†These authors contributed equally.
Academic Editor: Graham Pawelec
Backgrounds: Dental avulsion due to trauma, especially in young
patients, is a worldwide problem, requiring tooth replacement. Delayed
replantation could cause tooth loss when the cementum is severely damaged. A
small number of studies has reported that photobiomodulation (PBM) therapy using
Er: YAG laser irradiation activates cellular signaling responses in different
cell types, resulting in a variety of favorable biological effects. The aim of
this in vitro study was to evaluate the potential biostimulatory effect
of low-level Er: YAG laser irradiation on the biological responses of cultured
mouse cementoblasts (OCCM-30), including the mitogen-activated protein kinases
(MAPKs). Methods: OCCM-30 cells were exposed to 2940 nm Er: YAG laser
irradiation for 15 s at 0.34 W (pulse duration of 100 or 1000
Dental avulsion due to trauma, especially in young patients, is a worldwide problem, which demands tooth replacement by either tooth replantation or placement of dental implants or prosthodontics. It is known that delayed replantation after avulsion may result in tooth loss because of root resorption [1]. So-called replacement resorption or inflammatory resorption is a probable adverse outcome after replantation of a tooth [2]. It is most likely that root resorption happens in cases where periodontal ligament (PDL) and cementum have been severely damaged [2]. To prevent or delay root resorption, the International Association of Dental Traumatology (IADT) has proposed different treatment methods for the root surface of avulsed teeth before replantation [3]. These different root surface treatment modalities include among others fluorides, steroids, sodium alendronate, enamel matrix derivatives, fibroblast growth factor (FGF) [4, 5].
Viable cementoblasts and/or intact molecules associated with these cells are likely to be actively involved in recruiting pre-cementoblasts that will form new cementum which is critical for structurally and functionally re-establishing a sound PDL attachment [6]. Thus, to achieve a successful tooth replantation cellular viability and function of periodontal tissues, such as cementoblasts and PDL, must be preserved [7]. In this sense, photobiomodulation (PBM) therapy applied with low-level lasers could be an adjunctive therapy because PBM improves cellular proliferation, migration, differentiation [8, 9], growth factor secretion [10], among others cellular biological activities [11]. Thus, PBM applied to be root surface could possibly improve the prognosis of tooth replantation. In fact, in recent year, many laser-based root surface treatments, mostly using high-power lasers like erbium-dopped yttrium aluminum garnet (Er: YAG) and neodymium-dopped YAG (Nd: YAG) lasers, have attracted attention [12].
Some studies have reported that Er: YAG laser irradiation, applied with
low-level energies, activates cellular signaling responses in different cell
types resulting in a variety of favorable biological effects [13, 14, 15, 16, 17]. Schwarz
et al. (2004) [13] showed that Er: YAG laser irradiation at an energy
density of 5.1–12.7 J/cm
Low-level Er: YAG laser irradiation enhances osteoblast proliferation through
activation of ERK1/2 [18]. Low-level Er: YAG laser irradiation also improves
proliferation and calcification of osteoblast-like cells [17] as well as
proliferation of cultured human gingival fibroblasts [14]. Finally, it has been
demonstrated that low-level Er: YAG laser irradiation increases prostaglandin E
production via the induction of cyclooxygenase-2 mRNA in human gingival
fibroblasts [15]. Recently, Lin et al. (2021) [19] also reported that
Er: YAG laser irradiation at 4.2 J/cm
OCCM-30 cell line was provided by Prof. M. Somerman (NIH, NIDCR, Bethesda,
Maryland, USA) and cultured according to a previous description [20]. Briefly,
cells were maintained in cell culture growing medium composed by
For inducing cementogenesis and performing mineralization assay, mineralizing
medium composed by 10 mM
The Er: YAG laser apparatus (Cat No.: MCL31 Dermablate; Asclepion Laser
Technologies, Jena, Germany) from the department of Periodontology of the
University of Aachen, emitting at a wavelength of 2940 nm was employed in this
study. In order to equalize the profile of the laser beam, laser irradiation was
performed perpendicularly to the bottom of the culture dish at a distance of
25–30 cm, by means of a handpiece attached to the end of the articulated arm, as
described previously (Fig. 1) [18]. The cultured cells were irradiated just once
without interval. The serum-free medium was removed immediately before
irradiation and all irradiations were performed in the absence of culture medium.
The output parameter settings were determined based on previous findings in the
literature [18] and a pilot study [14]. The irradiations were done using the
pulsed model and 20 Hz on the panel, with an irradiation time of 15 s (Table 1).
The actual energy level reaching at the cell surface was 17 mJ/pulse, resulting
in a total energy density of 2 J/cm
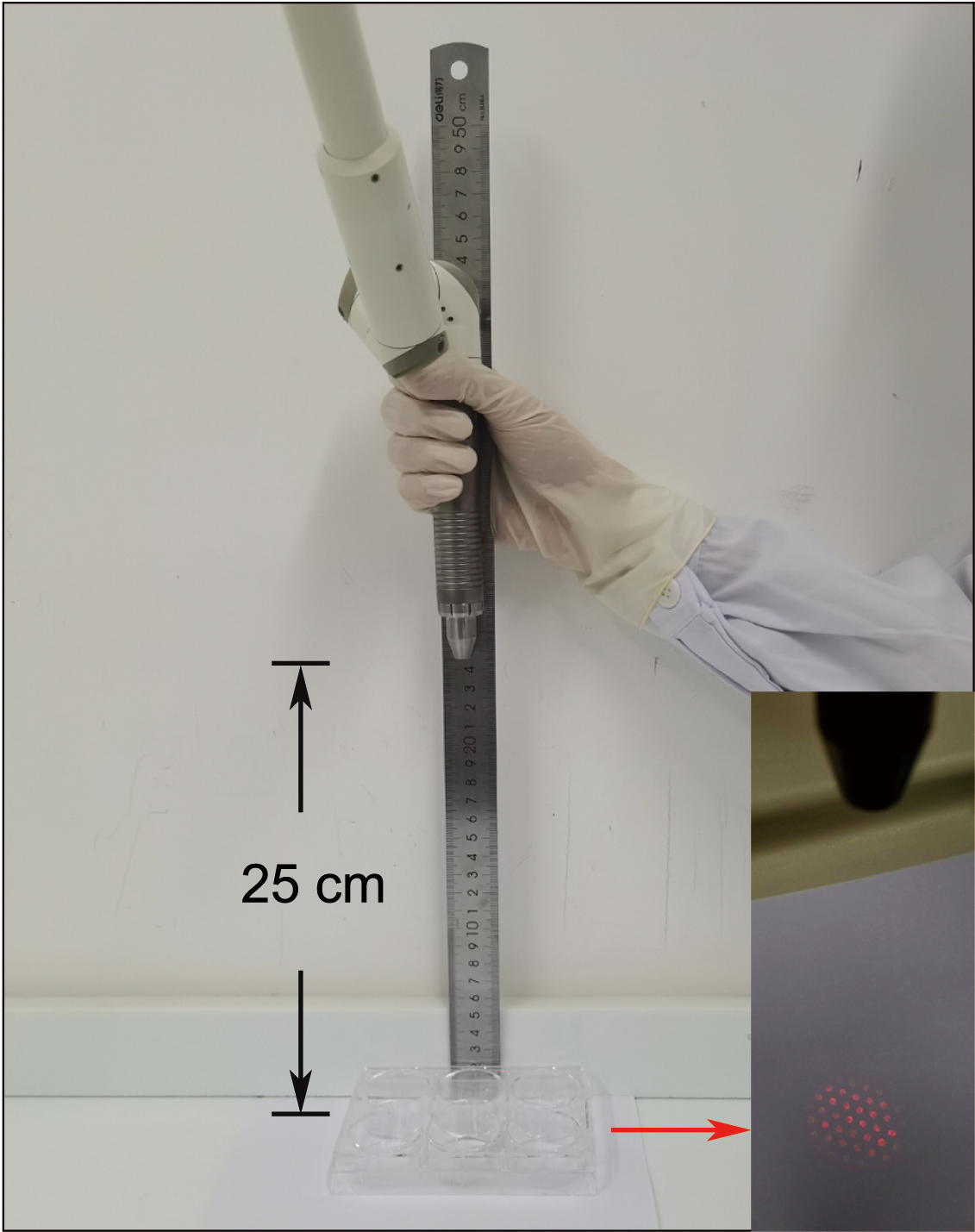
Schematic illustration of the low-level Er: YAG laser irradiation procedure. The Er: YAG laser device was equipped and performed perpendicularly to the bottom of the culture dish at a distance of 25 cm (black arrow). Energy densities were generated in a round homogeneous spot with a diameter of 35 mm (red arrow).
Parameter (units) | Values | |
Wavelength (nm) | 2940 | |
Operating Mode | Pulsed | |
Frequency (Hz) | 20 | |
Pulse Duration ( |
1000 | 100 |
Duty Cycle | 2% | 0.2% |
Spot Area of Tip (cm |
1.69 | |
Cell Area (cm |
9.6 | |
Energy Density (J/cm |
2 | |
Number of irradiation sessions | 1 | |
Intervals between sessions | - | |
Irradiation Time (s) | 15 | |
Power Average (W) | 0.34 | |
Power Peak (W) | 17 | 170 |
Energy Level (mJ/pulse) | 17 |
In order to analyze the cell migration ability, a previously established wound
healing assay was applied. Briefly, OCCM-30 cells were plated at a density of 8
The viability and proliferation of OCCM-30 cementoblasts were examined by using
3-(4,5-dimethylthiazol-2-yl)-5-(3-carboxymethoxyphenyl)-2-(4-sulfophenyl)-2H-tetrazolium
(MTS) reduction assay (CellTiter 96
Cells were kept overnight in starvation medium. Different pulse duration
parameters of Er: YAG laser were used to stimulate cells. Total RNA was isolated
using the NucleoSpin
The SsoAdvanced
OCCM-30 cells were seeded to 6-well plates at a density of 3
Cells were irradiated or not after serum-free starvation overnight. 200
The intensity of phosphorylated MAP kinase (p-MAPK) was measured using the NIH Image J program and normalized to the control group, which was considered as 1.0.
Statistical analysis was performed using the GraphPad Prism 8.0 software
(GraphPad software, California, USA). All values are expressed as the mean
Firstly, we investigated the effect of Er: YAG laser irradiation with a constant
pulse frequency of 20 Hz and irradiation time of 15 s at different energy
densities (1 or 2 J/cm
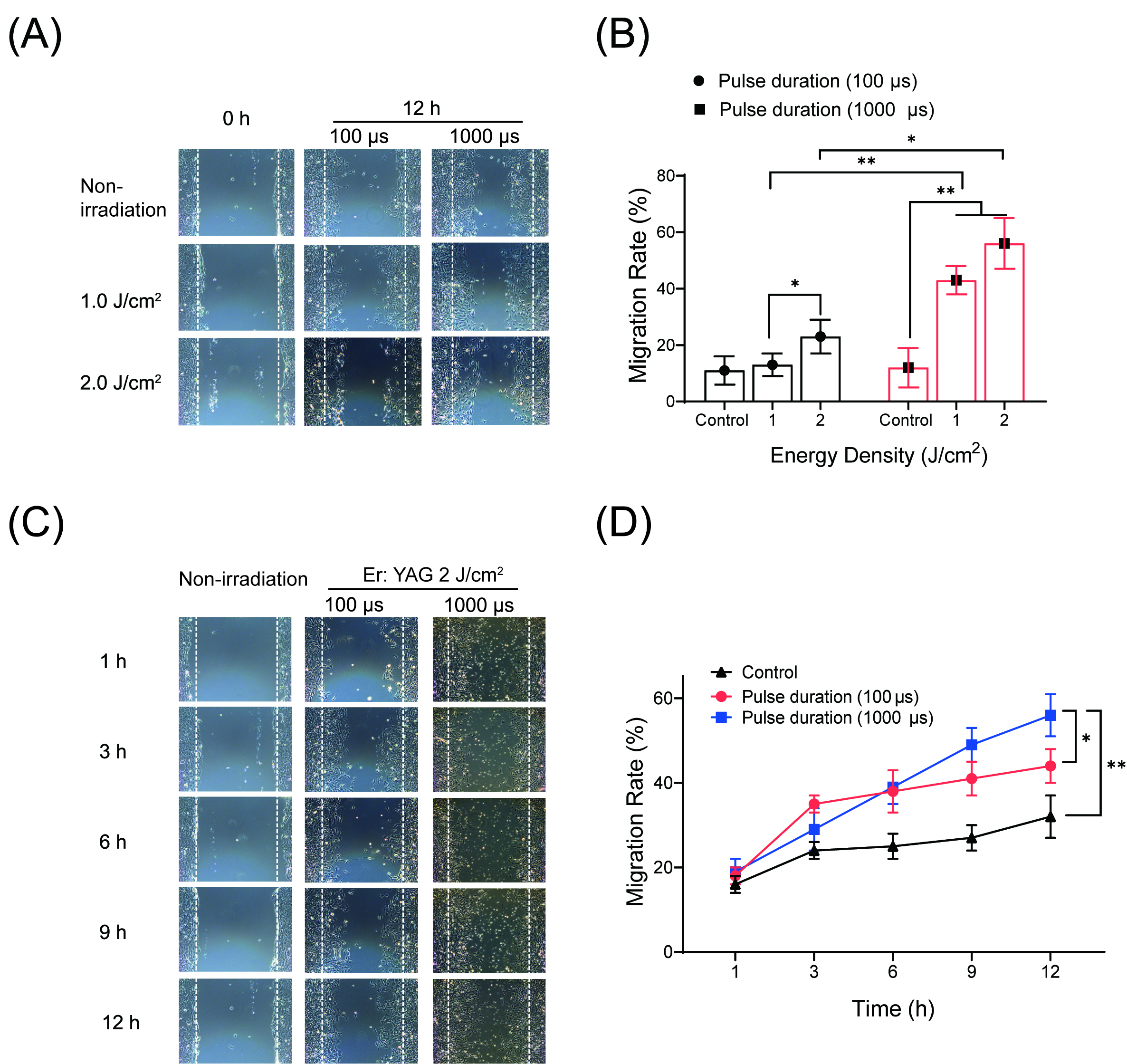
Effects of low-level Er: YAG laser irradiation at 1 and 2
J/cm
Secondly, we followed the migration rate of OCCM-30 cells under the influence of
different pulse durations (100
The effect of different pulse duration (100
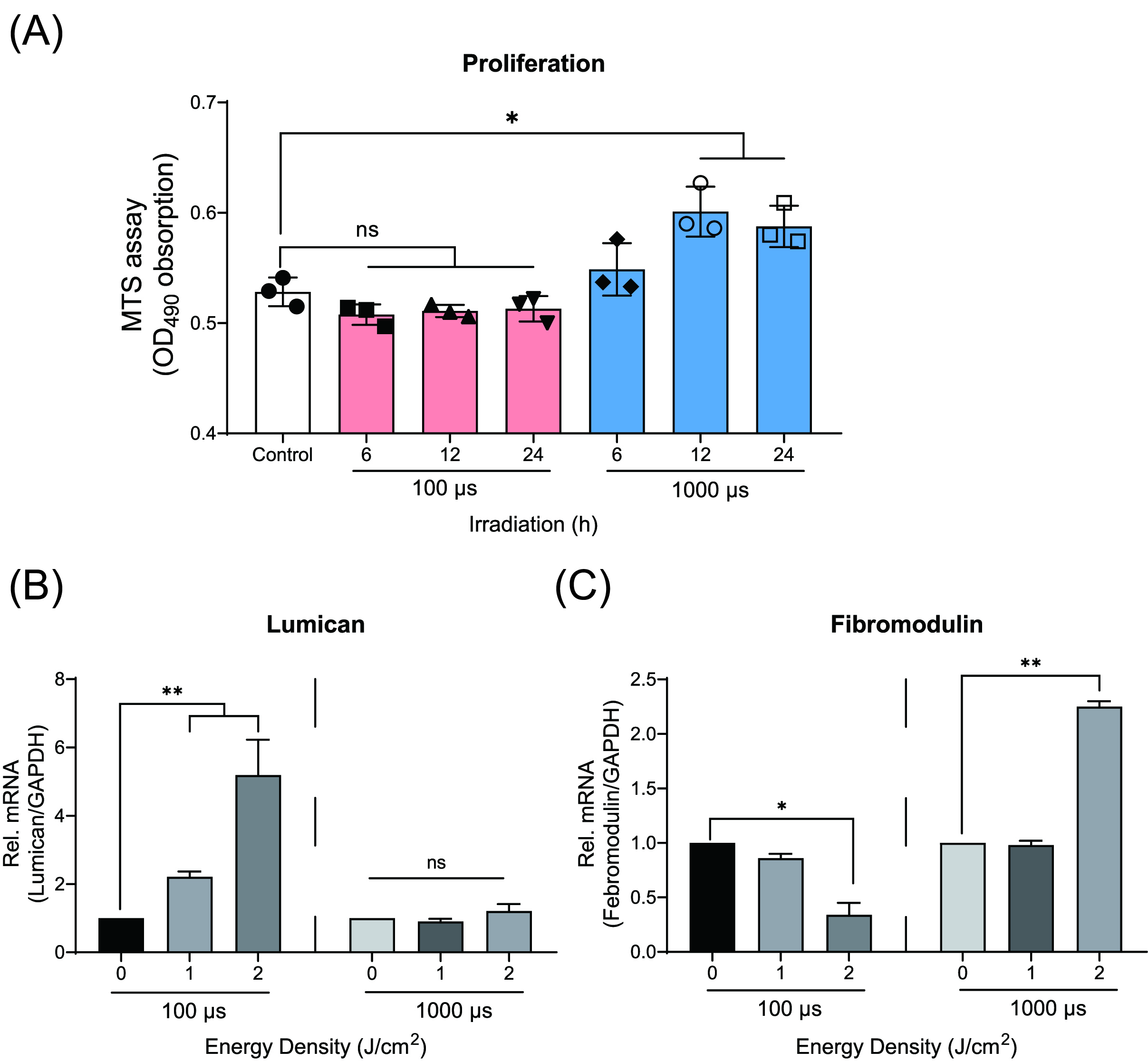
Effects of low-level Er: YAG laser irradiation on proliferation
of cementoblasts. (A) Graphic representation of the MTS assay. The highest
response of OCCM-30 cells to the Er: YAG irradiation at 2 J/cm
The total RNA was collected at 1 and 2 h after laser irradiation. qRT-PCR showed
significant increase of mRNA expression of Lumican (Lum) in
cells irradiated with 100
Next, we performed a mineralization assay to evaluate the effect of different
pulse duration (100
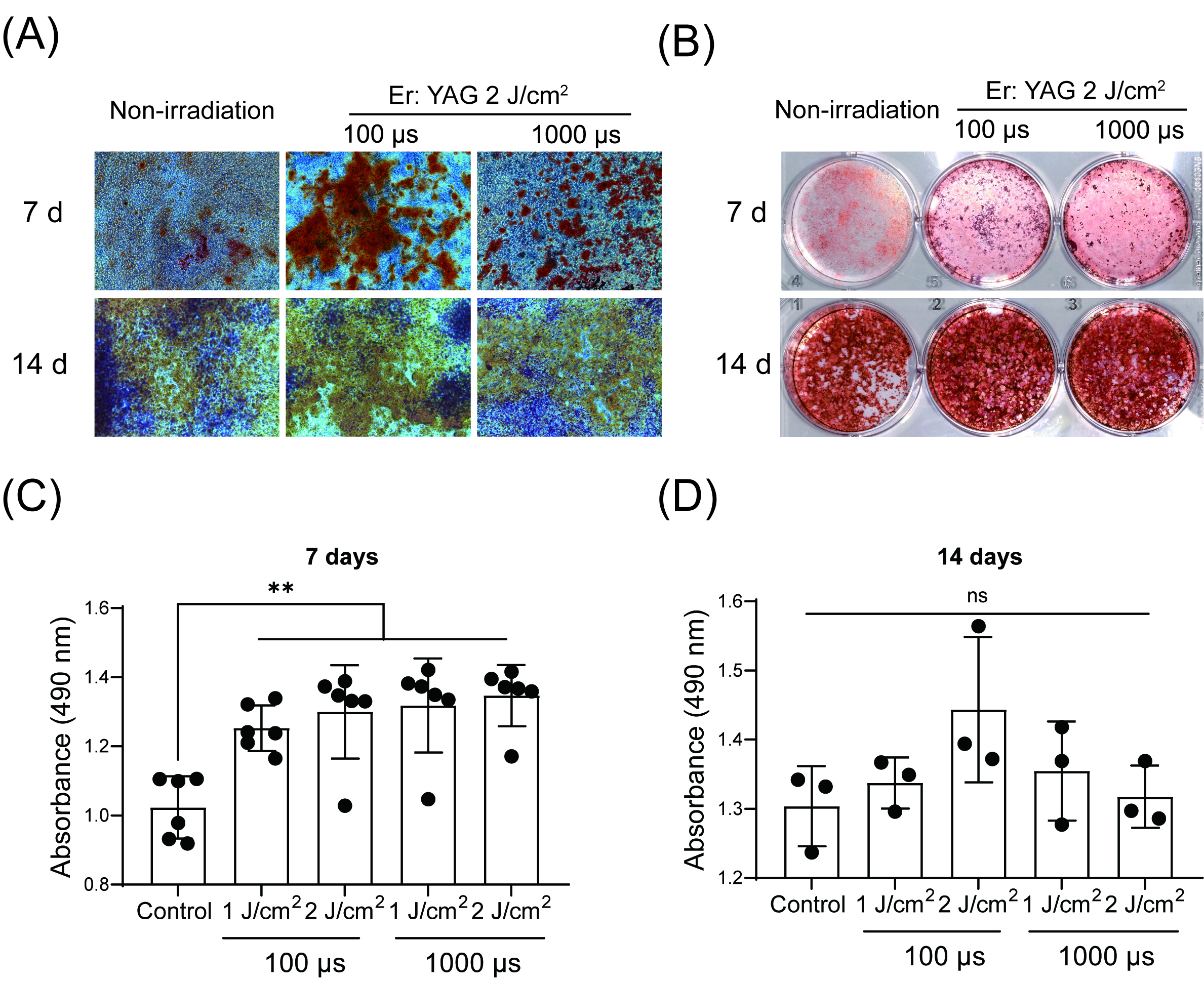
Effects of low-level Er: YAG laser irradiation on mineralization
of cementoblasts. (A) Illustrative phase photomicrographs of OCCM-30 cells
submitted to different pulse duration parameters of Er: YAG irradiation at 2
J/cm
To determine whether or not the MAPK pathway would be involved in the low-level
Er: YAG irradiation effects on the OCCM-30 cells, we checked the expression of
phosphorylated MAP kinase protein. Based on the Western Blot results, irradiation
at both pulse duration was found to enhance ERK1/2, JNK and P38 phosphorylation
as compared to the control as soon as 5 min after irradiation (Fig. 5). These
increased phosphorylation of P38 was observed from 10 to 30 min, with a peak at
10 min, when 100
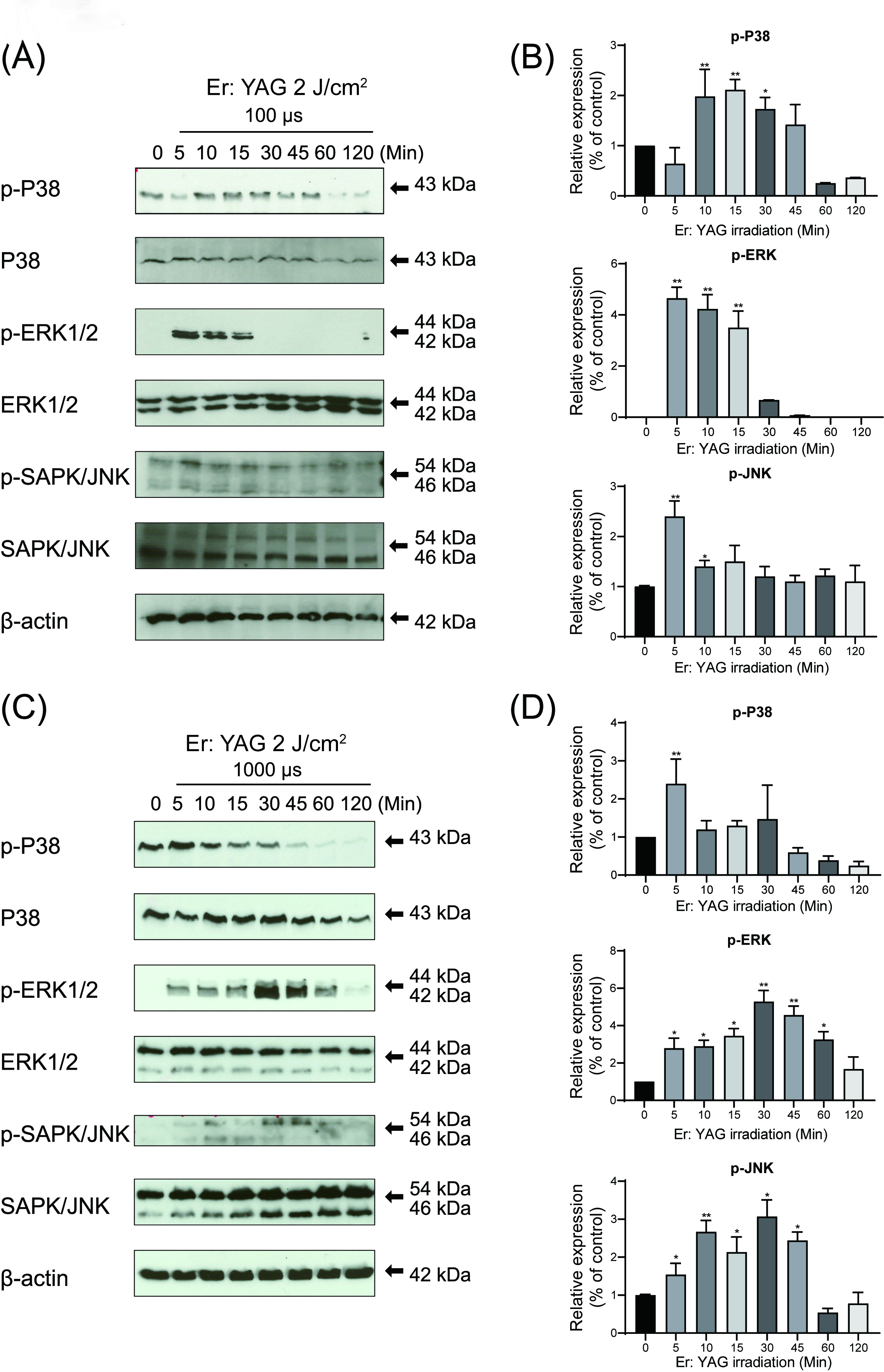
Effects of low-level Er: YAG laser irradiation on the MAP kinase
activation in cementoblasts. (A, C) Photographs of the gels: OCCM-30 cells were
stimulated with 100
In the present study, we demonstrated that low-level Er: YAG laser at specific irradiation parameters improved the migration, proliferation and functional differentiation of OCCM-30 cells along with the regulation of mRNA expression of Lum and Fmod genes. Moreover, low-level Er: YAG laser irradiation activated the MAP kinase signaling pathway of the cultured mouse cementoblasts.
In our study three important parameters were considered, the energy output, pulse repetition rate and irradiation time, which were predetermined according to results of previous studies [18]. An energy output of 170 mJ/pulse was fixed for the Er: YAG laser, and 25–30 cm of irradiation distance was determined based on previous studies [14, 15]. An irradiation time of 15 s and a pulse repetition rate of 20 Hz was selected as the most safe and effective parameter, which is used for hard tissue [25]. After determining the basic setting for laser device, the effects of different pulse duration of low-level Er: YAG laser were evaluated by changing the pulse duration parameter while keeping the other parameters fixed.
Firstly, we demonstrated that OCCM-30 cells irradiated with low-level Er: YAG
laser exhibited an increased proliferation and migration response to 1.0 or 2.0
J/cm
The effects of PBM on different cells and tissues have been studied by several
researchers in vitro and in vivo showing the ability of this
therapy to increase migration, proliferation and also cell differentiation
depending on the applied irradiation parameters [26, 27, 28, 29, 30, 31, 32]. Also, studies were
conducted to investigate the effects of PBM therapy on cell survival at different
time intervals between alveolar tooth avulsion and replantation [33]. For
instance, Kim et al. (2017) [34] reported that PBM with lasers with four
wavelengths (415, 525, 660, and 830 nm) at different low energy
levels variably promoted the proliferation and migration of human outer root
sheath cells. Alsulaimani et al. [33] (2015) using an in vivo
rat model revealed that two weeks treatment of daily application of PBM therapy
(Ga-Al-As, at a wavelength of 830 nm) significantly increased dental root
cementum thickness in rats as determined histologically. Pourzarandian et
al. (2005) [14] reported that low-level Er: YAG laser irradiation at an energy
density of 3.37 J/cm
Within our knowledge up to now, only one study has described the effects of
laser irradiation on cementoblasts [35]. It reported that diode laser irradiation
(940 nm, 0.3 W in continuous wave, 18 J/cm
Cementogenesis, including the deposition of extracellular matrix (ECM) and its
subsequent mineralization, is an important process during cementum repair,
homeostasis maintenance and periodontium regeneration [37]. The cementum matrix
is composed of a variety of macromolecules presented in the ECM including
proteoglycan such as Lumican (Lum) and Fibromodulin(Fmod), both of which were first found in bovine cementum [38] and are
likely to play a regulatory role with respect to cementum homeostasis [38].
Fmod, a small leucine-rich proteoglycan, is involved in migration of
periodontal cells and in the regulation of collagen organization of the
pericellular and extracellular matrix in vivo [39]. Thus, it has been
proven to be one of the significant components of the ECM synthesized during
cementogenesis [39]. Lum, another small leucine-rich proteoglycan, has
been shown to promote wound healing in vitro [40]. From our present
data, the up-regulated expression of Lum along with the down-regulated
expression of Fmod observed when low-level Er: YAG when applied at 100
The expression of Fmod is reported to promote not only proliferation,
but also migration and cementogenesis [39]. Then, 1000
Previous in vitro study has shown that 100
In the current experiment using low-level Er: YAG laser irradiation, irradiated cultures, independently of pulse duration, showed higher cementogenesis induction at 7 days when compared with the control group. These results appear to be in agreement with previous in vivo studies showing higher or favorable bone tissue regeneration following periodontal Er: YAG therapy [25]. In addition, previous in vitro studies using low-level diode laser irradiation have shown laser-induced promotion of bone formation through accelerated cellular proliferation and differentiation, higher ALP activity and subsequent OCN expression in rat calvarial cells [43]. Stein et al. (2005) [44] have observed that low-level He-Ne laser irradiation induced a higher proliferation and differentiation of human osteoblasts followed by enhanced osteogenic marker expression.
Further, low-level Er: YAG laser irradiation is able to activate various cellular signaling responses in different cell types, mostly osteoblasts and fibroblasts, resulting in positive biological effects [13, 14, 15, 16, 17]. Previous studies also reported that triggered MAPKs have strong relation with cell proliferation [45, 46, 47]. Upon extracellular stimulation, by for example PBM, MAPKs serine/threonine kinases phosphorylate their corresponding specific substrates at serine and/or threonine residues [48]. Thereafter, the phosphorylation events can differently modulate substrates and through this the entire signaling cascade activity. Thus, the MAPK signaling pathways are likely to be involved in migration, proliferation and apoptosis.
The present results revealed, that the MAPK pathway on the protein level was activated in OCCM-30 cells in response to low-level Er: YAG irradiation. Phosphorylated ERK1/2 and JNK were strongly increased with long duration of expression after stimulation with higher pulse duration of low-level Er: YAG laser irradiation (Fig. 6). These results suggest that Er: YAG laser-induced cementoblasts migration, proliferation and mineralization may require ERK1/2, P38 or JNK activation, which needs to be further investigated and verified by relevant inhibitors. Moreover, the peaks of expression of the phosphorylated proteins were observed as soon as 5 min after the PBM stimuli for ERK1/2 and JNK and 10 min for P38. These findings indicate the possibility of faster cementum repair following low-level Er: YAG laser therapy, providing novel mechanistic insights into the positive effects of Er: YAG laser on cementoblasts biological response.
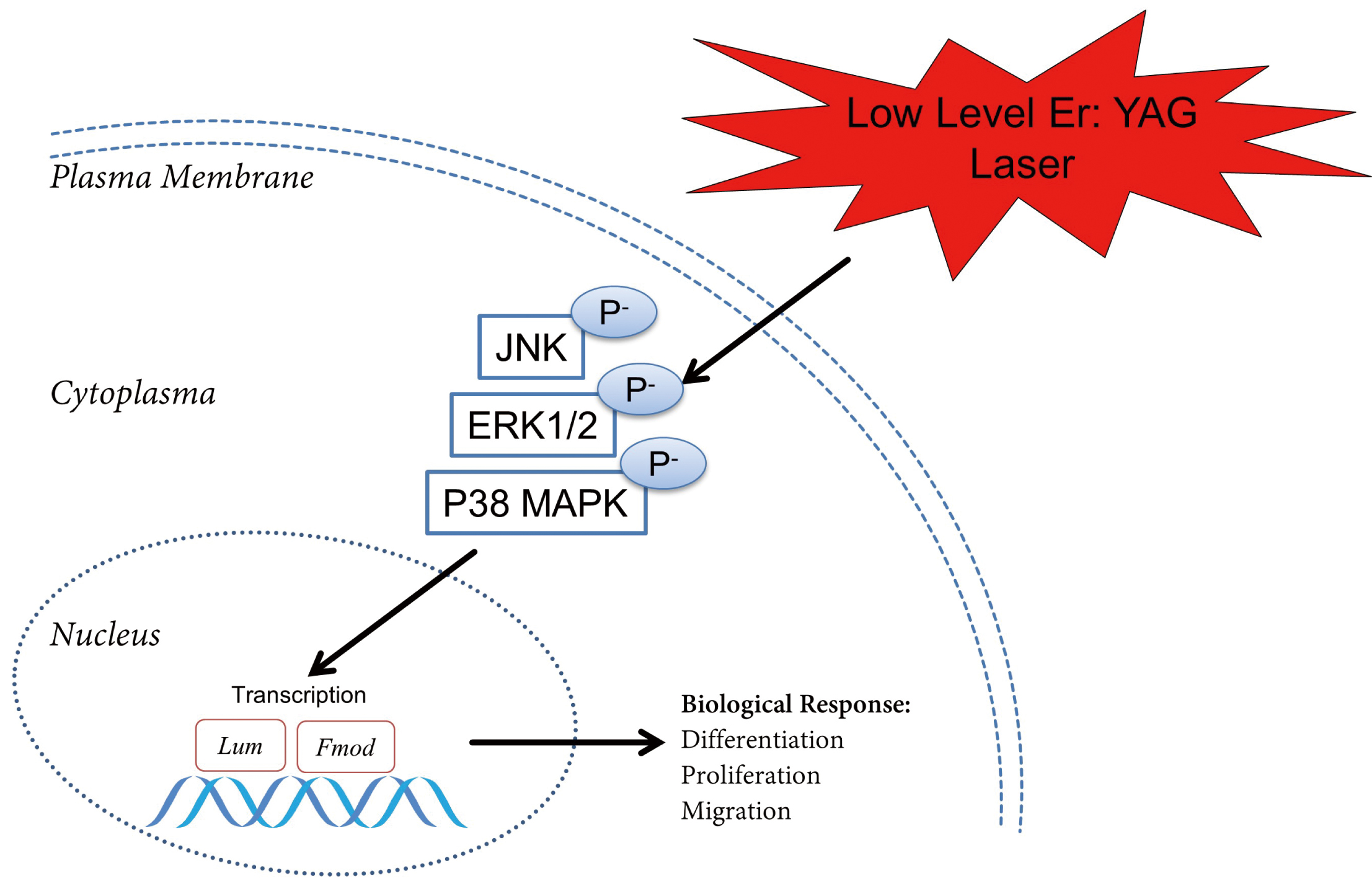
Graphic representation of the possible mode of action of the irradiation on the MAP kinase activation in cementoblasts. The proposed model describing that low-level Er: YAG laser irradiation mediates the proliferation, migration and differentiation of cementoblasts and actives the MAPK signaling pathway.
To our knowledge, until now there is no research aiming to investigate the
different pulse duration on cementoblasts. Thus, this would be the first study to
report that different effects caused by two pulse durations, which may due to the
different activation levels of MAPK signaling pathways, resulting in different
biological responses in cementoblasts. Here we have shown that the effects of 100
and 1000
Our western blot results are in line with previous research showing that PBM with several lasers activates signaling molecules [18, 49, 50]. For instance, Miyata et al. (2010) [18] reported that ERK1/2 plays a role in the increased proliferation of human dental pulp cell following low-level diode laser irradiation. Shefer et al. (2006) [49] reported that biostimulatory effect of PBM using a He-Ne laser (wavelength of 632.8 nm) induced skeletal muscle cell activation by ERK1/2. Miyata et al. (2006) [50] demonstrated that low-level diode laser (Ga-Al-As, wavelength of 810 nm) laser irradiation is able to active ERK1/2 to play an increased proliferation effect on human dental pulp cells.
The present study suggested a strong relationship between cementoblast proliferation, migration and mineralization in response to low-level Er: YAG laser irradiation with activation of ERK1/2, P38 and JNK. However, one of the limitations of the present study is the lack of a MAPK inhibition assay that could better clarify the involvement of MAPK pathways in the laser-induced biological response. Thus, further detailed investigation is required to better understand the potential involvement of these pathways in the positive effects of low-level Er: YAG laser irradiation on cementoblasts biology.
In conclusion, the results of the present study show that low-level Er: YAG laser irradiation influences OCCM-30 cells migration, proliferation and differentiation and activates the ERK1/2, P38 and JNK signaling depending on the pulse duration. Thus, it could be considered as an adjunctive therapy to be applied in some clinical situations involving dental roots, such as tooth replantation.
JY, PL—Conceptualization; Data curation; Formal analysis; Software; Writing - original draft. IKM—Data curation; Formal analysis; Investigation; Methodology. RF, SG, SR, NG, MMM—Funding acquisition; Supervision; Validation; Writing - review & editing.
Not applicable.
We sincerely thank J. Deschner and M. Nokhbehsaim (Department of Periodontology, University of Bonn, Germany) and M. Somerman, (Laboratory of Oral Connective Tissue Biology, NIH, Bethesda, MD, USA) to provide OCCM-30 cells. We also thank Stefan Gruemer (Department of Periodontology of the University of Aachen, Germany) and P. P. Li (Department of VIP center, University of Shandong, China) for their kind devices provision.
We receive financial support from China Scholarship Council (CSC). MMM is supported by CNPq (#306423/2018-9).
The authors declare no conflict of interest.
The data underlying this article will be shared on reasonable request to the corresponding author.