Academic Editor: Graham Pawelec
Purpose: This study investigated the association of
serum lipid peroxidation (LPO) and glutathione peroxidase 4
(GPx4) with gestational diabetes mellitus (GDM) and metabolic abnormalities in
Chinese pregnant women. Methods: The present case-control study was
matched at a ratio of 1:1, and it recruited 132 pairs of participants at 24–28
gestational weeks. The serum LPO and GPx4 level were determined in each subject
by enzyme-linked immunosorbent assay. The associations of LPO and GPx4 with
metabolic parameters were analyzed. Thereafter, this study classified all
subjects based on metabolic abnormality frequency (including body mass index,
blood pressure, triglycerides, and fasting plasma glucose), and explored the
association of the serum LPO and GPx4 levels in relation to metabolic
abnormalities and clinical outcomes. Simultaneously, logistic regression analysis
was used to estimate the odds radio (OR) and 95% confidence interval (CI)
expressing the association between LPO/GPx4 and metabolic abnormalities.
Results: Women with gestational diabetes mellitus (GDM) in second
trimester displayed an increased LPO concentration, whereas the GPx4
concentration was decreased compared with normal subjects (174.58
Gestational diabetes mellitus (GDM) is an aberrant pathophysiological alteration in glucose metabolism among gestational women. Throughout the pregnancy, hyperglycemia may cause oxidative stress (OS) in both the fetus and the mother, which has important effects on pregnancy as well as normal childbirth, and is associated with poor fetal outcome, including giant infant, fetal distress, and an increased incidence of congenital aplasia. Globally, the morbidity in GDM continues to increase, particularly in developing countries, including India, China, and in Africa, and it thus receives considerable attention [1, 2].
In a recent meta-analysis that included 79,064 pregnant women, the GDM incidence
was reported to be 14.8% in mainland China [3]. Currently, the pathophysiology
of GDM remains strongly debated. GDM generally results from
Oxidative stress frequently occurs during pregnancy; upon the increased OS level, antioxidant defenses may also be altered [10]. OS involves DNA oxidation/impairment, protein damage, and lipid peroxidation. Antioxidants and oxidants are widely investigated in T2DM as well as the related complications [11]. However, there are few data on GDM, which shares similar pathophysiology with T2DM.Some studies on GDM report increased lipid peroxidation products and reduced activities of antioxidant enzymes [12], even though there are no consistent findings. Lipid peroxidase (LPO) are produced through radical attack on the phospholipid polyunsaturated fatty acid (PUFA) residues, which later react with redox metals and eventually produce the carcinogenic and mutagenic 4-hydroxynonenal, malondialdehyde (MDA), together with additional exocyclic DNA adducts (propano and/or etheno adducts) [13, 14]. With regard to selenoperoxidase, glutathione peroxidase 4 (GPx4) plays an important role in the anti-peroxidant defense. Combined results regarding the enzymological mechanisms of GPx4 and LPO suggest that ferrous iron-generated alkoxyl radicals from lipid hydroperoxide derivatives initiate LPO, and their associated trace elements are normally seen in aerobic metabolism [15].
A recent study found increased oxidative stress (e.g., LPO level) and decreased antioxidative defense (e.g., glutathione peroxidase [GPx] concentration) among women suffering from late-onset GDM, which might have important clinical significance in the pregnancy course and/or pathogenesis among such women [16]. Currently, no consensus has been reached about the relationship of the serum levels of GPx4 or LPO with GDM. In relation to prior work concerning the association of LPO with GDM, the present study determined the second trimester serum GPx4 and LPO concentrations. Considering the earlier detection time, it may be of greater significance in predicting and controlling GDM. The present study focused on exploring the possible associations of GPx4 and LPO with metabolic abnormalities apart from hyperglycemia during the progress of pregnancy.
At 24–48 gestational weeks, each participant received an oral glucose tolerance
test (OGTT). In brief, after fasting for 10 h or more, the women received the
75-g OGTT. GDM was classified according to the World Health Organization (WHO)
[17] endorsed International Association of Diabetes and Pregnancy Study Groups
[18] (fasting plasma glucose (FPG)
(a) The inclusion criteria were as follows:
(1) aged 20–40 years;
(2) planning to receive regular prenatal examinations and deliver at Shengjing Hospital;
(3) diagnosed with GDM at the 24–28 gestational weeks.
(b) Participants conforming to any one of the following criteria were excluded:
(1) no available data on the last menstrual period;
(2) with fetal growth restriction, fetal malformation, or stillbirth history, or
those with
(3) receiving assisted reproductive technology;
(4) with surgical or medical disorders;
(5) hepatitis B virus, human immunodeficiency virus, or syphilis carrier;
(6) diagnosed with gestational complications in addition to GDM;
(7) the use of insulin therapy or hypoglycemic drugs;
(8) with autoimmune disease, thyroid disease, tumors or hematopathy among others.
The NGT gestational women (NGTGW) did not receive topical or systemic medication, and had no underlying systemic disease. The NGTGW were recruited during their second trimester at the Out-patients Clinic, Department of Obstetrics and Gynecology, Shengjing Hospital of China Medical University between January 2019 and July 2021. During this period, we identified 677 gestational women who received periodic systematic examinations at the outpatient department and were undergoing comprehensive routine inpatient prenatal care, all of whom had no abnormality in physical or OGTT examinations, and had similar exclusion criteria (as the above-mentioned in GDM) matched with those in the GDM group. Informed written consent was obtained from all 649 NGTGW before participating in this study. Fig. 1 displays the participant screening and recruitment procedure.
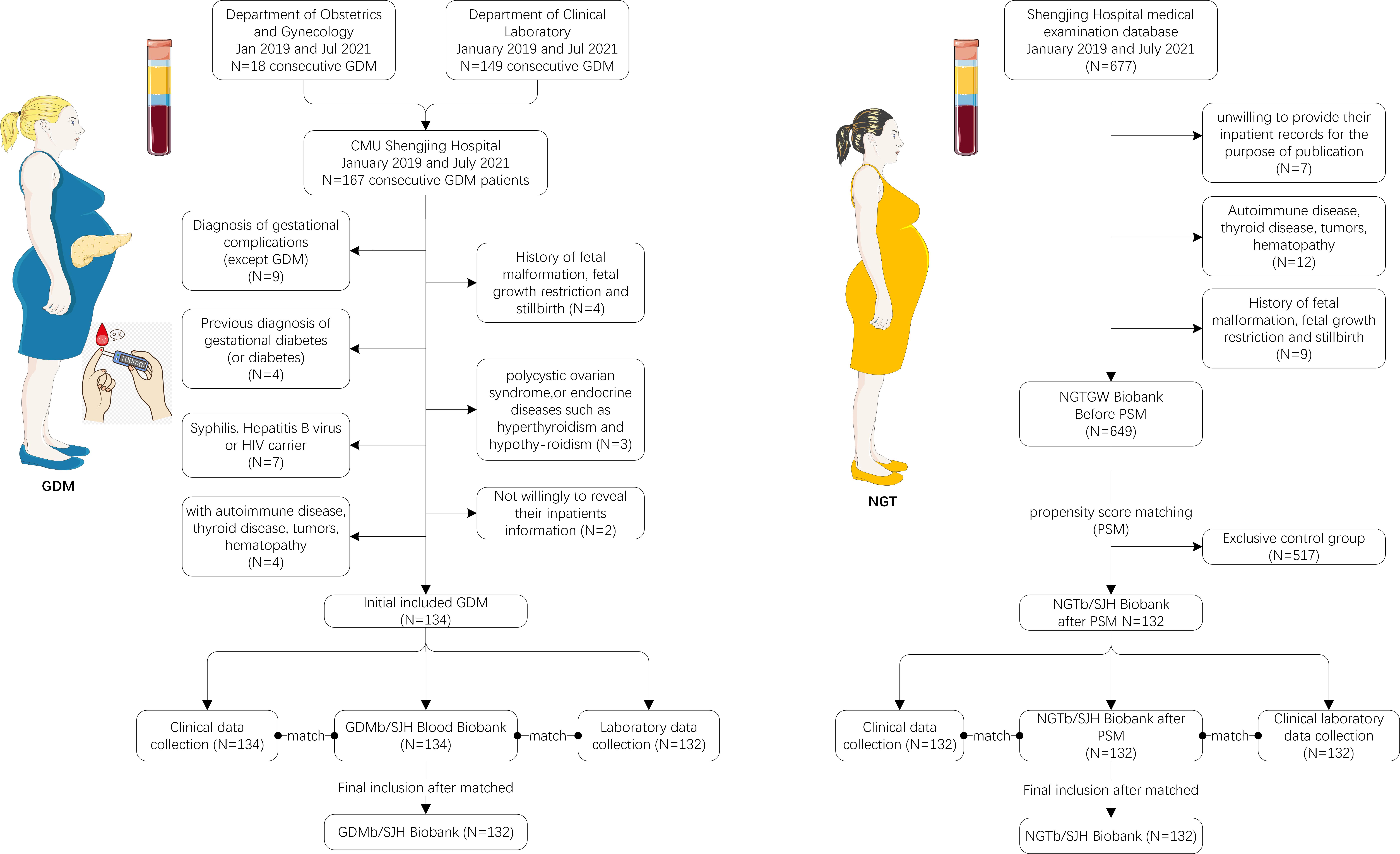
Flowchart displaying the patient and blood sample screening procedures of Shengjing Hospital of blood samples for the Gestational Diabetes Mellitus Blood sample from the Shengjing Hospital Biobank (GDMb/SJH) and for the Normal Glucose Tolerance gestational women Blood sample from the Shengjing Hospital Biobank (NGTb/SJH).
This study adopted the propensity score matching (PSM) approach
to reduce bias in case control selection and to reduce the candidate clinical
confounding factors at the matching ratio of 1:1 [20]. Eventually, 132 eligible
NGT cases were collected based on PSM, and additional analyses were performed to
match with the GDM group. Each participant and the corresponding clinical
measurements were previously presented [21]. In total, 132 pairs of GDM cases and
controls in their second trimester were recruited based on age (years),
gestational week (weeks), and pregestational body mass index (BMI, kg/m
Finally, the present case-control study recruited 264 pregnant women (132 GDM
and 132 normal glucose tolerance (NGT) who were in their second trimester at the
out-patients Clinic, Department of Obstetrics and Gynecology, Shengjing Hospital
of China Medical University in Shenyang, China, between January 2019 and July
2021. The majority (approximately 80%) of pregnant women living in this region
(latitude 123
After excluding the unqualified participants, we eliminated another 25
participants because they were unwilling to provide their inpatient records for
the purpose of publication. We included clinical information and blood samples,
respectively (Fig. 1). Finally, blood was sampled from 132 eligible GDM patients
for further analysis of the Biobank that was registered as the Gestational
Diabetes Mellitus Blood sample from the Shengjing Hospital Biobank (GDMb/SJH). At
the same time, 132 blood was sampled from 649 NGTGW, and registered as the Normal
Glucose Tolerance gestational women Blood sample from the Shengjing Hospital
Biobank (NGTb/SJH). After admission, each eligible participant received an
intravenous puncture for blood collection into 5.0 mL silica/gel plastic tubes
(SST BD Vacutainer® gold) and 5.0 mL anticoagulant EDTA plastic
tubes (BD Vacutainer® lavender). Additionally, the plasma was
extracted from the sampled blood by centrifugation, which was stored at –80
Serum LPO and GPx4 levels were determined using an enzyme-linked immunosorbent
assay kit (Enzyme-linked Biotechnology Co., Ltd. Shanghai, China) according to
the manufacturer’s instructions. This assay was highly sensitive to LPO
(ml856631), and the range detectable concentration was 0.1–32.0 ng/mL for human
LPO. In addition, this assay was also highly sensitive for GPx4 (ml060706), and
the detectable concentration range was 1.0–200.0 ng/mL for human GPx4. The
coefficients of variation (CV) in the inter-assay and intra-assay were
All participates (132 GDMs and 132 NGTs) were in their third trimester and planned to attend regular visits for prenatal examinations and delivery at the In-patients Clinic, Department of Obstetrics and Gynecology of Shengjing Hospital. Their clinical measurements (In-patient Clinic) in the third trimester were collected, including blood routine test, hepatic panel, lipid panel, blood coagulation function, renal function, and serum electrolyte, and the selective solubilization approach are summarized in Supplementary File 1. Blood tests were completed by the Department of Laboratory Medicine of Shengjing Hospital of China Medical University. After extracting the related clinical data, we constructed the related blood database, followed by implementation of laboratory tests. We also collected maternal clinical information, including delivery mode and gestational weeks at birth. In addition, we extracted neonatal outcomes from birth records, which included body length (cm) and birth weight (g).
We applied the PSM approach in this study to reduce the selection bias of
case-controls and to reduce the possible clinical confounding factors at the
matching ratio of 1:1. In addition, the model was evaluated by the
Hosmer-Lemeshow degree of fitting for the C-statistic test and logistic
regression. Prior to PSM, we compared continuous variables in patient clinical
features with controls by the Student’s t-test, and compared categorical
variables using the chi-squared test. Following PSM, we inspected the variable
normality visually. The Mann-Whitney U-test was conducted for comparing
continuous variables, whereas differences among groups were compared by the
Kruskal-Wallis H test. The chi-squared test was conducted to compare categorical
variables. In addition, the associations of GPx4 and LPO with clinical parameters
were evaluated by multiple linear regression and Spearman’s correlation analyses.
This study adjusted confounders to conduct multiple regression analysis,
including age, smoking status, blood pressure (BP), and BMI before pregnancy.
Related thresholds were determined by logistic models, receiver operating
characteristic (ROC) curves, and area under the ROC curve. Subsequently, the
lower and upper strata of the LPO/GPx4 concentrations were determined
accordingly. This study compared the LPO/GPx4 concentrations and the pregnancy
outcomes of both groups (e.g., lower strata of LPO as the reference group and
upper strata of LPO as the case group). Thereafter, this study divided the
subjects based on metabolic abnormality numbers (which included blood lipids, PG,
and BP) as the one metabolic abnormality,
Table 1 displays the clinical features of the 649 NGT subjects and 132 GDM cases. Among them, BMI, age, ethnicity, smokers, and hypertension showed statistical significance between the two groups prior to PSM to reduce the selection bias and possible clinical confounding factors. We subsequently compared the 132 pairs of GDM and controls using PSM by logistic regression. p = 0.524 and p = 0.781 were obtained upon Hosmer-Lemeshow goodness of fit test and C-statistic test, respectively. Following PSM, we excluded those heterogeneities in clinical features between the two groups. Clinical features of the GDM cases and NGT participants is elaborated in Table 1.
Full cohorts | After PSM | |||||
NGTWG | GDM | p value | NGTb | GDMb | p value | |
649 | 132 | 132 | 132 | |||
Age (Years) | 29.4 |
30.8 |
0.213 | 31.2 |
30.8 |
0.637 |
Ethnicity | 1.000 | 0.871 | ||||
Han | 528 (81.4) | 108 (81.8) | 110 (83.3) | 108 (81.8) | ||
Others | 121 (18.6) | 24 (18.2) | 22 (16.7) | 24 (18.2) | ||
Pre-pregnancy BMI (kg/m |
23.8 |
24.4 |
0.170 | 25.1 |
24.4 |
0.454 |
Family history of hypertension | 17 (2.6) | 4 (3.0) | 0.768 | 2 (1.5) | 4 (3.0) | 0.684 |
Family history of DM | 13 (2.0) | 6 (4.5) | 0.113 | 8 (6.0) | 6 (4.5) | 0.785 |
Smokers | 10 (1.5) | 3 (2.2) | 0.469 | 4 (3.0) | 3 (2.2) | 1.000 |
SBP (mmHg) | 106.4 |
111.9 |
0.006 | 108.1 |
111.9 |
0.184 |
DBP (mmHg) | 67.1 |
71.9 |
0.048 | 70.4 |
71.9 |
0.241 |
HR (bmp) | 78.3 |
81.5 |
0.052 | 79.5 |
81.5 |
0.665 |
OGTT time (week) | 24.6 |
24.3 |
0.525 | 24.8 |
24.3 |
0.693 |
FFG (mmol/L) | 4.7 |
5.6 |
4.5 |
5.6 |
||
1 h PG (mmol/L) | 9.6 |
11.4 |
9.4 |
11.4 |
0.025 | |
2 h PG (mmol/L) | 8.3 |
9.4 |
0.002 | 8.7 |
9.4 |
0.042 |
Gravidity | 0.036 | 0.620 | ||||
1, n (%) | 341 (52.5) | 56 (42.4) | 61 (46.2) | 56 (42.4) | ||
2, n (%) | 175 (27.0) | 37 (28.0) | 40 (30.3) | 37 (28.0) | ||
3, n (%) | 133 (20.5) | 39 (29.6) | 31 (23.5) | 39 (29.6) | ||
Note, values are mean |
According to the WHO guidelines released in 2013, all subjects were assigned to
the NGT or the GDM group. Fig. 2A exhibits the serum LPO concentrations of both
groups. The GDM group displayed a markedly increased LPO concentration relative
to the NGT group (174.58
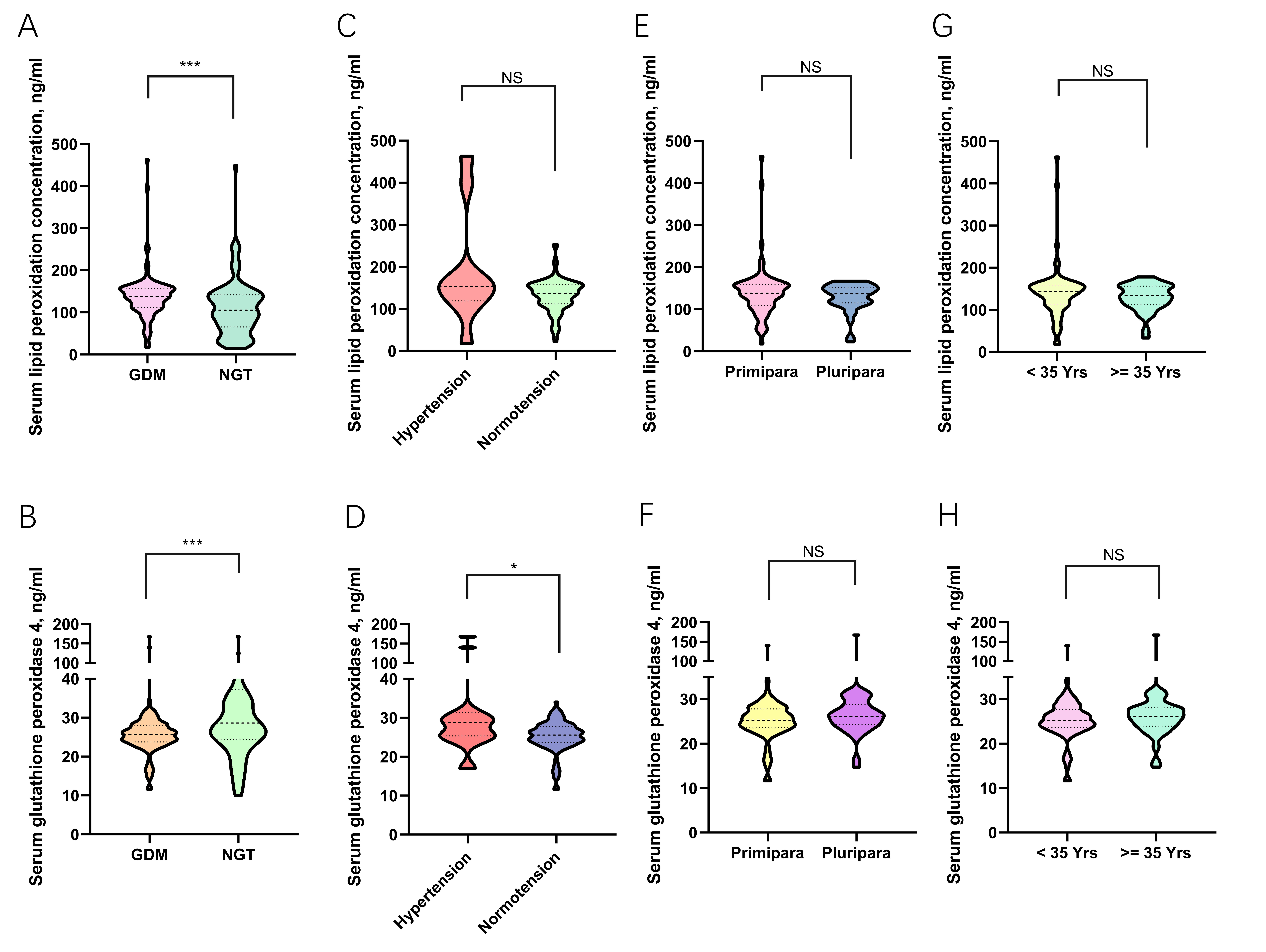
The LPO (A) and GPx4 (B) concentrations of the GDM and
NGT groups at 24–28 gestational weeks. Subgroup analysis between family history
of hypertension and normotension (C,D), between the primipara and pluripara
(E,F), and between those aged
Fig. 2C–F present subgroup analyses of the GDM patients. The serum LPO
concentrations were not statistically significantly different between
a family history of hypertension and normotension (Fig. 2C).
The GPx4 level in the GDM without hypertension group was significantly elevated
compared with the GDM with a family history of hypertension group (49.50
AUC | 95% CI | p value | Cut-off | Sensitivity (%) | Specificity (%) | |
LPO (ng/mL) | 0.623 | 0.539–0.707 | 0.008 | 160.54 | 0.437 | 0.813 |
GPx4 (ng/mL) | 0.607 | 0.520–0.694 | 0.044 | 24.81 | 0.415 | 0.770 |
LPO+GPx4 (ng/mL) | 0.643 | 0.560–0.727 | 0.042 | 0.495 | 0.780 |
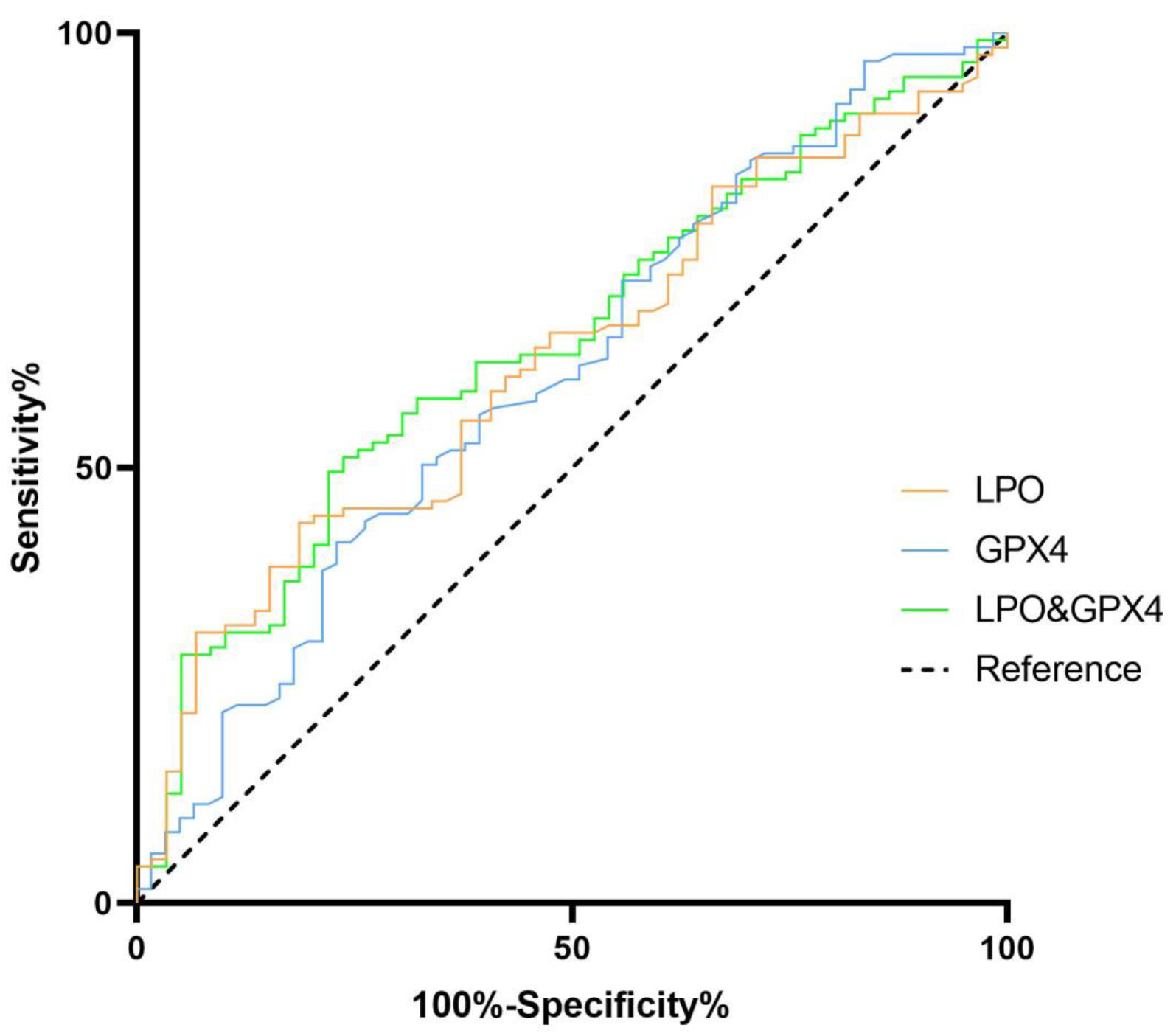
ROC analysis of the LPO and GPx4 levels for the evaluation of the GDM population. The vertical axis represents the sensitivity and the horizontal axis represents the 1-specificity.
As presented in Table 3, patient features and anthropometric variables were
analyzed according to their serum GPx4 and LPO concentrations (classified as four
quartiles). As revealed by the Kruskal-Wallis H test, as the GPx4 concentration
was elevated, differences in the percentage glycated albumin (GA%), FPG, and
2h-PG were significant between the different groups, and vice
visa (p = 0.004, p = 0.028, and p = 0.010,
respectively) (Fig. 4). By contrast, the Kruskal-Wallis H test analysis showed
that with the rise in LPO, there were no significant differences in glucose
metabolism-related indicators within the GDM group (Table 2). Using bivariate
correlation, this study analyzed the association of the GPx4 concentration with
glucose metabolic parameters of the GDM group. The serum GPx4 level was
significantly negatively correlated with the FPG (r = –0.237, p =
0.004), 2h-PG (r = –0.258, p = 0.040), and GA% (r = –0.395, p
LPO | Quartile1 | Quartile2 | Quartile3 | Quartile4 | p value |
( |
(111.79–137.91 ng/mL) | (137.92–157.76 ng/mL) | ( | ||
n | 33 | 33 | 33 | 33 | |
Age, Years | 33.6 |
33.4 |
32.1 |
33.9 |
0.540 |
FPG, mmol/L | 5.2 |
5.7 |
5.3 |
5.5 |
0.404 |
1h-PG, mmol/L | 10.7 |
10.8 |
10.8 |
12.3 |
0.837 |
2h-PG, mmol/L | 9.3 |
9.0 |
8.4 |
9.2 |
0.739 |
FINS, mU/L | 13.3 |
12.3 |
15.6 |
35.0 |
0.687 |
Glycosylated hemoglobin (HbA1c), % | 5.6 |
5.6 |
5.8 |
5.6 |
0.270 |
GA, % | 12.1 |
10.6 |
13.3 |
10.8 |
0.459 |
GPx4 | Quartile1 | Quartile2 | Quartile3 | Quartile4 | p value |
( |
(23.869–25.898 ng/mL) | (25.898–27.890 ng/mL) | ( | ||
n | 33 | 33 | 33 | 33 | |
Age, Years | 33.2 |
33.1 |
33.1 |
33.6 |
0.948 |
FPG, mmol/L | 5.9 |
5.4 |
5.3 |
5.1 |
0.028 |
1h-PG, mmol/L | 12.5 |
11.3 |
10.6 |
10.5 |
0.409 |
2h-PG, mmol/L | 10.1 |
10.0 |
8.5 |
8.3 |
0.010 |
FINS, mU/L | 11.7 |
19.7 |
13.1 |
18.6 |
0.229 |
Glycosylated hemoglobin (HbA1c), % | 5.4 |
5.7 |
5.5 |
5.8 |
0.233 |
GA, % | 15.9 |
13.0 |
12.1 |
9.1 |
0.004 |
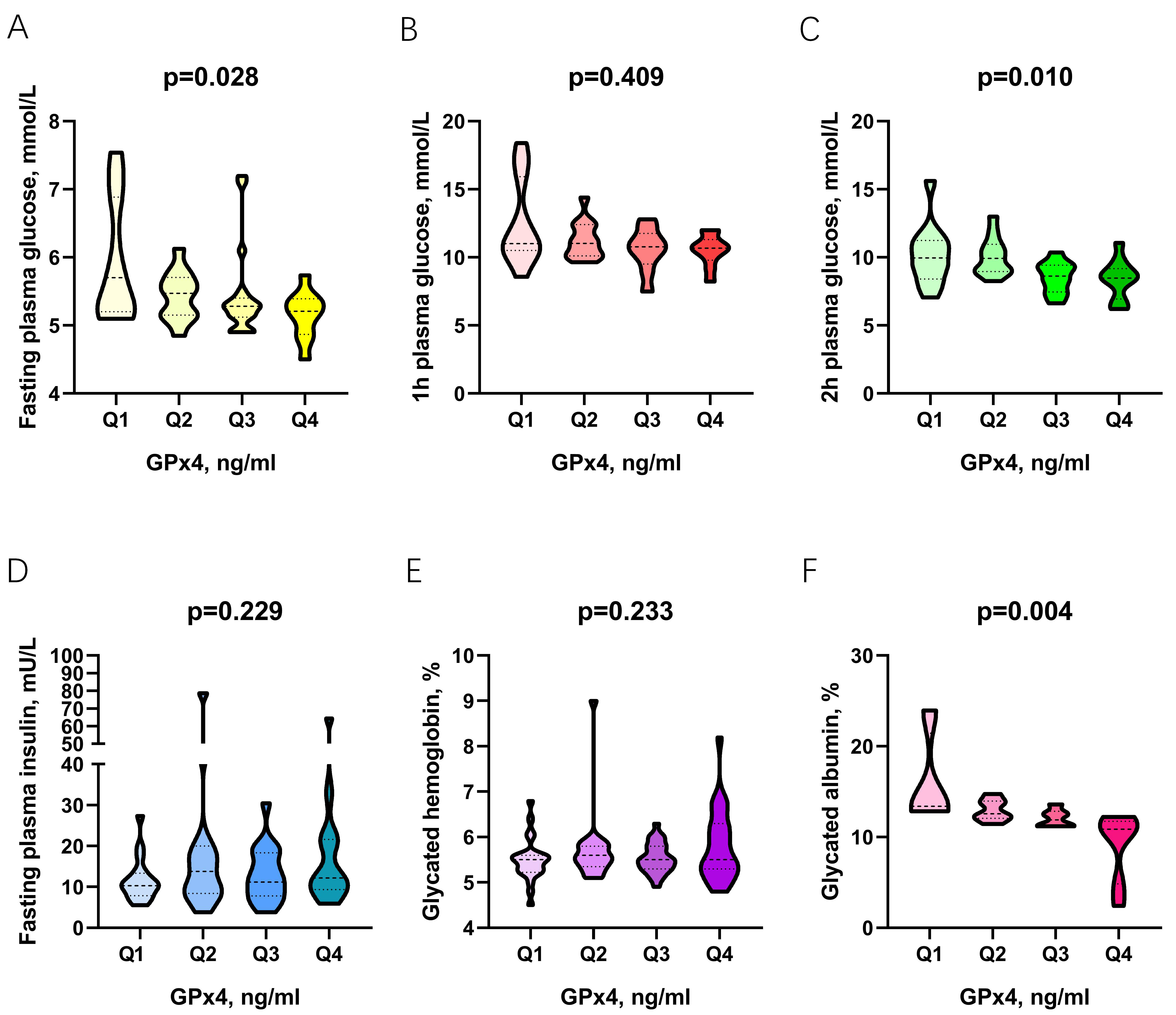
The relationship between GPx4 and fasting plasma glucose (A), 1h-PG (B), 2h-PG (C), fasting plasma insulin (D), glycated hemoglobin (E), and glycated albumin (F).
Table 3 presents the clinical outcome features for each subject. Using LPO =
160.54 ng/mL in the second trimester as the threshold, this study classified GDM
cases into LPO upper and lower strata groups. We found that GDM patients in the
second trimester with a high LPO level were more likely in the third trimester to
have higher levels of total bilirubin, direct bilirubin, unconjugated bilirubin,
and serum creatinine (all p
In addition, multivariate logistic regression was conducted.
Supplementary Table 1 presents the associations of metabolic syndrome
components with the upper stratum of the LPO and GPx4 levels when compared with
the lower stratum, according to Chinese GMS diagnostic criteria. After the GDM
subjects were grouped based on the metabolic abnormality component, the metabolic
abnormality risk was found to be elevated with increased LPO concentration
(elevated DBP, OR = 1.04, p = 0.048; and high TGs, OR = 2.19, p

The correlations between the LPO and GPx4 levels and the clinical features of the blood routine (A), serum lipid profile (B), blood coagulation function (C), liver function (D), thyroid function (E), and serum electrolyte (F).
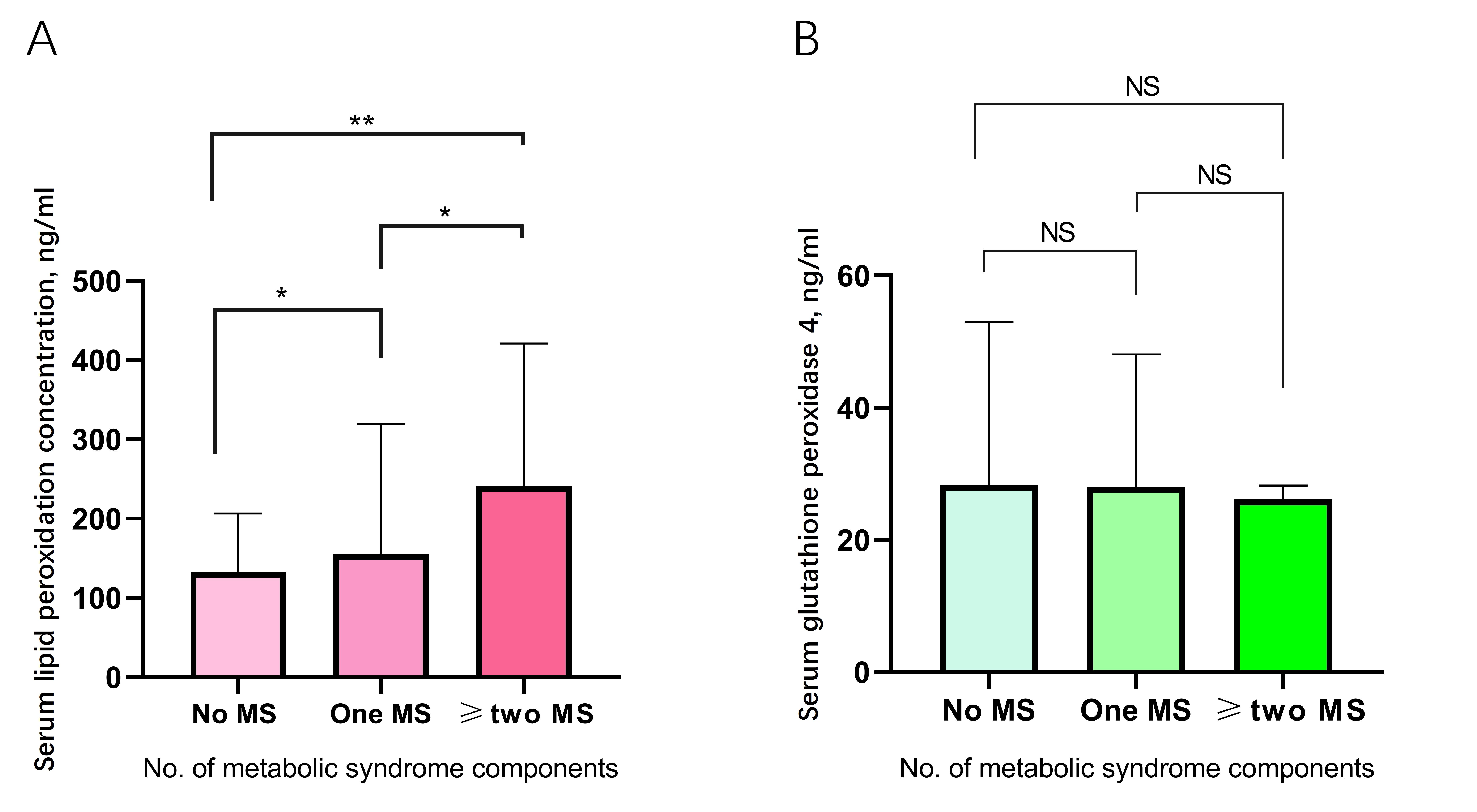
The serum LPO level (A) and GPx4 level (B) in the no metabolic abnormality, one metabolic abnormality, and two or more metabolic abnormalities groups.
The present study investigated the GDM-related risk factors. The results showed that pregnant women who displayed higher LPO concentrations and lower GPx4 concentrations during the 24–28 gestational weeks were at an increased risk for GDM. This study focused on investigating the relationships of the GPx4 and LPO concentrations during the second trimester with blood glucose metabolism and metabolic abnormalities, as well as pregnancy outcomes among the GDM cases.
The studies to date have mostly focused on increased lipid oxidative stress or reduced lipid antioxidant defense, particularly in T2DM patients. For example, Bandeira et al. [23] suggested that lipid peroxidation was markedly increased in the diabetes mellitus (DM) group in comparison with the prediabetic groups and the normotensive and hypertension controls. Based on these findings, lipid peroxidation is related to DM, whether or not there is hypertension. As reported by some studies conducted among T2DM patients, the markers of oxidative damage increased, whereas those of antioxidant defenses decreased, in particular for the T2DM-related complications [24, 25].
In the third trimester, insulin resistance that is increased physiologically may lead to elevated circulating lipids (including free fatty acids [FFAs], total cholesterol, TG, low-density lipoprotein) [10, 26]. At the same time, the up-regulation of lipid OS markers is detected among cases who display various pregnancy-complicating conditions [27]. Numerous studies compared the lipid OS markers between non-pregnant and normal pregnant women [28, 29]. GDM results in a higher risk for macrosomia, together with increased perinatal mortality and morbidity among fetuses, and predicts a greater risk for T2DM among the mothers [30]. In GDM, multiple metabolic defects occur before T2DM develops, therefore, GDM is recognized as a prediabetic state [8]. The pathophysiology of GDM remains strongly debated. Consequently, it is of great significance to develop an efficient approach to predict and prevent GDM. The relationships of T2DM (which shares similar clinical features with GDM) and T2DM-related complications with OS have been extensively investigated. Some studies demonstrated the imbalance between lipid antioxidation and pro-oxidation in GDM and T2DM [31, 32].
Lipid peroxides are produced by polyunsaturated fatty acids
(PUFAs) oxidative degradation, which generates products that exacerbate OS in
tissues and cells. The markers of lipid peroxidation include MDA and
8-iso-prostaglandin F2
Once gestational women were classified as NGT or GDM based on the blood glucose
status, the present study showed that the GPx4 concentration was markedly
decreased in the GDM group compared with the NGT group. Moreover, the GPx4
concentrations among gestational women displayed a linear correlation with the
FPG, 2h-PG, and GA% for glucose metabolism in Chinese pregnant women, even when
adjusted for gestational age and age. In recent years, Krümmel and colleagues
discovered markedly elevated GPx4 concentrations within
The present study had some limitations. Firstly, there was a small sample size, which had some impact on the relationship of serum LPO and GPx4 concentrations with GDM occurrence. Secondly, this study determined LPO and GPx4 contents only in the second trimester. We did not perform dynamic monitoring of the of LPO or GPx4 concentrations or determine their clinical values in the third trimester. Thirdly, when collecting data, the time the LPO concentration was determined were inconsistent with that for the biochemical examination in some subjects (differences of up to 3 weeks), thus, potentially resulting in numerical differences and compromising the model interpretability.
In summary, the present study suggested that GPx4 played a vital role in glucose metabolism and that LPO was a possible risk factor associated with the metabolic abnormalities in the Chinese pregnant women. An increased serum LPO concentration might predict the incidence and degree of metabolic abnormalities among the Chinese gestational diabetes mellitus population in the future. To date, limited research has been conducted on the associations of serum GPx4 and LPO concentrations with GDM, such that more evidence-based studies are warranted. The small sample size and restricted geographical distribution were potential limitations in the present study. Consequently, a multi-area and multi-center prospective study with a large sample size should be conducted to illustrate the associations of serum LPO/GPx4 with GDM.
BMI, body mass index; SBP, systolic blood pressure; DBP, diastolic blood pressure; TP, total protein; ALT, alanine aminotransferase; AST, aspartate aminotransferase; ALP, alkaline phosphatase; ALB, albumin; GGT, gamma-glutamyl transpeptidas; PA1, pre-albumin; BilT, total bilirubin; CB, conjugated bilirubin; UCB, un-conjugated bilirubin; TBA, total bile acid; FPG, fasting plasma glucose; TG, triglyceride TC, total cholesterol; HDL-C, high-density lipoprotein-cholesterol; LDL-C, low-density lipoprotein-cholesterol; ApoA1, apolipoprotein A1; AopB, apolipoprotein B. WBC, white blood cells; RBC, red blood cells; HGB, hemoglobin; PLT, platelet; BA, basophil; EO, eosinophil; LY, lymphocyte; MO, monocyte; NE, neutrophil; MCH, mean corpuscular hemoglobin; MCHC, mean corpuscular hemoglobin concentration; MCV, mean corpuscular Volume; MPV, mean platelet volume; HCT, hematocrit; PCT, platelet hematocrit; PDW, platelet distribution width; RDW, red blood cell volume distribution width; APTT, activated partial thromboplastin time; PT, prothrombin time; PTA, prothrombin activity; INR, international normalized ratio; CRP, C-reactive protein; PSM, propensity score matching.
BZ and LS was responsible for the study design, collection of blood sample, experiment implementation, study screening, data analysis and interpretation, statistics analysis, and drafting. LS, BZ, and TZ were in charge of research and drafting. SH, BZ, and LS were responsible for data collection and analysis. All authors made contributions to study design and interpretation, and further drafting. LS was the guarantor.
The Medical Ethics Committee of Shengjing Hospital of China Medical University approved our study protocol (Ethics Approval No. 2017PS066K). This study was performed following the Declaration of Helsinki (revised in October 2013, Fortaleza, Brazil). Informed written consent was obtained from each participant before participating in this study.
We thank International Science Editing (http://www.internationalscienceediting.com) for editing this manuscript.
This research received no external funding.
The authors declare no conflict of interest.