCG and its underlying mechanisms and summarised BCG’s latest research progress in preventing COVID-19, especially the ongoing clinical trials. We hope this review will provide new strategies for fighting against COVID-19.
Coronavirus disease 2019 (COVID-19) is a respiratory infectious disease caused by severe acute respiratory syndrome coronavirus 2 (SARS-CoV-2). Since 2019, COVID-19 has had an significant impact on the global economy, politics, and health system. As of April 21, 2022, the number of confirmed cases globally has reached 505,035,1854, including 6,210,719 deaths (https://covid19.who.int). Vaccination is the best way to prevent and control COVID-19. At present, at least 13 COVID-19 vaccines have been urgently authorized, including (1) inactivated vaccines, BBV152 (Covaxin, India) [1], BBIBP-CorV (Sinopharm, China) [1, 2], and Sinovac (CoronaVac, China) [1, 2]; (2) Viral vector vaccine, ChAdOx1 nCoV-19 (AstraZeneca, UK) [2, 3], Sputnik V (Gamaleya, Russia) [2], CanSino’Ad5-nCoV (China) [3], Ad26.COV2.S (Janssen, USA) [2]; (3) Nucleic acid vaccine, BNT162b1/BNT162b2 (Pfizer-BioNtech, USA) [2], mRNA1273 (Modern, USA) [2], CureVac (Germany) [4]; (4) Subunit vaccine, NVX-CoV2373 (Novavax, USA) [5], EpiVacCorona (Russia) [2], and ZF2001 (Anhui Zhifei Longcom, China) [6, 7]. As of April 17, 2022, a total of 11,324,805,837 doses of COVID-19 vaccine have been vaccinated worldwide (https://covid19.who.int).
Although the above vaccines approved for emergency use in COVID-19 prevention
have sound protective effects, they also face many challenges [3, 8]. First, the
ongoing emergence of SARS-CoV-2 variants poses a continuing threat to the
efficacy of COVID-19 vaccines, such as immune escape, reduced protection
efficiency and antibody neutralization ability [9]. Second, the accessibility of
COVID-19 vaccines varies among different countries, which affects the balanced
global supply of COVID-19 vaccines. Third, the acceptance of COVID-19 vaccines
has been decreased by the shortened process of COVID-19 vaccine development and
production. Finally, most COVID-19 vaccines require high transportation
conditions, especially the mRNA vaccines should be stored at –80
Previous studies have found that the trained immunity induced by the Bacillus Calmette-Guerin (BCG) vaccine might have a protective effect on infectious diseases caused by respiratory viruses, including respiratory syncytial virus (RSV), influenza viruses, and SARS-CoV-2 [3, 10, 11, 12]. To further explore the impact of BCG vaccination on COVID-19 morbidity, severity, hospitalization, mortality, and the protection efficiency of the COVID-19 vaccines, this review focuses on the trained immunity induced by the BCG vaccine and its underlying mechanisms, highlights the latest research progress on BCG effect on the prevention of COVID-19, and provides potential solutions for many challenges faced by COVID-19 vaccines.
SARS-CoV-2 is an RNA virus belonging to coronaviridae, having a high mutation
rate [13]. During the COVID-19 pandemic, a growing number of SARS-CoV-2 variants
emerged and spread rapidly worldwide
(https://www.who.int/activities/tracking-SARS-CoV-2-variants/tracking-SARS-CoV-2-variants,
Table 1 [5, 6, 14, 15, 16, 17, 18, 19, 20, 21, 22, 23, 24, 25, 26, 27, 28, 29, 30, 31, 32, 33, 34, 35, 36, 37, 38, 39, 40, 41, 42, 43, 44, 45, 46, 47, 48, 49, 50, 51]). As of March 24, 2022, the World Health Organization (WHO)
defined five SARS-CoV-2 variants as Variants of Concern (VOCs) (Fig. 1),
including two currently circulating VOCs (Table 1), Delta (B.1.617.2) and Omicron
(B.1.1.529), and three previously circulating VOCs such as Alpha (B.1.1.7), Beta
(B.1.351), and Gamma (P.1). Furthermore, eight SARS-CoV-2 variants are also
listed as previously circulating Variants of Interest (VOIs), including Lambda
(C.37) and M
Type | WHO label Variant/lineage | Origin | Mutations in spike protein* | Influence | Reference |
VOC* | Alpha( |
Sep-2020 United Kingdom | del69/70, del144/144, N501Y, A570D, D614G, P681H, T716I, S982A, D1118H | (1) N501Y mutation can enhance the affinity between Alpha variant S protein and human host cell ACE2 receptor, have relative resistance to neutralising antibodies and enhance virus transmission [16, 17]. | [14, 21] |
(2) The del144/144 mutation makes it difficult for the Alpha variant to be neutralised by most monoclonal antibodies against the N-terminal domain of S protein [18]. | |||||
(3)The effectiveness of the NVX-CoV2373 vaccine on the original variant of SARS-CoV-2 is 95.6%, and the efficacy on the Alpha variant is 85.6% [17]. | |||||
(4) The protection rate of the NVX-CoV2373 vaccine against SARS-CoV-2 infection was 89.7%, and the effectiveness against the Alpha variant was 86.3% [22]. | |||||
(5) The effectiveness of the mRNA-1273 vaccine on Alpha variant infection was 81.6% and 94.4%, respectively, in the third and fourth weeks after the first dose and 99.2% in the second week after the double dose [24]. | |||||
(6) The neutralisation effect of neutralising antibodies induced by two doses of the BNT162b2 vaccine on the Alpha variant was significantly increased, and the neutralisation titers were higher than 1:40 [25]. | |||||
(7) The effectiveness of one dose and two doses of BNT162b2 vaccine against the Alpha variant was 47.5% and 93.7%; The efficacy of one dose and two doses of ChAdOx1 nCoV-19 vaccine against the Alpha variant was 48.7% and 74.5%, respectively [26]. | |||||
Beta( |
May-2020 South Africa | D80A, D215G, K417N, E484K, N501Y, D614G, A701V | (1) E484k mutation can reduce the sensitivity of the SARS-CoV-2 virus to convalescent serum and vaccine-induced neutralising antibodies [27, 28]. | [5, 6, 19, 22, 23, 24, 25, 26, 27, 28] | |
(2) The infectivity of the Beta variant in Calu-3 cells was enhanced; the three mutations on S protein (E484k, K417N/T, N501Y) significantly increased the ability of infected mice to overexpress ACE2 cells [28]. These mutations increased the propagation rate of the Beta variant by 50% [30]. | |||||
(3) The effectiveness of the mRNA-1273 vaccine against SARS-CoV-2 original strain infection was 94.1% [23]. | |||||
(4) The effectiveness of the mRNA-1273 vaccine against Beta variant infection was 47.9% and 73.7%, respectively, in the third and fourth week after the first dose and 96.4% in the second week after the double dose [24]. | |||||
(5) The effectiveness of NXV-CoV2373 vaccine for Beta variant was 51.0% (95% CI, 0.6 to 76.2) [5]. | |||||
(6) The efficacy of two doses of ChAdOx1 nCoV-19 vaccine against mild to moderate COVID-19 caused by Beta variant was 10.4% [32]. | |||||
(7) Compared with SARS-CoV-2 wild-type, the neutralisation titers of convalescent plasma and antiserum inoculated with mRNA vaccine to Beta variant decreased by about 10 and 6 times, respectively [6, 33, 34]. | |||||
(8) Compared with SARS-CoV-2 wild-type, the neutralisation titers of antibodies induced by ZF2001 and BBIBP-CorV vaccines to Beta variant decreased by 1.59 times and 1.56 times [6]. | |||||
Gamma( |
Nov-2020 Brazil | L18F, T20N, P26S, D138Y, R190S, K417T, E484K, N501Y, D614G, H655Y, T1027I, V1176F | (1) Compared with the SARS-CoV-2 wild type, the neutralisation of the Gamma variant by various monoclonal antibodies, convalescent plasma, and inoculated serum decreased or even lost [35]. | [20, 29, 30] | |
(2) The neutralisation titer of antibody-induced by BNT162b2 vaccine to Gamma variant was 3.3 times lower than that of Alpha variant [14]. | |||||
(3) The neutralisation effect of the BNT162b2 vaccine on the Gamma variant was significantly lower than that of the SARS-CoV-2 original strain [25]. | |||||
Delta(δ) B.1.617.2 | Oct-2020 India | T19R, E156G, del157/158, L452R, T478K, D614G, P681R, D950N | (1) L452R and P681R mutations may enhance the infection and proliferation of the virus [30]. L452R can make the Delta variant escape the attack of CD8+ T cells. Delta variant is an immune escape variant with more robust host cell entry and fusion ability [31]. | [21, 30, 31, 32, 33, 34, 35, 36] | |
(2) COVID-19 patients infected with the Delta variant had a higher risk of emergency admission or death and a more significant burden on the health system than the Alpha variant [32]. | |||||
(3) Early data from the UK showed that BNT162b2 and ChAdOx1 nCoV-19 vaccines had only 33% protection efficiency against the Delta variant after the first dose [33, 34]. | |||||
(4) The effectiveness of two doses of BNT162b2 vaccine on Alpha variant and Delta variant was 93.7% and 88%, respectively; The efficacy of two doses of ChAdOx1 nCoV-19 vaccine against Alpha variant and Delta variant was 74.5% and 67%, respectively [21]. | |||||
(5) Compared with the SARS-CoV-2 wild type, the neutralising antibody titer of the Delta variant decreased by 5.8 times, which was significantly higher than that of the Alpha variant (reduced by 2.6 times) and the Beta variant (reduced by 4.9 times [35]. | |||||
(6) Compared with G614 variant, the neutralisation titer of serum neutralising antibody induced by BNT162b2, mRNA-1273 and Ad26 COV2.S vaccine to Delta variant decreased by 3 times, 2 times and 12 times, respectively [36]. | |||||
Omicron(ο) B.1.1.529 | Nov-2021 South Africa | A67V, del69/70, T95I, G142D, del143/145, N211I, del212/212, G339D, S371L, S373P, S375F, Q493R, G496S, Q498R, N501Y, Y505H, T547K, D614G, H655Y, N679K, P681H, N764K, D796Y, N856K, Q954H, N969K, L981F | (1) D614G can increase the viral load of hathe master’s upper respiratory tract, which may be conducive to virus transmission [37]. | [14, 15, 37, 38, 39, 40, 41, 42, 43, 44, 45, 46] | |
(2) N501Y mutation can enhance the affinity between virus S protein and ACE2 and prevent neutralising antibodies [14, 15]. | |||||
(3) The Omicron variant has strong transmissibility and immune evasion, which can cause re-infection of COVID-19 patients infected with other variants of SARS-CoV-2 [38, 39]. | |||||
(4) Compared with the Delta variant, the disease severity and risk of death of patients infected with the Omicron variant were significantly reduced [40, 41]. The Omicron variant has a 6-fold higher risk of reinfection than the Delta variant [41]. | |||||
(5) Compared with the Delta variant, the Omicron variant grows 70 times faster in the human bronchus and 10 times slower in the lung [42]. | |||||
(6) The neutralizing effect of neutralising antibody induced by BNT162b1/BNT162b2 vaccine on Omicron variant decreased 41 times compared with SARS-CoV-2 wild type [43]. Compared with the Delta variant, the neutralising effect of the antibody induced by the BNT162b2 vaccine on the Omicron variant was reduced by 12–44 times [44]. | |||||
(7) Compared with the SARS-CoV-2 wild type, The neutralisation effect of BNT162b2, mRNA-1273 and Ad26 COV2.S vaccine on the Omicron variant were reduced by 7–45 times [45]. | |||||
(8) The efficacy of 2 and 3 doses of mRNA vaccine on the Omicron variant was 65% and 86%, respectively [46]. | |||||
VOI* | Lambda( |
Dec-2020 Peru | G75V, T76I, R246N, del247/253, L452Q, F490S, D614G, T859N | (1) L452Q enhances the infectivity of Lambda variant, and its affinity for ACE2, F490S can prevent virus neutralisation by vaccine-induced neutralising antibody and reduce the efficacy of the vaccine and monoclonal antibody [45]. | [47, 48, 49, 50] |
(2) Compared with the D614G strain, the Lambda variant increased the infection ability of LLC-MK2 and Calu-3 cells by 1.6 and 3.3 times, respectively; the neutralisation effect of convalescent serum on the Lambda variant is reduced by 1.3 times [48]. | |||||
(3) Compared with D614G strain, the neutralisation effect of neutralising antibodies induced by the CanSino’Ad5-nCoV vaccine, BNT162b2 vaccine and mRNA-1273 vaccine on Lambda variant decreased by 2.5 times [48], 3.0 times and 2.3 times [49], respectively. | |||||
(4) The neutralisation effect of antibody-induced by CoronaVac vaccine on Lambda variant was 3.05 times lower than that of SARS-CoV-2 wild type [50]. | |||||
M |
Jan-2021 Columbia | T95I, Y144S, Y145N, R346K, E484K, N501Y, D614G, P681H, D950N | (1) D614G mutation can increase the viral load of upper respiratory tract infected hamsters [37]. | [14, 15, 22, 37, 51] | |
(2) N501Y mutation on M | |||||
(3) E484k mutation can reduce the neutralisation titer of vaccine-induced neutralising antibody to SARS-CoV-2 virus [22]. | |||||
(4) M | |||||
(5) Compared with the wild-type SARS-CoV-2, the neutralisation titer of neutralising antibody induced by BNT162b2 vaccine on M | |||||
*: VOC: Variant of Concern, Variants with increased transmissibility, virulence,
and/or decreased diagnostic, therapeutic, or vaccine efficacy. VOI: Variant of
Interest, Variants with mutations suspected or confirmed to cause a change in
transmissibility, virulence, or diagnostic/therapeutic/vaccine efficacy, plus
community transmission, a cluster of cases, or detection in multiple countries. **: The data were obtained from the outbreak.info website (https://outbreak.info/). The inclusion threshold is 75%, and the data acquisition time is February 10, 2022. |
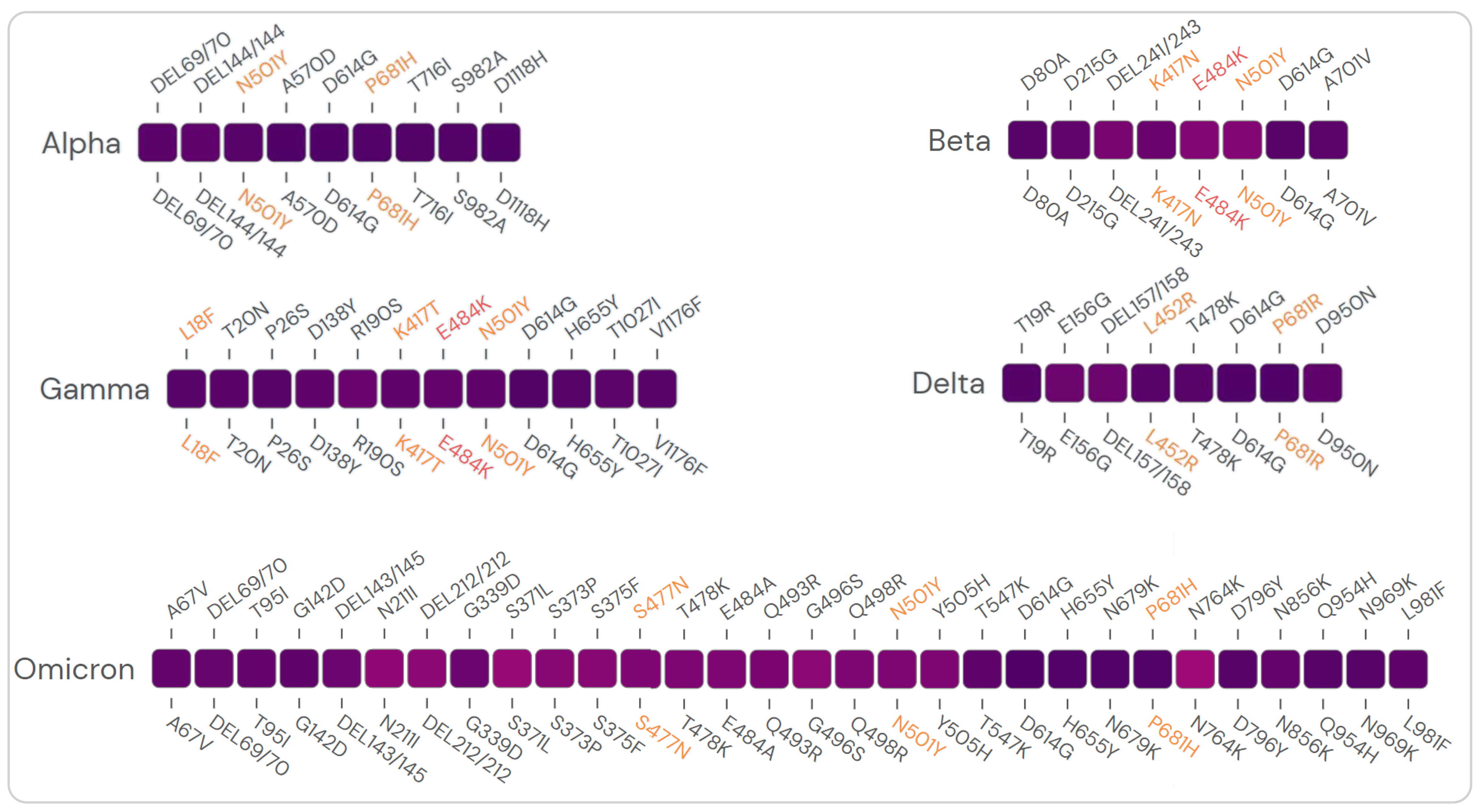
The prevalence of mutations in the S protein of the SARS-CoV-2
variants. Mutations in the S protein of five VOCs with
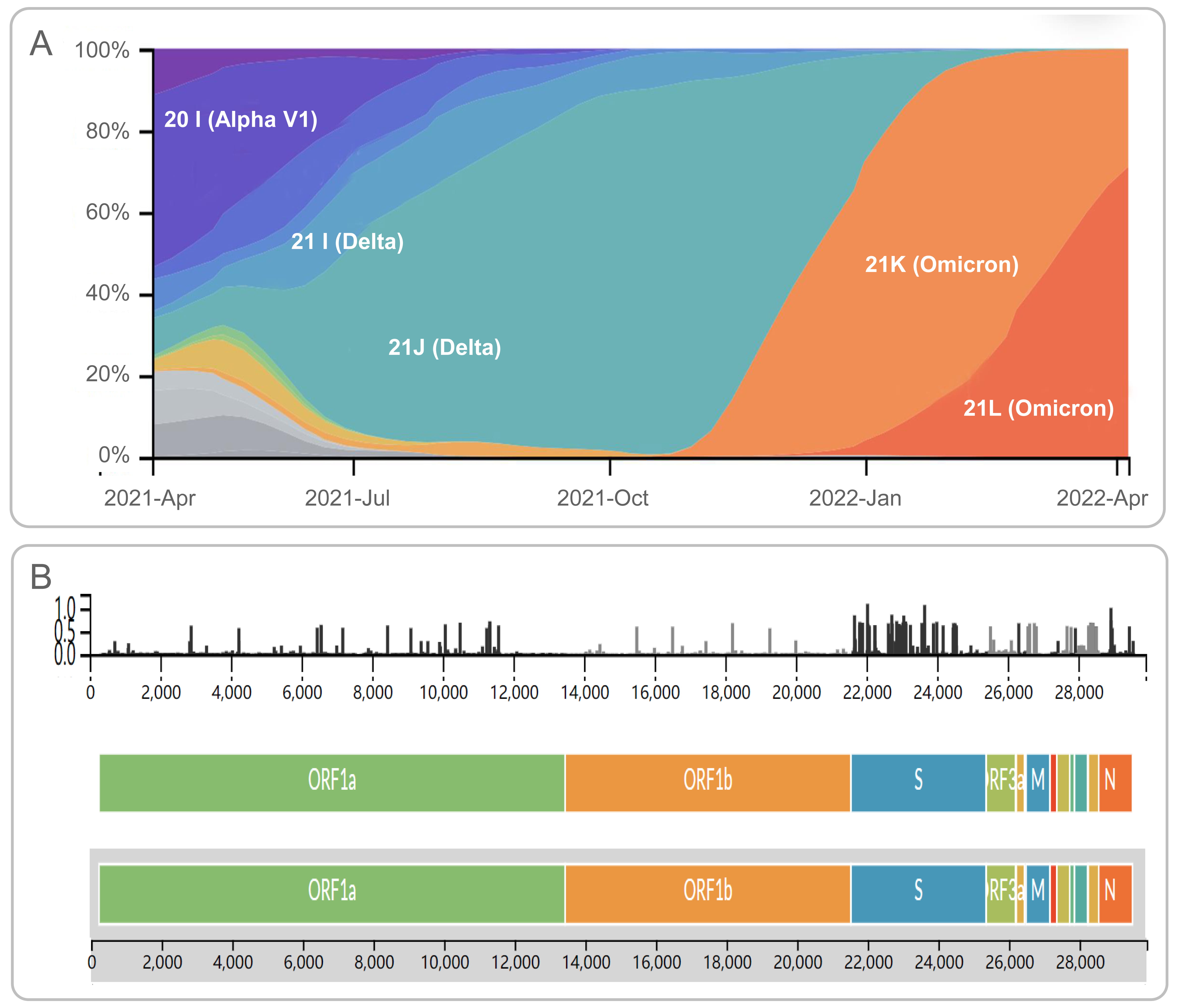
Frequencies and diversity of SARS-CoV-2 variants. (A) The dynamic change diagram of the frequencies of SARS-CoV-2 variants from February 2021 to April 2022. (B) The diversity of amino acid mutations in SARS-CoV-2 were shown by entropy. The data were obtained from https://nextstrain.org/ncov/gisaid/global, and the data acquisition time was April 16, 2022.
The primary mutation sites of these SARS-CoV-2 variants occurred on the Spike (S) protein, and most of the COVID-19 vaccines were designed based on the S protein of the original strain [14] (Fig. 2B). Many studies have shown that the transmission speed of these SARS-CoV-2 variants is enhanced, and the neutralizing ability and protection efficiency of neutralizing antibodies induced by the COVID-19 vaccine are reduced.
The Alpha variant was firstly discovered in the UK in September 2020. It spread
in Europe in the early stage and then became the dominant strain worldwide. As of
April 20, 2021, the global proportion of Alpha variant reached 40%. The S
protein of Alpha variant has nine characteristic mutations (characteristic
mutations for a lineage are defined as nonsynonymous substitutions or deletions
that occur in
Previous studies have evaluated the efficacy of COVID-19 vaccines against the
Alpha variant infection. (1) NVX-CoV2373 vaccine. A previous study indicated that
the NVX-CoV2373 vaccine was 95.6% effective against the original SARS-CoV-2
strain and 85.6% effective against the Alpha strain [17]. Similarly, in a phase
III clinical trial conducted in the UK, 15,187 adults aged 18–84 were randomly
divided into two groups vaccinated with 5
The Beta variant was first developed in South Africa in May 2020. Its S proteins have eight characteristic mutations: D80A, D215G, del241/243, K417N, E484K, N501Y, D614G, and A701V (https://outbreak.info/). It was found that E484k mutation can reduce the sensitivity of the SARS-CoV-2 virus to convalescent serum and vaccine-induced neutralizing antibodies [22, 23], resulting in a relative resistance. In the early recovery period of COVID-19 patients, the neutralization activity of plasma to Beta variant decreased significantly. Still, there was no significant change in the neutralization activity of the Alpha variant [16]. The infectivity of the Beta variant in Calu-3 cells (human lung adenocarcinoma cell line) was enhanced. Three mutations on S protein (E484k, K417N/T, and N501Y) significantly increased the ability of infected mice to overexpress ACE2 cells [23] and enhanced the affinity of virus S protein for ACE2 [55]. These mutations increased the propagation rate of the Beta variant by 50% [24].
In addition, studies have analyzed the impact of the Beta variant on the protective efficacy of COVID-19 vaccines. Preliminary data showed that the effectiveness of the mRNA-1273 vaccine against SARS-CoV-2 original strain infection was 94.1% [25]. With the emergence of the Beta variant, studies have shown that the neutralizing effect of neutralizing antibodies induced by the mRNA-1273 vaccine against the Beta variant is reduced [28]. A controlled study in adults conducted in Qatar showed that the effectiveness of the mRNA-1273 vaccine against Beta variant infection was 47.9% and 73.7%, respectively, in the third and fourth week after the first dose, and 96.4% in the second week after the double dose [19]. Therefore, it is suggested that two doses of the mRNA-1273 vaccine can effectively fight the Beta variant infection, and the second injection’s optimal vaccination time may vary according to different populations and regions, which needs to be studied and determined. A study on serum neutralization showed that compared with the original strain of SARS-CoV-2, the sera of people who received two doses of the BNT162b2 vaccine had a lower neutralization effect on the Beta variant, but the BNT162b2 vaccine still had a protective effect on the Beta variant [23]. According to previous reports, the NXV-CoV2373 vaccine was 95.6% effective against the primary strain of the SARS-CoV-2 strain, and the vaccine was 60.0% effective against the Beta variant [17]. Recently, a Phase II clinical trial in South Africa yielded similar results, and the analysis data showed that the effectiveness of the NXV-CoV2373 vaccine against the Beta variant was 51.0% (95% CI, 0.6 to 76.2) [5]. Therefore, it is suggested that the protective effect of the NXV-CoV2373 vaccine against the Beta variant is lower than its protective effect on SARS-CoV-2 original strain.
Furthermore, the previous studies also evaluated the protective efficacy of other COVID-19 vaccines against Beta variant infection. The effectiveness of two doses of ChAdOx1 nCoV-19 vaccine against mild to moderate COVID-19 caused by Beta variant was 10.4% [26], and the neutralization titers of antibodies induced by ZF2001 and BBIBP-CorV vaccines to Beta variant decreased by 1.59 times and 1.56 times [6]. These results suggest that the protective efficacy of COVID-19 vaccines against the Beta variant decreased [6, 27, 28].
The Gamma variant firstly reported in Brazil in November 2020 includes 12 characteristic mutations: L18F, T20N, P26S, D138Y, R190S, K417T, E484K, N501Y, D614G, H655Y, T1027I, V1176F (https://outbreak.info/). Interestingly, both Gamma and Beta variants share four mutations, including K417T, E484K, N501Y, and D614G. These mutations may also affect the efficacy of the COVID-19 vaccines against the Gamma variant infection and the effectiveness of monoclonal antibodies. Wang Pengfei et al. [29] investigated the neutralizing effect of 18 monoclonal antibodies, convalescent plasma, and serum after vaccination with the Moderna or Pfizer vaccine against Gamma variant infection. The results showed that compared with SARS-CoV-2 wild type, the neutralization of various monoclonal antibodies, convalescent plasma, and vaccinated serum against Gamma variant decreased or even lost [29]. Similarly, a study in Italy collected the sera of 90 health care workers (HCWs) who received two doses of the BNT162b2 vaccine and tested their neutralizing antibodies titers against the SARS-CoV-2 variant. The results showed that the neutralization titer of neutralizing antibody induced by BNT162b2 vaccine to Gamma variant was 3.3 times lower than that of Alpha variant [30]. In addition, another study on the neutralization effect of serum antibody after two doses of BNT162b2 vaccine showed that the neutralization effect of neutralizing antibody against Gamma variant was significantly lower than that of neutralizing antibody against original strain [20]. Furthermore, it was found that there was a significant difference in the neutralization of serum antibodies collected on days 10–14 and 15–20 after the second dose of vaccine [20]. These data show that the neutralization effect of the BNT162b2 vaccine against the Gamma variant is reduced, and the antibody level induced by the vaccine varies at different times after vaccination.
Delta variant was first discovered in India in October 2020. About six months
later, the variant became the most common SARS-CoV-2 variant in India
(https://nextstrain.org/ncov/gisaid/global?f_country=IndiaFZ). Delta variant’s S
protein has eight characteristic mutations: T19R, E156G, del157/158, Ll452R,
T478K, D614G, P681R and D950N (https://outbreak.info/). Studies have shown that
L452R and P681R mutations may enhance the infection and proliferation of the
virus [30]. Delta variant is an immune escape variant with a more robust host
cell entry and fusion ability, and its L452R mutation can make itself escape the
attack of CD8
Previous studies have also assessed the neutralization of COVID-19 vaccine-induced neutralizing antibodies against the Delta variant. A study conducted in the UK evaluated the neutralizing antibodies induced by the BNT162b2 vaccine against the SARS-CoV-2 variant. Compared with the SARS-CoV-2 wild type, the titer of neutralizing antibody against Delta variant decreased by 5.8 times (95% CI, 5.0 to 6.9), which was significantly higher than that of the Alpha variant (decreased by 2.6 times, (95% CI, 2.2 to 3.1)) and Beta variant (reduced by 4.9 times, (95% CI, 4.2 to 5.7)) [35]. These data suggest that the protective ability of the BNT162b2 vaccine against the Delta variant was lower than that of the Alpha and Beta variants. Furthermore, studies have shown that compared with the G614 variant, the titer of neutralizing antibody induced by BNT162b2, mRNA-1273, and Ad26 COV2.S vaccines against Delta variant decreased by three times, two times, and 12 times, respectively [36]. It can be found that the Delta variant reduces the efficacy of the COVID-19 vaccines, especially the Ad26 COV2.S vaccine.
The Omicron variant was first detected in South Africa in November 2021 and was
defined as a VOC by WHO in the early stages of its spread. Subsequently, it was
found that the most significant difference from the Delta variant is the faster
transmission speed [56]. The S protein of the Omicron variant has 30
characteristic mutations (Fig. 1): A67V, del69/70, T95I, G142D, del143/145,
N211I, del212/212, G339D, S371L, S373P, S375F, S477N, T478K, E484A, Q493R, G496S,
Q498R, N501Y, Y505H, T547K, D614G, H655Y, N679K, P681H, N764K, D796Y, N856K,
Q954H, N969K, and L981F (https://outbreak.info/). The number of mutations in the
S protein of the Omicron variant is about twice that of other variants. Among
them, D614G is an essential mutation. It can also be found in Alpha, Beta, Gamma,
Delta, Lambda, M
The Omicron variant has strong transmissibility and immune evasion [56] and can lead to reinfection with the Omicron variant in COVID-19 patients already infected with other variants [38, 39]. It was reported that the Omicron variant has a 6-fold higher risk of reinfection than the Delta variant [41]. In addition, studies from the UK and South Africa found that compared with the Delta variant, the disease severity and risk of death of patients infected with the Omicron variant were significantly reduced [40, 41], which may be due to the reduced ability of Omicron variant to resist the interferon response of host cells [57].
In addition, neutralizing effect of antibodies induced by the COVID-19 vaccine against the Omicron variant and the effectiveness of COVID-19 vaccines against the Omicron variant were also evaluated. A previous study found that the neutralizing effect of antibodies induced by the BNT162b1/BNT162b2 vaccine against the Omicron variant decreased 41 times compared with SARS-CoV-2 wild type [43]. Compared with the Delta variant, the neutralizing effect of antibodies induced by the BNT162b2 vaccine against the Omicron variant was reduced by 12–44 times [44]. Compared with the SARS-CoV-2 wild type, the neutralization effect of BNT162b2, mRNA-1273, and Ad26 COV2.S vaccines against the Omicron variant was reduced by 7–45 times [45]. In addition, the efficacy of 2 and 3 doses of mRNA vaccines (BNT162b2 and mRNA-1273) against Delta variant were 85% (95% CI, 83 to 87%) and 94% (95% CI, 92 to 95%), and against Omicron variant were 65% (95% CI, 51 to 75%) and 86% (95% CI, 77 to 91%), respectively [46]. From these data, it is not difficult to find that the protection efficiency of the mRNA vaccines against the Omicron variant is lower than that of the Delta variant, and the protection efficiency of the three-dose vaccination is significantly higher than that of the two-dose vaccination. In short, the Omicron variant is a variant with more mutation sites and high transmission power, which leads to the decline of the neutralization effect of vaccine-induced neutralizing antibodies and patient serum antibodies and another wave of COVID-19 pandemic.
Lambda variant was first discovered in Peru [58], and its S protein has eight characteristic mutations, including G75V, T76I, R246N, del247/253, L452Q, F490S, D614G, and T859N (https://outbreak.info/). The two mutations, F490S and L452Q in the receptor domain, are antigenic site mutations recognized by the antibody. Studies have shown that L452Q mutation enhances the infectivity of the Lambda variant and its affinity for ACE2. F490S can prevent the neutralization of the virus by vaccine-induced neutralizing antibodies and reduce the efficacy of the vaccine and monoclonal antibody [47, 48]. Compared with the D614G strain, the Lambda variant increased the infection ability of LLC-MK2 (rhesus monkey kidney cell line) and Calu-3 cells by 1.6 and 3.3 times, and the neutralization effect of convalescent serum against the Lambda variant is reduced by 1.3 times, respectively [48]. The possible reason may be that the concentration of serum antibodies in the convalescent phase is low.
Moreover, another two studies confirmed the impact of the Lambda variant on COVID-19 vaccines. The results indicated that compared with D614G strain, the neutralisation effect of neutralizing antibodies induced by the CanSino’Ad5-nCoV vaccine, BNT162b2 vaccine, and mRNA-1273 vaccine against the Lambda variant decreased by 2.5 times, 3.0 times, and 2.3 times [48, 49], respectively. Similarly, another study showed that the neutralization effect of antibody-induced by CoronaVac vaccine against the Lambda variant was 3.05 times lower than that of SARS-CoV-2 wild type [50]. In conclusion, the Lambda variant reduces the efficacy of vaccines and monoclonal antibodies, which threatens the immune protection induced by vaccines and the effectiveness of monoclonal antibody therapy.
M
BCG is a live attenuated TB vaccine developed and bred by Albert Calmette and Camille Guerin about 100 years ago [62], and is the only vaccine approved for TB prevention. BCG has a certain protective effect on TB pleurisy and miliary TB in children [63]. At present, 154 countries and territories have introduced neonatal BCG vaccination policies, of which 53 countries report vaccination coverage of at least 95% [64]. In addition to its application in preventing TB, BCG has also been proven to prevent and treat infections caused by other bacteria and viruses and even some tumors. Many studies have found that the prevention and treatment effect of BCG on bacteria other than M. tuberculosis or tumors is related to the non-specific immune response induced by the BCG, which is called “trained immunity” [65, 66, 67].
Trained immunity refers to the non-specific immune response of the self-immune
system to the secondary stimulation of the same pathogen or different pathogens
after the initial challenge caused by some pathogen infection or vaccination. The
trained immune response is usually faster and more active (Fig. 3) [68, 69].
Previous studies have shown that when the ligand of some pathogenic
microorganisms binds to specific receptors on innate immune cells, it induces
long-lasting enhanced metabolic activity and epigenetic changes in innate immune
cells [70]. The increased metabolic activity provides faster energy support and
necessary metabolites for the immune response. Epigenetic changes increased the
susceptibility of transcription factors to promoter and enhancer regions of
inflammatory genes. When immune cells are repeatedly stimulated by the same
pathogen or infected with other pathogens, they accelerate the process of gene
expression. The combination of these metabolic and epigenetic effects
up-regulates the production of pro-inflammatory cytokines (IL-1
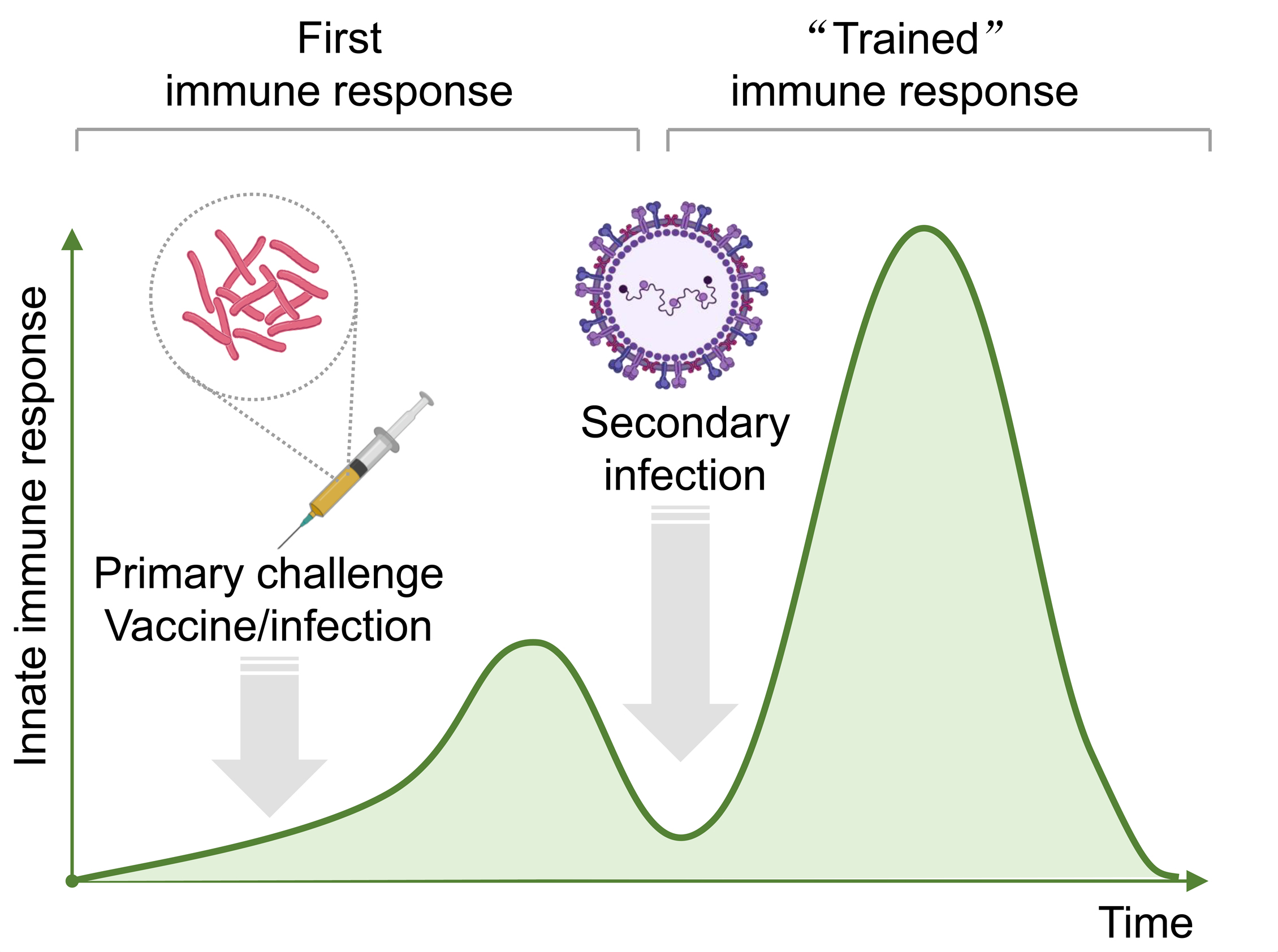
The curve of trained immunity over time. After the initial immunization, the host’s innate immune response was gradually enhanced and reached the first immune peak followed by a steadily decreased trend. Subsequently, the second infection with another pathogen will rapidly induce a more intense immune response and then reach a second immune peak followed by a gradually decreased trend.
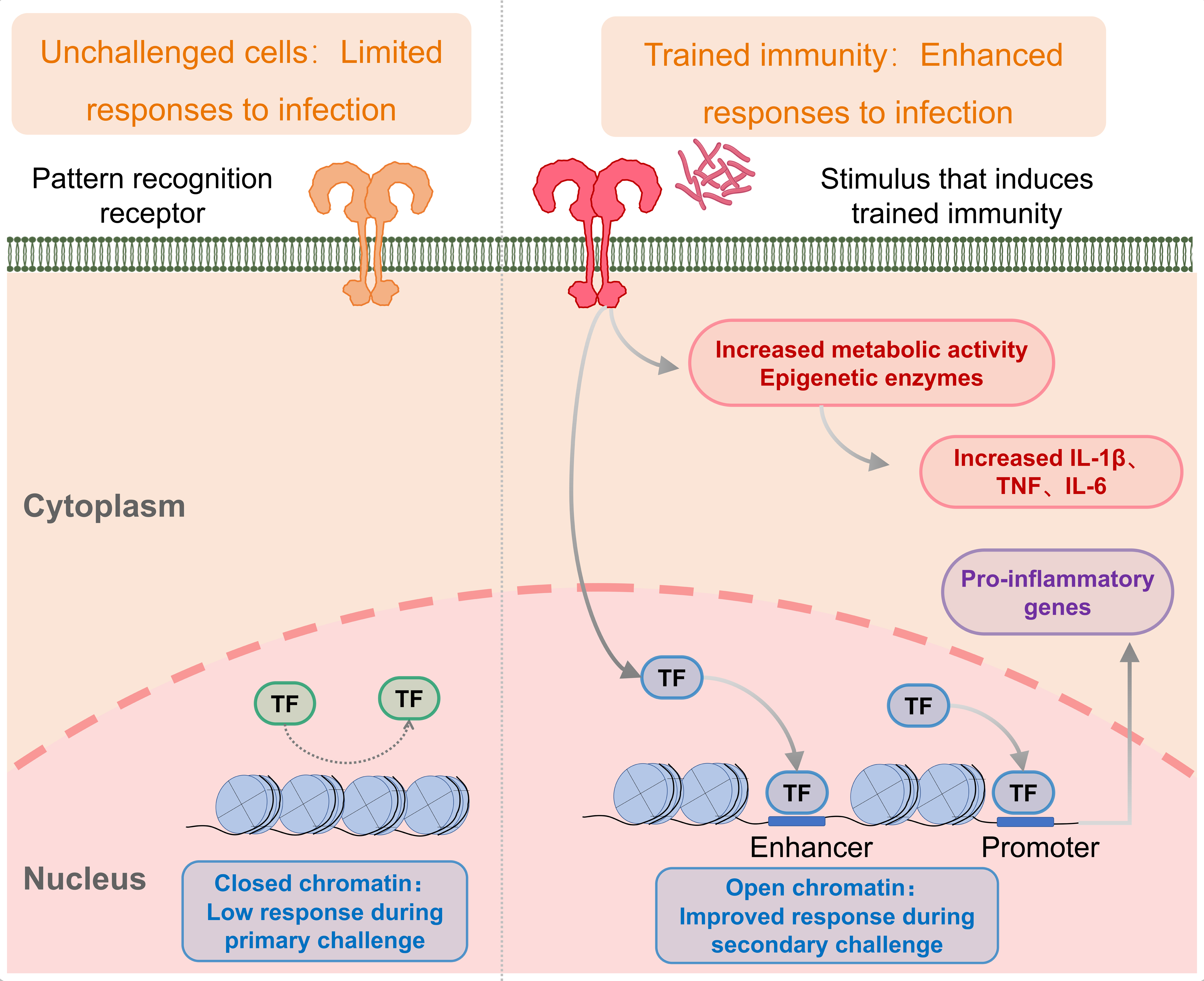
The mechanism of trained immunity induced by the BCG vaccine. Trained immunity is an enhanced innate immune response to different pathogens after an initial challenge, such as vaccination or infection. When the ligand of some pathogenic microorganisms binds to specific receptors on innate immune cells, it induces durable enhancement of metabolic activity and epigenetic changes in innate immune cells. Epigenetic changes increase the susceptibility of transcription factors to promoter and enhancer regions of inflammatory genes when immune cells undergo repeated stimulation or other pathogens, accelerating the gene expression process. In addition, the increased metabolic activity provides faster energy support and necessary metabolites for the immune response. This combination of metabolism and epigenetics prompts the immune system to respond faster and more vigorously to secondary stimuli caused by the same or different pathogens.
Interestingly, there is growing evidence that BCG vaccination induces trained immunity against multiple respiratory viruses, such as RSV and influenza viruses [71]. Therefore, in the face of the COVID-19 pandemic, some researchers have proposed a hypothesis that BCG vaccination may be one of the ways to prevent or control the spread of COVID-19 and reduce the morbidity and mortality of COVID-19 [2, 72].
To explore the impact of BCG vaccination on the incidence, mortality and
severity of COVID-19, many studies have been conducted in many countries and
organizations around the world. Statistics show a correlation between the
morbidity and mortality of COVID-19 and BCG vaccination rates in different
countries and regions at the beginning of the global pandemic (Table 2, Ref. [12, 73, 74, 75, 76, 77, 78, 79, 80, 81, 82, 83, 84, 85, 86, 87, 88, 89]). For
example, the number of confirmed COVID-19 cases in developed countries such as
North America and Western Europe is significantly higher than that in developing
countries such as South America, Asia, and Africa, where BCG vaccination policies
for newborns are widely adopted. Observing this phenomenon, Madan et al. [73]
published a cross-sectional study that included data collected from 174 countries. The 174 countries were divided into four groups based on TB incidence and
BCG vaccination coverage, and the incidence and mortality of COVID-19 in each
group were statistically analyzed. The results showed that the incidence and
mortality of COVID-19 in countries with a high incidence of TB were lower than
those in countries with low TB incidence. Similarly, the incidence and mortality
of COVID-19 were lower in countries with high BCG vaccination coverage [73].
Furthermore, a large-scale observational study involved in 175 countries found
similar results, the results show that countries with a universal BCG vaccination
program (n = 138) have significantly lower incidence of COVID-19 than
countries without a universal BCG vaccination program (n = 37) [0.0147
Studies | Years | Subjects | Results | Reference |
A cross-sectional study | 30 May 2020 | 174 countries | Countries with high TB incidence have lower levels of COVID-19 than countries with low TB incidence. Similarly, countries with high BCG coverage have lower rates of COVID-19, suggesting that some protective mechanisms exist in TB-endemic areas. | [73] |
An observational study | 24 April 2020 | 175 countries | Countries with neonatal BCG vaccination policies (n = 138) had lower prevalence and COVID 19 related deaths than countries without neonatal BCG vaccination policies (n = 37). | [74] |
Meta regression analysis | 5 Sep 2020 | 160 countries | Compared with unvaccinated countries, countries with BCG coverage |
[75] |
Linear regression analysis | 4 Nov 2020 | 213 countries | The incidence of COVID-19 in countries where BCG is recommended is significantly lower than in countries where BCG is not recommended, and the mortality rate of COVID-19 in countries where BCG vaccination coverage is |
[12] |
Epidemiological analysis | 9 Jul 2020 | Worldwide | There is a strong correlation between national BCG mandatory vaccination policies and COVID-19 mortality. A 10% increase in the BCG index was associated with a 10.4% decrease in COVID-19 deaths. These results indicate that BCG vaccination has a protective effect on COVID-19. | [76] |
Multiple regression analysis | 31 Jul 2020 | 171 countries | Countries with universal BCG vaccination had a 30-fold reduction in COVID-19 deaths (95% CI, 17 to 52) compared with countries without universal BCG vaccination; BCG vaccination helps reduce mortality from COVID-19. | [77] |
Longitudinal, retrospective observational study | 19 Jan 2021 | 6201 HCW | The serum positive rate of SARS-CoV-2 and clinical symptoms associated with COVID-19 were significantly lower in the BCG vaccination group than in the non-BCG vaccination group, suggesting that BCG may have certain value in reducing the severity of COVID-19 disease. | [78] |
A cohort study | 9 Jul 2020 | 120 adult patients, | Individuals vaccinated with BCG were less likely to require hospitalization during the course of disease (3.7% vs 15.8%, p = 0.019). Suggesting that BCG has the potential to reduce the severity of COVID-19. | [79] |
An observational study | 5 June 2020 | In Rhode Island, US | BCG vaccine was associated with reduced mortality rates (while adult pneumococcal and adult seasonal influenza vaccines were not ) | [80] |
An Ecological study | 16 Mar 2021 | 25 level 4 European countries | Countries with high BCG coverage in children have a reduced risk of COVID-19, while there is no significant correlation between MCV coverage and COVID-19. But there are significant differences in Healthcare Access and Quality Index (HAQI) across the 140 countries. The correlation between BCG coverage and COVID-19 becomes uncertain when combined with the HAQI function system analysis. | [81] |
A Multivariable Analysis | 5 Aug 2020 | 140 countries | Countries with mandatory BCG vaccination policies have lower incidences of COVID-19 and disease-related deaths than countries without BCG vaccination policies. Countries that eliminated BCG vaccination policies before 2000 showed no significant difference in COVID-19 incidence and disease-related mortality compared with countries that had never implemented BCG vaccination policies. | [82] |
An Ecological study | 3 Aug 2020 | 135 countries | BCG coverage (%) was inversely associated with COVID-19-related mortality, but not morbidity. | [83] |
Correlation analysis | 25 Jan 2021 | 173 countries | Conclusions support the view that BCG vaccination may help prevent deaths from COVID-19. | [84] |
A Multivariable Analysis | 11 Jul 2020 | Worldwide | BCG coverage was negatively correlated with the number of deaths per million (DPM), especially among young people 0-24 years of age. These results suggest that BCG vaccination can help control the spread of COVID-19 and reduce the severity of the disease. | [85] |
Linear regression analysis | 28 Aug 2020 | 55 countries | A significant negative correlation was observed between COVID-19 morbidity and mortality and BCG coverage. | [86] |
A Cohort Study | 5 Aug 2020 | 225 countries | BCG vaccination is safe and reduces the incidence of COVID-19 | [87] |
Investigative study | 12 Aug 2020 | 430 people | In Japan, BCG coverage among young people has a significant impact on preventing local transmission of COVID-19. | [88] |
Exploratory study | 22 Oct 2020 | Japan | Countries that have implemented neonatal BCG vaccination policies have reduced COVID-19-related deaths by 58%. However, the results of this exploratory study are based on secondary cross-sectional data. | [89] |
To further explore the relationship between BCG vaccination coverage and
COVID-19, Klinger D et al. [75] conducted a meta-regression analysis based on the data
from 160 countries. They divided countries into three groups based on BCG
vaccination coverage
Those studies indicate a negative correlation between BCG vaccination and the incidence and mortality of COVID-19. However, many studies have shown that in the early stage of epidemiological studies, there are often a large number of confounding factors, which have a significant impact on epidemiological data and often lead to distortion of research results. Confounding factors such as differences in health systems, levels of economic development, income, education, population, rural and urban environments, time lag between COVID-19 outbreaks, number and standard of diagnostic tests, and national control strategies are also present in the above studies [90]. To minimize the impact of these confounding factors on epidemiological results, some researchers analyzed the relationship between the BCG index (the level of universal BCG vaccination coverage in a country) and COVID-19 mortality after controlling confounding factors. For example, Escobar and his colleagues performed a major epidemiological study among European countries [76]. A negative correlation was found between the BCG index and COVID-19 mortality in European countries. On the other hand, a 10% increase in the BCG index was associated with a 10.4% decrease in COVID-19 mortality, suggesting that BCG may have a protective effect during the COVID-19 epidemic. With the outbreak of COVID-19 in the Americas, this study analyzed COVID-19-related mortality rates in STATES without BCG vaccination policies and states with BCG vaccination policies located at major entry points in Mexico and Brazil and found that COVID-19 mortality rates in states without BCG vaccine were significantly higher than in countries with BCG vaccination [76]. However, this study did not consider the significantly higher population density in South America than in North America, so the results are controversial. Another study performed multiple regression analyses on data from 171 countries, adjusting for socioeconomic and climatic covariates to avoid potential confounding factors [77]. The results showed a 30-fold reduction in COVID-19 deaths in countries with universal BCG vaccination policies compared with countries without universal BCG vaccination policies, further supporting the role of BCG vaccination in reducing COVID-19 deaths [77].
Although the above ecological or epidemiological data to some extent support the negative association between BCG vaccination rates and COVID-19 morbidity and mortality, other studies have questioned this. Some epidemiological studies have shown no clear association between BCG vaccination policies and morbidity and mortality of COVID-19 (Table 3, Ref. [24, 29, 91, 92, 93, 94, 95, 96, 97]). For example, Szigeti et al. [91] conducted a comprehensive epidemiological study using data from public databases in 68 countries. The relationship between BCG prevalence and mortality in COVID-19 cases found no significant correlation [91]. In addition, several previous epidemiological analyses suggested that although some ecological studies showed that BCG vaccination had a protective effect on COVID-19, the results of these studies were significantly affected by confounding factors. Their literature review found that, after adjusting for confounding variables, there was no longer a clear association between BCG vaccination policy and COVID-19 morbidity and mortality, especially after adjusting for detection rates [24].
Studies | Years | Subjects | Results | Reference |
Validation study | 7 Oct 2020 | 68 countries | Global COVID-19 epidemiological data are unreliable, and the results do not definitively indicate whether BCG has a protective effect against COVID-19 infection. | [91] |
Analyses of the epidemiological studies | 27 Oct 2020 | Review of literature | After adjusting for confounding variables (particularly detection rates), there was no association between BCG vaccination policy and morbidity or mortality from COVID-19. | [24] |
Cohort study | 13 May 2020 | 3064 + 2869 people (Israelis) | There was no statistical difference in the positive rate of COVID-19 detection between the BCG vaccination group and the non-BCG vaccination group. | [29] |
Regression discontinuity study | 23 Aug 2020 | 2,044,848 people in Sweden | BCG vaccination at birth does not protect against COVID-19 in middle-aged individuals | [92] |
Analyses of the epidemiological studies | 9 May 2020 | Review of literature | There is insufficient evidence to support or deny the hypothesis that COVID-19 reduces morbidity and mortality in countries where compulsory BCG vaccination. | [93] |
Comparative analyses of the data | 14 Aug 2020 | Review of literature (18 countries) | There is no evidence that BCG vaccination has a beneficial effect on reported COVID-19 cases or deaths. | [94] |
A systematic review and meta-analysis | 11 May 2020 | Review of literature | Studies have rejected a true correlation between COVID-19 incidence and BCG vaccination rates and/or national vaccination policies. | [95] |
Retrospective study | 21 Sep 2020 | 334 people | Intravesical BCG injection did not reduce the incidence of Novel coronavirus infection. | [96] |
A retrospective cross-sectional study | 17 Sep 2020 | 123 adults | BCG vaccination is not associated with the severity of COVID-19 pneumonia. Instead, age and low income are significant determinants of severe COVID-19 pneumonia. | [97] |
The above studies indicate that confounding factors significantly impact the analysis and evaluation of BCG vaccination’s protective effect against COVID-19, leading to the opposite conclusion of the study. Therefore, several cohort studies or longitudinal retrospective observational studies have further analyzed the relationship between BCG vaccination rates and COVID-19 morbidity and mortality. The advantage of these studies over previous epidemiological and ecological studies is that they eliminate confounding factors as much as possible. In a longitudinal, retrospective observational study performed in 6201 HCWs, 29.6% of the participants in the experimental group reported a history of BCG vaccination, and 68.9% of the participants in the control group had never received BCG vaccination [78]. Clinical symptoms associated with COVID-19 and serum anti-SARS-CoV-2 IgG concentrations were compared. The results showed that the sero-positive rate of SARS-CoV-2 and clinical symptoms related to COVID-19 were significantly lower in the BCG vaccinated group than those in the non-BCG vaccinated group [78].
Similarly, a Rhode Island study analyzed the hospitalization rates of 120 patients with COVID-19 and found that those vaccinated with BCG had lower hospitalization rates than those who had not been vaccinated with BCG [79]. These findings suggest that BCG may have some value in reducing the severity of COVID-19 disease. Though the above two studies in order to reduce the influence of confounding factors, choose the same country of the people in the same area as the research object, the application of unified testing means to control confounding variables as much as possible. However, there are still unavoidable potential confounding factors, such as the research object of the age, BCG vaccination time, growing environment and condition. To further reduce the influence of these variables on the results, a cohort study in Israel compared 3064 patients with BCG vaccination and 2869 patients without BCG vaccination. The positive rate of the COVID-19 test in the two groups was analyzed, and no statistically significant difference was found between the two groups [29]. Since Israel abolished its mandatory BCG neonatal vaccination program in 1982, the study looked at individuals born three years before and three years after the BCG vaccination program stopped. This study minimised the impacts of confounding factors such as health systems, national levels of economic development, education, rural and urban environments, time lag between COVID-19 outbreaks, number and standard of diagnostic tests, and national control strategies. In addition, Aksu K et al. [92] conducted a natural experiment in Sweden to evaluate the effect of BCG vaccination on COVID-19 related outcomes by using the regression discontinuities method. Two groups of volunteers were recruited, including 1026,304 people born before 1975 and 1018,544 people born after 1975. The impact of BCG vaccination on COVID-19 related outcomes was evaluated between two groups. Analysis results showed that although BCG vaccination at birth was not protective against COVID-19 in middle-aged individuals, universal BCG vaccination reduced the number of COVID-19 cases by 19% and the number of hospitalizations by 25% [92]. The rigorous experimental design and sufficiently large sample size of this study allow its reliability and precision to exceed that of a randomized controlled trial.
In summary, there is pronounced heterogeneity in the results of these studies. Therefore, it is impossible to answer whether BCG vaccination is meaningful to control the spread of COVID-19, decrease the morbidity and mortality of COVID-19, and reduce the severity of COVID-19 disease. Furthermore, although the above studies have minimized the interference of confounding factors, there are still many potential confounding factors that may affect the study results. Therefore, many clinical trials are needed to assess the significance of BCG vaccination in controlling the COVID-19 pandemic.
At present, as many as 56 clinical trials are conducted to evaluate the protective efficacy of BCG in preventing COVID-19 worldwide, including 13 in phase IV, 27 in phase III, 4 in phase II, and 13 in the unknown phase (Table 4). These 56 clinical trials will be categorized and discussed by country, and we will focus on analyzing the clinical trial status, results, and the potential impact on fighting against COVID-19.
Trial ID | Vaccine (strain) | Countries | Recruitment Status | Target size | Phase | Population Age | Intervention | Primary outcome |
ACTRN12620000707965 | VPM1002 (NA) | Australia | Not Recruiting | 3468 | Phase 3 | 18 Years and older (HRPs) | Experimental group: 0.1 mL VPM1002 with 2–8 × 10e |
The incidence of SARS CoV-2/COVID-19 infection is associated with acute respiratory symptoms. |
Placebo: 0.1 mL 0.9% sodium chloride, i.d. | ||||||||
NCT04327206 | BCG vaccine (Danish strain 1331) | Australia Brazil Netherlands Spain United Kingdom | Not recruiting | 10,078 | Phase 3 | 18 Years and older (HCWs) | Experimental group: 0.1 mL vaccine with 2–8 × 10e |
COVID-19 disease incidence; Severe COVID-19 disease incidence. |
Placebo: Intracutaneously 0.1 mL NaCl 0.9% i.d. | ||||||||
ISRCTN47802196 | BCG (Moreau strain) | Brazil | Recruiting | 200,805 | N/A | N/A | Experimental group: BCG vaccine (Moreau) strain, no placebo. | COVID-19 incidence; COVID-19 severity |
NCT04659941 | BCG (NA) | Brazil | Recruiting | 1000 | Phase 2 | 18 Years and older (HCWs) | Experimental group: 0.1 mL of the reconstituted vaccine to be administered intradermally; | Compare the cumulative incidence of SARS-CoV-2 infection and severe forms of COVID-19; Assess the BCG vaccine-mediated immune response in HCWs. |
Placebo 1: 0.1 mL of 0.9% NaCl saline solution to be administered intradermally | ||||||||
RBR-4kjqtg | BCG (NA) | Brazil | Recruiting | 400 | Phase 2 | 18 Years and older (HCWs) | Experimental group: receive a dose of BCG vaccine; The control group will not be vaccinated. | Reduction of positivity for COVID-19; Reduction of HCWs related to COVID-19 |
RBR-5ysj54 | BCG (NA) | Brazil | Not Recruiting | 1000 | N/A | 18 years and older | Experimental group: vaccinated with BCG vaccine; Control group: normal saline. | Expected to find a smaller number of coronavirus infections and severe coronavirus infections in the BCG vaccinated group compared to those inoculated with placebo. |
NCT04369794 | BCG (NA) | Brazil | Recruiting | 1000 | Phase 4 | 18 years and older | Experimental group: 0.1 mL BCG vaccine with 2–8 × 10e |
Clinical evolution of COVID-19; SARS-CoV-2 elimination; Seroconversion rate and titration |
Placebo: 0.9% saline solution, i.d. | ||||||||
NCT04439045 | VPM1002 (rBCGΔureC::hly) | Canada | Not recruiting | 3626 | Phase 3 | 18 years and older | Experimental group: 0.1 mL VPM1002 with 2–8 × 10e |
Incidence of SARS-CoV-2 infection (confirmed by positive test) following vaccination with either VPM1002 or placebo |
Placebo: 0.1 mL 0.9% sodium chloride, i.d. | ||||||||
NCT04826718 | BCG (NA) | Cape Verde | Recruiting | 400 | N/A | 18 years and older | Other: Questionnaire | Total number of days absent from work due to COVID-19; Unplanned Absenteeism; Symptomatology after infection by SARS-CoV-2; Presence or absence of anti-SARS-CoV-2 Acs; Duration of anti-SARS-CoV-2 Acs |
NCT04641858 | BCG vaccine (Danish strain 1331) | Cape Verde; Guinea-Bissau; Mozambique | Recruiting | 1050 | Phase 4 | 18 Years and older (HCWs) | Experimental group: 0.1 mL BCG vaccine with 2–8 × 10e |
Days of unplanned absenteeism due to illness |
Placebo: 0.1 mL 0.9% NaCl, i.d. | ||||||||
NCT04362124 | BCG (NA) | Colombia | Not recruiting | 1000 | Phase 3 | 18–65 Years old (HCWs) | Experimental group: 0.1 mL BCG with 1 × 10e |
Incidence of COVID-19 cases confirmed or probable in the study population. |
Placebo: 0.1 mL normal saline solution, i.d. | ||||||||
NCT04542330 | BCG vaccine (Danish strain 1331) | Denmark | Recruiting | 1900 | Phase 3 | 65–110 Years old | Experimental group: 0.10 mL BCG with 2–8 × 10e |
“Acute infection” identified either by a doctor, antibiotics use, hospitalisation, or death due to infection. |
Placebo: 0.1 mL normal saline (0.9% NaCl), i.d. | ||||||||
EUCTR2020003904-15-DK | BCG vaccine (Danish strain 1331) | Denmark | Authorised | 1900 | Phase 3 | 65 years and older | Experimental group: 0.1 mL BCG vaccine with 2–8 × 10e |
The primary outcome is acute infection identified by a doctor, antibiotics use, hospitalisation or death due to infection. |
Placebo: 0.1 mL NaCl. | ||||||||
NCT04373291 | BCG vaccine (Danish strain 1331) | Denmark | Completed | 1293 | Phase 3 | 18–100 Years old (HCWs) | Biological: BCG-Denmark; Biological: Saline. | Number of days of unplanned absenteeism for any reason |
EUCTR2020001888-90-DK | BCG vaccine (Danish strain 1331) | Denmark | Authorised | 1500 | Phase 3 | 18–64 Years old (HCWs) | Experimental group: 2–8 × 10e |
To reduce absenteeism among HCWs with direct patient contacts during the COVID-19 pandemic. |
Placebo: Solution for injection, i.d. | ||||||||
NCT04347876 | BCG (NA) | Egypt | Recruiting | 100 | N/A | 12–80 Years old | Group A: COVID-19 positive with positive tuberculin test; Group B: COVID-19 positive with a negative tuberculin test. | Pneumonia severity index; Need for ICU admission |
NCT04350931 | BCG vaccine (Danish strain 1331) | Egypt | Not recruiting | 900 | Phase 3 | 18 years and older | Experimental group: 0.1 mL BCG with 2–8 × 10e |
Incidence of confirmed COVID-19; Effectiveness of BCG vaccine |
Placebo: 0.1 mL normal saline (0.9% NaCl), i.d. | ||||||||
NCT04384549 | BCG vaccine (Danish strain 1331) | France | Recruiting | 1120 | Phase 3 | 18 Years and older (HCWs) | Experimental group: 0.1 mL BCG vaccine (AJ Vaccine) with 2–8 × 10e |
Incidence of documented COVID-19 among health care workers exposed to SARS-CoV-2 and vaccinated with BCG compared to placebo. |
Placebo: One i.d. of 0.1 mL NaCl | ||||||||
EUCTR2020001678-31-FR | BCG vaccine (Danish strain 1331) | France | Authorised | 1120 | Phase 3 | 18 Years and older (HCWs) | Experimental group: 2–8 CFU/mL, i.d.; | The protection of BCG for HCWs exposed to COVID-19 |
Placebo: Solution for injection, i.d. | ||||||||
NCT04435379 | VPM1002 (rBCGΔureC::hly) | Germany | Recruiting | 2038 | Phase 3 | 60 years and older | Experimental group: 0.1 mL VPM1002 with 2–8 × 10e |
Number of days with severe respiratory disease at the hospital and at home |
Placebo: Physiological saline 0.1 mL. | ||||||||
NCT04387409 | VPM1002 (rBCGΔureC::hly) | Germany | Active, not recruiting | 59 | Phase 3 | 18 Years and older (HCWs) | Experimental group: 0.1 mL VPM1002 with 2–8 × 10e |
Number of days absent from work due to respiratory disease (with or without documented SARS-CoV-2 infection) |
Placebo: Physiological saline 0.1 mL, i.d. | ||||||||
EUCTR2020-001675-33-DE | VPM1002 (rBCGΔureC::hly) | Germany | Authorised | 2038 | Phase 3 | 18 years and older | Experimental group: VPM1002, 2–8 × 10e |
Reduction of days with severe respiratory infectious diseases at the hospital and/or at home in elderly subjects |
Placebo: Solution for injection, i.d. | ||||||||
EUCTR2020-001376-15-DE | VPM1002 (rBCGΔureC::hly) | Germany | Prematurely Ended | 59 | Phase 3 | 18 Years and older (HCWs) | Experimental group: VPM1002, 2–8 × 10e |
Health Care Workers absenteeism |
Placebo: Solution for injection, i.d. | ||||||||
NCT04414267 (EUCTR2020-002448-21-GR) | BCG (Moscow strain 361-1) | Greece | Completed | 301 | Phase 4 | 50 years and older | Experimental group: 0.1 mL BCG vaccine, i.d.; | Susceptibility for COVID-19 |
Placebo: 0.1 mL of sodium chloride 0.9%, i.d. | ||||||||
NCT03296423 | (BCG vaccine Bulgaria strain 1331) | Greece | Completed | 200 | Phase 4 | 65 years and older | Experimental group: 0.1 mL BCG Vaccine i.d.; | Time to the first infection |
Placebo: 0.1 mL of sodium chloride 0.9%, i.d. | ||||||||
EUCTR2020-001783-28-HU | BCG vaccine (Danish strain 1331) | Hungary | Authorised | 1000 | Phase 3 | 18–64 Years old (HCWs) | Experimental group: BCG vaccine, suspension for injection, i.d.; | Health Care Workers absenteeism |
Placebo: Solution for injection, i.d. | ||||||||
CTRI/2020/09/027684 | BCG (NA) | India | Recruiting | 400 | N/A | 18–50 Years old (HCWs) | Experimental group: 0.1 mL BCG vaccine i.d.; | Rate of infection of COVID-19 in healthcare workers re-vaccinated with BCG as compared to controls |
Placebo: unknown | ||||||||
CTRI/2020/07/026668 | BCG (NA) | India | Not Recruiting | 800 | Phase 3 | 18–60 Years old | Experimental group: 0.1 mL BCG vaccine i.d.; | Incidence of COVID-19 by 9 months of follow-up. |
Placebo: 0.1 mL Normal saline | ||||||||
NCT04475302 | BCG (NA) | India | Not recruiting | 2175 | Phase 3 | 60–80 Years old | Experimental group: Each 1 mL contains between 2 × 10e |
Mortality due to COVID-19 disease |
Placebo: No intervention | ||||||||
CTRI/2020/06/025854 | BCG (NA) | India | Not Recruiting | 1450 | N/A | 60–95 Years old | Experimental group: Single dose of 0.1 mL of BCG vaccine; | Proportion of patients with Severe COVID-19 disease and balance of death due to COVID-19 disease |
Placebo: No BCG vaccine. | ||||||||
CTRI/2020/06/025798 | BCG (NA) | India | Not Recruiting | 70 | Phase 4 | 18–80 Years old | Experimental group: 120 mg intravesical BCG (50 mL Normal Saline) | The rates of infectivity of SARS-CoV-2 virus in patients of NMIBC on BCG therapy |
CTRI/2020/05/025013 | BCG (Moreau strain) | India | Not Recruiting | 60 | Phase 2 | 20–40 Years old | Experimental group 1: 0.1 mL BCG with 2 × 10e |
The total duration of Hospitalization with COVID-19 symptoms such as febrile respiratory distress, decrease in Viral Titer, duration of COVID-19 symptoms |
Placebo 1: Tamiflu, Hydroxychloroquine, Azithromycin; Placebo 2: saline plus standard of care as indicated by DCGI | ||||||||
CTRI/2020/04/024833 | BCG vaccine (Danish strain 1331) | India | Not Recruiting | 1826 | N/A | 18–65 Years old (HCWs) | Experimental group: 0.1 mL, i.d.; | The proportion of HCWs with symptomatic COVID 19 disease 6 months after randomisation. |
Placebo: 0.1 mL Normal saline, i.d. | ||||||||
CTRI/2020/04/024749 | VPM1002 (NA) | India | Not Recruiting | 5946 | Phase 3 | 18–99 Years old (HCWs) | Experimental group: 0.1 mL reconstituted vaccine, i.d.; | Number of subjects with laboratory-confirmed COVID-19 infection among HCWs. |
Placebo: 0.1 mL 0.9% sodium chloride, i.d. | ||||||||
IRCT2020041-1047019N1 | BCG (NA) | Iran (the Islamic Republic of) | Recruiting | 500 | Phase 3 | 18 Years and older (HCWs) | Experimental group: 0.10 mL BCG Vaccine, i.d.; | COVID-19 infection |
Placebo: 0.1 mL of 0.9% NaCl solution, i.d. | ||||||||
NCT04461379 | BCG (Tokio 172 strain) | Mexico | Not recruiting | 908 | Phase 3 | 18 years and older | Biological: BCG vaccine; Other: Placebo | Cumulative incidence of infection in 6 months, the cumulative incidence of hospitalisation for COVID-19, incidence of specific Antibodies |
EUCTR2021-000182-33-NL | BCG (NA) | Netherlands | Authorised | 40 | Phase 4 | 18–64 Years old | Biological: BCG vaccine | Analyse whether BCG-vaccination before COVID-19 vaccination can enhance the immunogenicity of the COVID-19 mRNA vaccine developed by BioNTech and Pfizer. |
EUCTR2020-003470-47-NL | BCG vaccine (Danish strain 1331) | Netherlands | Authorised | 5200 | Phase 4 | 18 years and older | Experimental group: BCG Vaccine SSI concentrate; | Based on the accrual of the two endpoints, the primary endpoint will be either COVID-19. |
Placebo: Concentrate and solvent for solution for injection, i.d. | ||||||||
NCT04537663 | BCG vaccine (Danish strain 1331) | Netherlands | Recruiting | 5200 | Phase 4 | 60 years and older | Drug: BCG Vaccine; Other: Placebo | A clinically relevant respiratory tract infection, or COVID-19. |
EUCTR2020-002456-21-NL | BCG vaccine (Danish strain 1331) | Netherlands | Not Recruiting | 100 | Phase 4 | 18–50 Years old | Experimental group: M-M-RVAXPRO® powder and solvent for suspension for injection; | To investigate the effect of bisphosphonates and the MMR vaccine on BCG-induced trained immunity as a preventive approach against COVID-19. |
Placebo: Solution for injection. | ||||||||
NCT04417335 | BCG vaccine (Danish strain 1331) | Netherlands | Not recruiting | 2014 | Phase 4 | 60 years and older | Experimental group: BCG vaccine; | SARS-CoV-2 related hospital admission |
Placebo: 0.9% NaCl. | ||||||||
NCT04328441 | BCG vaccine (Danish strain 1331) | Netherlands | Not recruiting | 1500 | Phase 3 | 18 years and older | Experimental group: 0.1 mL BCG vaccine with 0.075 mg of attenuated Mycobacterium bovis; | Health Care Workers absenteeism |
Placebo: 0.1 mL NaCl 0.9%. | ||||||||
EUCTR2020-000919-69-NL | BCG vaccine (Danish strain 1331) | Netherlands | Not Recruiting | 1000 | Phase 4 | 18 Years and older (HCWs) | Experimental group: BCG vaccine concentrate and solvent for solution for injection, i.d.; | Health Care Workers absenteeism |
Placebo: Concentrate and solvent for solution for injection, i.d. | ||||||||
EUCTR2020-001591-15-NL | BCG vaccine (Danish strain 1331) | Netherlands | Authorised | 2000 | Phase 4 | 18 years and older | Experimental group: BCG vaccine; | Primary Objective: To reduce hospital admission of older adults during the SARS-CoV-2 outbreak. |
Placebo: Pharmaceutical Concentrate and solvent for solution | ||||||||
NL8609 | BCG (NA) | Netherlands | Not Recruiting | 100 | N/A | 18–50 years old | 1 Placebo treatment; 2. BCG vaccination; 3. BCG vaccination + oral bisphosphonate supplementation (alendronic acid); 4. BCG vaccination + MMR vaccine; 5. MMR vaccine alone. | The primary study parameter is the fold-increase in production of pro-inflammatory cytokines by PBMCs/monocytes following immunisation. |
NL8547 | BCG (NA) | Netherlands | Recruiting | 1600 | N/A | 60 years and older | Participants will be randomised between intracutaneous administration of BCG vaccine or placebo in a 1:1 ratio. | SARS-CoV-2 related hospital admission |
NL8477 | BCG (NA) | Netherlands | Not Recruiting | 1500 | N/A | 18 Years and older (HCWs) | Participants will be randomised between intracutaneous administration of BCG vaccine or placebo in a 1:1 ratio. | Number of days of unplanned absenteeism for any reason |
NCT04648800 | BCG (Moreau strain) | Poland | Recruiting | 1000 | Phase 3 | 25 Years and older (HCWs) | Experimental group 2: 0.10 mL BCG with 1.5–6 × 10e |
Death and life-or health-threatening condition |
Placebo: 0.1 mL normal saline (0.10% NaCl), i.d. | ||||||||
EUCTR2020-002111-22-PL | BCG (NA) | Poland | Authorised | 1000 | Phase 3 | 18–64 Years old (HCWs) | Experimental group: Anti-Tuberculosis Vaccine BCG 10, powder and solvent for suspension for injection, i.d.; | COVID-19 cases and deaths |
Placebo: Solution for injection. i.d. | ||||||||
NCT04379336 | BCG vaccine (Danish strain 1331) | South Africa | Recruiting | 500 | Phase 3 | 18 Years and older (HCWs) | Experimental group: 0.1 mL BCG vaccine with 0.075 mg of attenuated Mycobacterium bovis, i.d.; | Incidence of HCWs hospitalised due to COVID-19 per arm. |
Placebo: 0.1 mL 0.9% NaCl, i.d. | ||||||||
NCT04453488 | RUTI |
Spain | Not recruiting | 315 | Phase 3 | 18 Years and older (HCWs) | Experimental group: 25 µg of fragmented, purified and liposomed heat-inactivated Mycobacterium tuberculosis bacilli in 0.3 mL, s.c.; | Positive serology at the end of the study or positive PCR test in the course of routine clinical practice |
Placebo: Physiological serum, 0.9% NaCl, s.c. | ||||||||
NCT04384614 | BCG (NA) | Tunisia | Withdrawn | 0 | N/A | 18–80 Years old | Diagnostic Test: Test PCR; Genetic: TDR; Other: Clinical Examination | Differences related to epidemiological, demographic characteristics |
NCT04632537 | BCG (Tice strain) | United States | Withdrawn | 550 | Phase 3 | 18–64 Years old | Experimental group: 0.10 mL BCG with 2 × 10e |
Incidence of symptomatic rt-PCR-confirmed SARS-CoV-2 infection |
Placebo: 0.1 mL normal saline (0.9% NaCl), i.d. | ||||||||
NCT04534803 | BCG (NA) | United States | Withdrawn | 0 | Phase 3 | 70 years and older | Experimental group: 0.1 mL of reconstituted BCG vaccine given intradermally at baseline. | Number of people diagnosed with severe Covid-19 disease |
Placebo: 0.1 mL of diluent (saline) given intradermally at baseline | ||||||||
NCT04348370 | BCG (Tice strain) | United States | Active, not recruiting | 1800 | Phase 4 | 18–75 Years old | Experimental group: 0.1 mL vaccine with 2 × 10e |
Incidence of COVID 19 Infection |
Placebo: 0.1 mL saline, i.d. | ||||||||
NCT02081326 | BCG (N/A) | United States | Active, not recruiting | 150 | Phase 2 | 18 Years to 65 Years | Experimental group: 2 BCG vaccinations spaced 4 weeks; | Change in HbA1c values in juvenileonset type 1 diabetics; COVID-19 and BCG Adaptive Study |
Placebo: 2 saline injections spaced 4 weeks | ||||||||
(1) The data were obtained from International Clinical Trials Registry Platform
(https://www.who.int/ictrp/en/), ClinicalTrials.gov
(https://clinicaltrials.gov/ct2/results?cond=COVID-19), EU Clinical Trials
Register (https://www.clinicaltrialsregister.eu/ctr-search/search), Australian
New Zealand Clinical Trials Registry (https://anzctr.org.au/Default.aspx),
Iranian Registry of Clinical Trials (https://en.irct.ir/trial/47279), and
Clinical Trials Registry-India (CTRI,
http://www.ctri.nic.in/Clinicaltrials/pmaindet2.php?trialid=44460) on March 27,
2022. (2) The list of abbreviations: BCG, Bacillus Calmette-Guérin; CFUs, colony-forming units; COVID-19, corona virus disease 2019; HCWs, healthcare workers; HRPs: High-Risk Participants; i.d., intradermal injection; s.c., subcutaneous injection; SARS-CoV-2, severe acute respiratory syndrome coronavirus 2. |
As of March 24, 2022, there have been 7,626,640 confirmed cases of COVID-19 with
21,836 deaths, and 33,943,469 doses of the COVID-19 vaccine have been
administered in the Netherlands (https://covid19.who.int). As a developed
country, the incidence and mortality of tuberculosis are very low in the
Netherlands, and the BCG vaccination program has not been widely promoted [3].
Therefore, it is admirable that Switzerlands is at the forefront of research in
evaluating the preventive effect of BCG against COVID-19. Currently, 11 clinical
trials have been conducted in the Netherlands to assess the protective effect of
BCG against COVID-19, including seven clinical trials in phase IV
(EUCTR2021-000182-33-NL, EUCTR2020-003470-47-NL, NCT04537663,
EUCTR2020-002456-21-NL, NCT04417335, EUCTR2020-000919-69-NL and
EUCTR2020-001591-15-NL), one clinical trial in phase III (NCT04328441), and three
clinical trials in unknown phase (NL8609, NL8547, and NL8477). Interestingly, the
BCG strains used in these clinical trials are all BCG Danish strand 1331 strain.
The objectives of these clinical trials are to assess the effect of BCG
immunization in reducing the hospitalization rate and COVID-19 disease severity
in the elderly (
The elderly have become a vulnerable group in the COVID-19 epidemic due to a
weakened immune system and primary diseases like hypertension and diabetes. Three
clinical trials (NCT04537663, NCT04417335, and NL8547) were performed to evaluate
the effect of BCG vaccination in reducing the hospitalization rate and the
severity of COVID-19 in 5200, 2014, and 1600 older adults (
India is a populous country second only to China, but the level of medical
security is relatively backward. According to the population data released by the
WHO, India has a population of 1.4 billion
(https://population.un.org/wpp/Download/Standard/Population/). By March 14, 2022,
India had 1.8 billion vaccinations, which means that only one dose of COVID-19
vaccine per person is average. Based on these disadvantages, India has 43,016,372
confirmed COVID-19 cases, ranking second only to the United States, and the
cumulative number of deaths is as high as 516,755, ranking third after the United
States and Brazil (https://covid19.who.int/table). Unlike Switzerland, India has
universal BCG vaccination, and BCG coverage has reached 92% in 2019
(http://www.bcgatlas.org/). As far as India’s challenges are concerned, if BCG
proves to be an excellent preventative against COVID-19, then India has one more
powerful weapon against COVID-19. To provide sufficient evidence for this
hypothesis, eight clinical trials have been conducted in India to evaluate the
preventive and protective effects of BCG against COVID-19, including one Phase IV
clinical trial (CTRI/2020/06/06/025798), three Phase III clinical trials
(NCT04475302, CTRI/2020/07/026668 and CTRI/2020/04/024749), one Phase II clinical
trial (CTRI/2020/05/05/0205013), and three clinical trials with unknown phase
(CTRI/2020/06/025854, CTRI/2020/09/027684 and CTRI/2020/04/04/024833). Seven of
these clinical trials were used to evaluate the preventive effect of BCG on
COVID-19, but one was used to assess the therapeutic effect of BCG immunization
in patients with COVID-19. Clinical trial CTRI/2020/05/025013 was carried out to
evaluate the therapeutic effect of BCG as a therapeutic agent on 60 hospitalized
patients aged 20–40 years with COVID-19 and explore the relationship between BCG
and COVID-19. These patients were divided into two groups, BCG group with 0.1 mL
BCG (2–8
Overall, the eight clinical trials conducted in India cover a wide range of age groups (20–40, 18–50, 18–60, 18–65, 18–80, 18–99, 60–80, and 60–95 years old, respectively) and high-risk groups such as HCWs, the elderly, and other high-risk workers. Therefore, if the final data from these clinical trials support the hypothesis that the BCG vaccine can prevent and treat COVID-19, widespread BCG vaccination in a populous and relatively underdeveloped country like India may be an effective and inexpensive way to combat the COVID-19 pandemic.
COVID-19 pandemic quickly disrupted health services, including Brazil’s Unified
Health System (SUS). As of March 25, 2022, Brazil has confirmed 29,729,991
COVID-19 cases, ranking third only to the United States and India; The total
number of deaths is 657,998, second to the United States. As of March 10, 2022,
383,413,147 doses of the COVID-19 vaccine had been administered, and total
vaccine doses administered per 100 population and persons fully vaccinated per
100 population are 180.38 and 71.35, respectively
(https://covid19.who.int/table). From these data, it can be seen that despite the
high COVID-19 vaccination rate in Brazil, the COVID-19 morbidity and mortality
remain high. To reverse this unfavourable situation, some scientists in Brazil
turned their attention to the BCG vaccine. At present, five clinical trials have
been conducted in Brazil, including one Phase IV clinical trial (NCT04369794),
two Phase II clinical trials (NCT04659941 and RBR-4kjqtg), and two clinical
trials with unknown phase (ISRCTN47802196 and RBR-5ysj54). NCT04369794 is a
multicenter, randomized, and double-blind clinical trial conducted in Brazil on
April 30, 2020, enrolling 1000 adults over 18 years. These participants will be
divided into the BCG vaccine group with 0.1 mL BCG (2–8
As of March 25, 2022, Denmark had a total of 3,029,228 confirmed cases and 5518 deaths, ranking 36th and 85th in the world, respectively. As of March 5, 2022, as many as 13,178,651 vaccine doses have been administered in Denmark. Total vaccine doses administered per 100 population and persons fully vaccinated per 100 population were 226.3 and 82.26, respectively, higher than the global average. Fortunately, although the number of confirmed cases is high in Denmark, the mortality rate is relatively low, which may be inseparable from the COVID-19 vaccination strategy actively pursued by the Danish government and the strong support of the health system. In addition, Denmark is actively exploring new ways to fight COVID-19, such as the BCG vaccine. At present, four clinical trials were performed in Denmark to evaluate the potential effect of BCG vaccination on preventing and controlling the COVID-19 pandemic. Like other countries, these clinical trials in Denmark have focused on groups at high risk for COVID-19, such as the elderly and HCWs. The clinical trial EUCTR2020-003904-15-DK conducted by the University of Southern Denmark and the clinical trial NCT04542330 conducted by Bandim Health Project intend to recruit 1900 and 1300 participants, respectively. All participants will be randomly divided into two groups, the intervention group will receive BCG Danish strain 1331, and the control group will receive normal saline. The objective of both clinical trials is to evaluate the protective effect of BCG immunization against COVID-19 infection in the elderly over 65 years. In addition to the elderly, HCWs in Denmark are also a high-risk group, accounting for more than 20% of the cumulative number of confirmed cases. A growing number of HCWs with COVID-19 will cause large-scale absenteeism, further leading to a shortage of medical resources. Therefore, there is an urgent need to develop strategies to prevent HCWs absenteeism. Two clinical trials, EUCTR2020-001888-90-DK (Estimated enrollment 1500) and NCT04373291 (Estimated enrollment 1293), have been conducted to evaluate the potential beneficial effects of BCG vaccination by reducing the work absenteeism rate of HCWs or alleviating the clinical process of COVID-19 infection. Both studies use the BCG vaccine to strengthen the non-specific protection of HCWs during the COVID-19 pandemic.
By analysing the available public data, we found an interesting thing: four clinical trials in Denmark were conducted independently by two different institutions, and each institution conducted a clinical trial in the elderly and HCWs. The rational layout of these clinical trials provides a model for a country to systematically evaluate BCG vaccines on the prevention and treatment of COVID-19. Furthermore, by comprehensively analyzing these experimental data, researchers can eliminate some confounding factors and improve the reliability and authenticity of the data.
As a developed country, the incidence of TB in Germany in 2020 is estimated to be 5.5 per 100,000 population, and the mortality rate is calculated to be 0.35 per 100,000 population (https://www.who.int/teams/global-tuberculosis-programme/data). Germany’s BCG vaccination policy has changed several times in its history, and the BCG policy was different between former East Germany and former West Germany. Former West Germany stopped recommending the BCG vaccine (BCG Denmark strain) in 1974 except for infants at risk, but former East Germany continued a mandatory BCG vaccine (Boehring-Hoechst/Jenapharm) until the German reunification in 1990 (http://www.bcgatlas.org/). Therefore, there is a significant difference in BCG coverage between former East Germany and former West Germany, but confounding factors such as geography, genetic background, and current epidemic prevention policy are similar in Germany. These unique advantages provide natural conditions for assessing the protective effect of BCG against COVID-19.
Currently, four clinical trials of the VPM1002 vaccine (a BCG replacement vaccine) have been performed in Germany. Another feature of the four clinical trials conducted in Germany is that they all used the VPM1002 vaccine instead of the BCG vaccine. In addition, the Max Planck Institute genetically modified the VPM1002 vaccine to improve its immune activity. Previous studies have demonstrated that the VPM1002 vaccine showed superior efficacy and safety than BCG in fighting against Mycobacterium tuberculosis infection in mice, guinea pigs, rabbits, nonhuman primates, HIV-unexposed newborn infants, and young adults [35, 38, 87, 88]. Thus, based on the experience of trained immunity induced by the BCG vaccine, some scientists speculate that the VPM1002 vaccine may also (partially) have potential preventive and therapeutic effects on COVID-19.
In Germany, Vakzine Projekt Management GmbH conducted four Phase III clinical
trials to evaluate the potential of the VPM1002 vaccine to prevent COVID-19.
Briefly, two clinical trials (NCT04435379 and EUCTR2020-001675-33-DE) were
performed to assess the efficacy and safety of the VPM1002 vaccine in reducing
the hospitalization rate of COVID-19 and severe respiratory infectious diseases
in adults. The two clinical trials have the same sample size of 2038, and the
difference is the vaccination dose and the age of the population (Table 4). The
former used a dose of 2–8
As a low TB incidence and mortality, BCG is not generally recommended for use in the United States. Currently, the Centers for Disease Control (CDC) of the United States recommends BCG vaccination only for HCWs and children who have a negative tuberculin skin test and who are continually exposed and cannot be separated from adults with a high risk of TB. However, as of March 25, 2022, the United States has confirmed 79,139,385 cases of COVID-19, with cumulative death of 967,905, ranking first in the world. Therefore, in the face of the world’s worst COVID-19 pandemic and widespread vaccination of specific COVID-19 vaccines, the United States has sought other anti-epidemic strategies, including BCG.
Researchers in the United States carried out two Phase III clinical trials
(NCT04632537 and NCT04534803) to confirm the prevention and improvement of the
BCG vaccine against COVID-19. However, both clinical trials were withdrawn due to
capital and other reasons. Furthermore, a randomized and controlled Phase III
clinical trial (NCT 04348370) was conducted in 1800 HCWs to assess the
BCG vaccine-induced trained immunity to protect HCWs from
SARS-CoV-2 infection. The HCWs were divided into the BCG vaccine group with 0.1
ml BCG (2
Like other high-income European countries, Greece has historically implemented universal BCG vaccination, but it is recommended only for high-risk groups, such as infants and children who have contact with an adult infected with TB, immigrants, and refugees (http://www.bcgatlas.org/). In Greece, as of March 25, 2022, as many as 2,884,100 confirmed cases of COVID-19 and 27,125 deaths have been reported to WHO, and a total of 20,181,739 vaccine doses have been administered. Currently, two clinical trials are being conducted in Greece to evaluate the efficacy of BCG in preventing COVID-19. As early as September 21, 2017, a randomized and controlled Phase IV clinical trial was conducted to explore the effect of BCG vaccination against influenza in the elderly. Interestingly, with the outbreak of the COVID-19 pandemic, the primary purpose of this clinical trial shifted from influenza to COVID-19. An interim analysis of this clinical trial was reported on April 29, 2020. The results showed that the first infection time (median 16 weeks vs 11 weeks) and the incidence of new infections (25.0% vs 42.3%) in the BCG group were significantly longer or lower than those in the placebo group [98]. These preliminary data suggest that BCG vaccination is safe, prolongs the first infection time, reduces the incidence of new infections, and has a protective effect on viral respiratory tract infection. This interim analysis provides evidence that “trained immunity induced by BCG vaccination may play a role in combating the COVID-19 epidemic”. Furthermore, another double-blind, placebo-controlled Phase IV clinical trial (NCT04414267 and EUCTR2020-002448-21-GR) was also performed to verify whether BCG vaccination can prevent COVID-19 and whether BCG vaccination can regulate the susceptibility of vaccinators to COVID-19 through clinical and immunological standards. The clinical trial is currently recruiting volunteers, and the trial results have not yet been announced. Overall, the two clinical trials in Greece were conducted by the same institution (Hellenic Institute for the Study of Sepsis), but the type of BCG strain, the number of volunteers, and the primary outcome were different between the two clinical trials (Table 4).
The COVID-19 pandemic has lasted more than two years since it began in late December 2019, killing more than 6 million people globally. And the continuous emergence of SARS-CoV-2 variants may lead to more pandemic waves. Since there is no effective drug to treat COVID-19, preventive vaccination has become the most promising method for epidemic prevention and control [99]. At present, there are 196 COVID-19 vaccines in preclinical development and 153 COVID-19 vaccines in clinical development. In addition, there are at least 13 COVID-19 vaccines under EUA, including inactivated vaccines, mRNA vaccines, virus vector vaccines, and subunit vaccines. These COVID-19 vaccines are designed based on SARS-CoV-2 S protein. Unfortunately, the S protein has the highest mutation rate, reducing the protection efficiency induced by the COVID-19 vaccines and even causing immune escape [99].
As a live attenuated TB vaccine with a history of 100 years, the BCG vaccine has saved countless lives [100]. However, BCG induced protection can only defence M. tuberculosis infection on infants and children rather than adults, and its protective efficacy maintaines 10–15 years. Fortunatelly, previous studies have shown that BCG can induce trained immunity, which has a specific preventive and protective effect against respiratory virus infection, including SARS-CoV-2 [101, 102]. Compared with COVID-19 specific vaccines, BCG vaccine has several significant advantages, such as better safety, matured production technologies, low cost, acceptable storage condition, easy to transport, and high accessibility. If this hypothesis can be confirmed by clinical trials in the future, BCG may be a useful tool to fight against COVID-19. Thus, this review focuses on 56 clinical trials conducted to evaluate the preventive effect of BCG against COVID-19, which provides new strategies and ideas for exploring and resolving challenges faced by the COVID-19 vaccines. Furthermore, this review highlighted the prime-booster strategy of the BCG and COVID-19 vaccine and found that prior BCG vaccination significantly increased neutralizing antibody levels induced by the influenza vaccine [103]. In addition, Netherlands’ clinical trial EUCTR2021-000182-33-NL was also conducted to confirm the prime-booster strategy of the BCG and COVID-19 vaccine. Furthermore, we suggest that the interpretation of the data from these clinical trials should be more cautious and objective. The early termination analysis data of the EUCTR2020-001376-15-DE clinical trial in Germany preliminarily show that the VPM1002 vaccine has a certain protective effect on preventing COVID-19. However, the sample size of this clinical trial is small, the reliability of the results is low, and its effectiveness needs to be further verified.
COVID-19, Coronavirus disease 2019; SARS-CoV-2, severe acute respiratory syndrome coronavirus 2; BCG, Bacillus Calmette-Guerin; CET, central European time; CFUs, colony-forming units; EUA, emergency use authorization; VOC, Variant of Concern; VOI, Variant of Interest; ACE2, angiotensin converting enzyme 2; HCWs, healthcare workers; HRPs, High-Risk Participants; i.d., intradermal injection; s.c., subcutaneous injection.
Not applicable.
All data generated or analyzed during this study are included in this published article.
Conceptualization—WPG and HMW; Data curation—JW and QZ; Formal analysis—JW and QZ; Funding acquisition—WPG; Methodology—JW and QZ; Software—JW, QZ, and WPG; Writing — original draft—JW and QZ; Writing — review & editing—WPG and HMW.
Not applicable.
Not applicable.
This study was funded by the Beijing Municipal Science & Technology Commission (Grant No. 19L2065, 7212103 and Z181100001718005).
The authors declare no conflict of interest. The funders had no role in the design of the study; in the collection, analyses, or interpretation of data; in the writing of the manuscript, or in the decision to publish the results.