Academic Editor: Muntasir Billah
Background: Although controversially discussed, paclitaxel is the only
clinically proven drug that inhibits restenosis when released from drug-coated
balloons (DCBs). Limus drugs are currently being explored as alternatives. The
aim of the preclinical studies was to investigate drug candidates beyond
paclitaxel considered for balloon coating. Methods: Drugs were tested with
respect to dissolution in organic solvents, coating on balloons, and drug
transfer to the vessel wall. Inhibition of neointimal proliferation was tested in
the porcine model of coronary in-stent stenosis. Intravascular drug treatment was
achieved by DCBs at the time of stent implantation. Results: Coating had to be
adjusted for each drug. Doses on the balloons ranged from 1.0 to
8.6
Drug-coated balloons (DCBs) are one endovascular treatment option to restore patency of stenotic or occluded coronary and peripheral arteries. While the balloon mechanically reopens or widens the vessel lumen, the drug is delivered into the vessel wall to reduce neointimal proliferation, the main cause of restenosis after balloon angioplasty. Paclitaxel (PTX) was the first drug on DCBs investigated in clinical trials [1] and, to the present day, is the standard drug on DCBs. This might be due to a combination of properties of PTX including fast drug transfer to the vessel wall, strong inhibition of cell proliferation, and long persistence in the vessel wall [2]. On the other hand, the cytostatic activity of PTX and its long tissue persistence might lead to adverse reactions and have raised concerns about its safety. These concerns reinforced the initial research on other drugs [3] in the field of balloon coatings. With its long and successful history in coronary drug-eluting stents (DES), sirolimus has attracted the attention of researchers investigating new DCB technologies. Sirolimus-coated balloons have so far shown promising results in inhibition of restenosis after percutaneous coronary interventions (PCIs) in a small number of patients included in clinical studies [4, 5, 6, 7, 8]. Furthermore, a wide range of other drugs with potential antiproliferative, anti-inflammatory, or antithrombotic actions might be candidates for balloon coating. However, due to the short contact time between the balloon and the vessel wall during inflation, pharmacokinetic properties like uptake, distribution, and persistence become especially important. A suitable formulation for a balloon coating requires an adequate amount of a potent drug, proper timing of release at the target site, and uptake of an effective dose into the vessel wall [9]. The aim of the preclinical studies was to test drugs with different modes of action as coatings on angioplasty balloons for inhibition of neointimal proliferation following vessel injury in comparison to uncoated and PTX-coated balloons. Criteria for the selection of a substance were potential antiproliferative and anti-inflammatory activity, commercial availability, and approval for use in humans. Final criteria were applicability as a stable coating on a balloon membrane in a way that facilitates transfer of the drug to the vessel wall. Criteria for the evaluation of efficacy in in vivo studies were the angiographic LLL and % in-stent stenosis and the vessel wall area measured histologically in vessel cross-sections. The following compounds were studied.
Arsenic trioxide (ATO) induces apoptosis and inhibits cell growth and angiogenesis via mechanisms that are not yet fully understood. ATO is available as an intravenously injectable formulation (Trisenox) and is approved for the treatment of acute promyelocytic leukemia [10]. Arsenic trioxide-coated stents have been shown to inhibit neointimal proliferation in preclinical models of in-stent stenosis [11, 12, 13].
Glucocorticoids have an inhibitory effect on inflammatory cell activation, cytokine release, platelet adhesion, smooth muscle cell proliferation, and collagen synthesis [14, 15]. In view of these properties, it is tempting to speculate that glucocorticoids might inhibit restenosis after PCIs. Oral glucocorticoid therapy was shown to reduce the occurrence of restenosis after angioplasty [16]. Both preclinical and clinical studies investigating local intravascular administration of glucocorticoids via coated stents found beneficial effects with respect to restenosis inhibition and target lumen revascularization (TLR) [14, 17].
Bortezomib is a selective proteasome inhibitor that is approved for the treatment of multiple myeloma. It inhibits proliferation and induces apoptosis of metabolically active, rapidly dividing cells at very low concentrations [18]. Systemically and locally delivered bortezomib reduced neointimal proliferation in preclinical models of arterial injury [19, 20].
Green tea extract contains a number of catechins, including epigallocatechin-3-gallate (EGCG), epigallocatechin (EGC), epicatechin-gallate (ECG), and epicatechin (EC). EGCG was shown to have potential beneficial effects on vascular function via vasodilatory and antiplatelet activity [21, 22, 23, 24]. Preclinical studies in rats indicate that EGCG reduces intimal hyperplasia in models of intravascular injury [25, 26].
Similar to taxol, the epothilones (A and B) are microtubule-stabilizing agents
causing cell cycle arrest [27, 28]. Locally administered epothilones suppress
neointimal thickening in the rat balloon injury model [29, 30, 31]. In our
experiments we used fantolon (Bayer-Schering Pharma AG, C
Methotrexate (MTX), an analogue of the B vitamin folic acid, is a first-line synthetic disease-modifying antirheumatic drug for patients with rheumatoid arthritis (RA) and other autoimmune diseases [32]. Systemic (IV) treatment with methotrexate showed beneficial effects in a rabbit model of in-stent neoatherosclerosis [33]. Studies in RA patients at high risk for cardiovascular diseases suggest that MTX has potential protective effects. It might exert beneficial effects on endothelial function, regulating immune responses and inflammation pathways responsible for the development of atherosclerosis and thrombosis [34, 35], possibly diminishing in-stent restenosis after PCIs [36].
Thalidomide inhibits neoangiogenesis, which contributes to intravascular plaque
formation. It is a partial inhibitor of the biosynthesis of the key
proinflammatory cytokine tumor necrosis factor alpha (TNF
All studies presented here were performed between 2007 and 2022 in the same research facilities using standardized protocols as described in previously published reports [3, 42, 43].
First, we tested the solubility of the selected drugs and their stability in
various organic solvents at room temperature. Formulations containing a mixture
of the active substance, solvents and, where appropriate, an excipient (Table 1)
were coated onto percutaneous transluminal coronary angioplasty (PTCA) balloon
catheters (3.5 mm
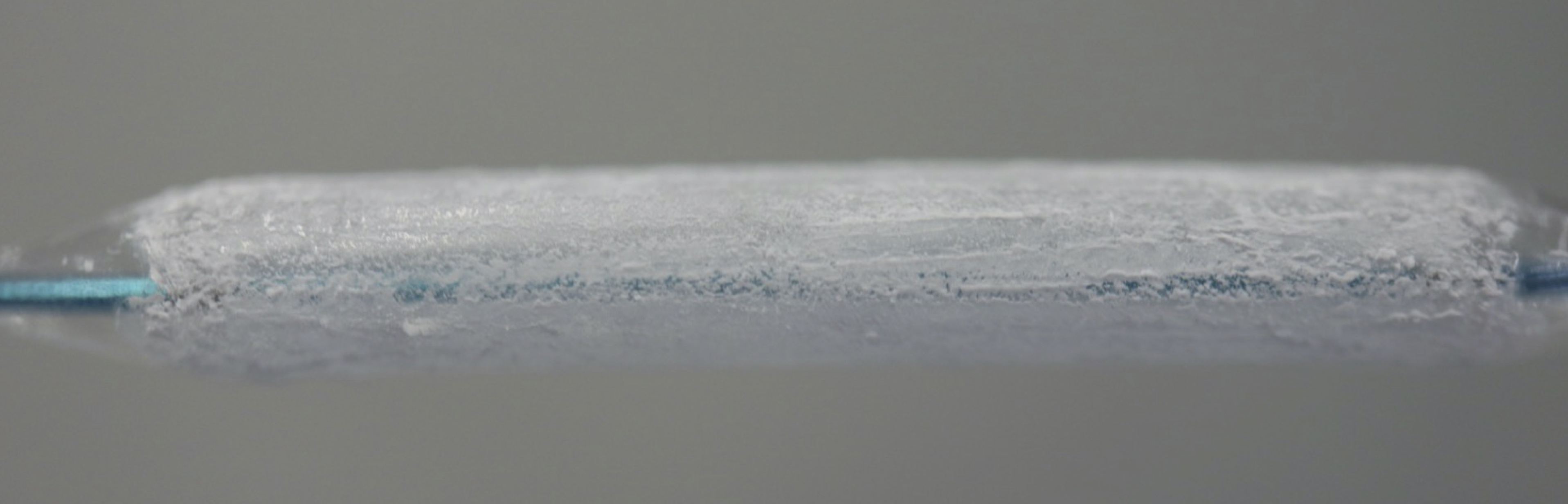
Drug coated, inflated PCTA balloon.
Drug | Solvent | Excipient | Analysis | |
arsenic trioxide | meOH | none | ICP-OES after wet incineration | |
betamethasone | meOH, THF, acetone, H |
none | HPLC/UV | |
dipropionate | ||||
bortezomib | ethanol | mannitol | HPLC/UV | |
EGCG | ethanol, acetone | UV 370 | HPLC/UV | |
fantolon | meOH | PEG 1500 | HPLC/UV or MS | |
methotrexate | meOH, aqueous bicarbonate | none | HPLC/UV | |
paclitaxel | Paccocath® | ethanol, acetone | UV 370 | HPLC/UV |
InPact® | not disclosed | urea | HPLC/UV | |
amorphous | dichloromethane | none | HPLC/UV | |
thalidomide | dioxane, DMFA | NDGA | HPLC/UV | |
meOH, methanol; THF, tetrahydrofuran; NDGA, nordihydroguaiaretic acid; DMFA, dimethylformamide; PEG, polyethylene glycol; EGCG, epigallocatechin-3-gallate; UV 370, Ultravist™, nonionic, iodinated X-ray contrast agent; ICP-OES, inductively couple plasma optical emission spectrometry; HPLC, high-pressure liquid chromatography; MS, mass spectrometry. |
All in vivo experiments were performed at the IMTR (Institute of Medical Technology and Research, Saxony-Anhalt) and conducted in accordance with the guidelines for animal experiments and approved by the animal protection committee of Saxony-Anhalt, Germany. Balloon catheters used for in vivo studies were sterilized with ethylene oxide according to a validated protocol. Studies in the porcine coronary in-stent stenosis model were conducted as described in Kelsch et al. [42]. Briefly, after sedation, anesthesia and analgesic treatment, the common carotid artery was surgically exposed and an intra-arterial sheath was introduced. The coronary arteries were visualized with a Siemens AXIOM Artis zee fluoroscope using a 6F Judkins L3.5 catheter and Ultravist®-370 as a contrast agent. Coronary angiograms were recorded after intracoronary nitroglycerin administration. After coronary imaging, a suitable segment of the left coronary artery circumflex ramus (CX), left anterior descending artery (LAD), and right coronary artery (RCA) was treated in each pig.
Drug transfer from the balloon surface to the vessel wall was examined as follows: coronary artery segments were labeled with a marker stent. Areas proximal to the implanted marker stent were treated with the coated balloons applying approximately 20% overstretch to ensure vessel wall contact. Balloons were left expanded for 1 minute, deflated, and retracted. The pigs were euthanized immediately after the last treatment in deep anesthesia using supersaturated potassium chloride. The treated vessel segments including the marker stents were dissected for drug analysis.
Neointimal proliferation was induced by implantation of balloon-expandable bare metal stents using either stents mounted onto balloons (coated or uncoated control) with a hand crimper HH100–101 (Machine Solutions, Flagstaff, AZ) or commercially available premounted bare metal stents on PTCA balloon catheters before postdilatation with experimental DCBs. Stents were expanded in coronary arteries applying approximately 20% overstretch to the vascular segment. Blood flow and lumen dimensions were monitored before and after stent implantation by coronary angiography (see below). Four weeks after the intervention, follow-up angiography of stented segments was done. Then, animals were euthanized in deep anesthesia, and the treated vessel segments were dissected and fixed with 4% buffered formalin for histomorphometric analysis.
Samples containing extraction solvent and unused balloons or balloons after use
were intensely shaken on a vortexer for at least 30 seconds, followed by
treatment in an ultrasound bath for 30 minutes and centrifugation for 10 minutes.
Except for arsenic trioxide, drug concentrations were determined by HPLC with UV
detection (Table 2). Drug analysis by HPLC was done on reversed-phase C18 columns
(5
Drug | Drug content on balloon | Residual drug on balloon after deployment in animals | Drug in the arterial wall | |
(µg/mm |
(% of dose on balloon) | (% of dose on balloon*) | ||
arsenic trioxide | 3.7 | 19.6 |
10.1 | |
betamethasone dipropionate | 6.0 | 15.9 |
12.9 | |
bortezomib | 1.0 | 53.0 |
not reported | |
EGCG | 8.6 | not reported | ||
fantolon | 2.4 | 5.1 | 5.2 | |
methotrexate | 7.6 | 2.0 |
not reported | |
paclitaxel | Paccocath® | 3.0 | 10 | 29.4 |
InPact® | 3.5 | 13 | 19.7 | |
amorphous | 3.3 | 30.2 |
7.4 | |
thalidomide | 5.9 | 6.8 |
29.0 | |
* calculated as % of (initial) dose on the balloon measured in the excised vessel segments. |
Quantitative coronary angiography (QCA) was done before, during, and after
balloon inflation and stent implantation. Four weeks after stent implantation,
follow-up QCA was performed at the respective implant sites to assess the primary
endpoint late lumen loss (LLL, defined as minimal lumen diameter at implantation
(MLD) – minimal lumen diameter at 4-weeks follow-up (MLD FU)) and in-stent
stenosis % (defined as (1 – MLD FU / reference diameter (RFD))
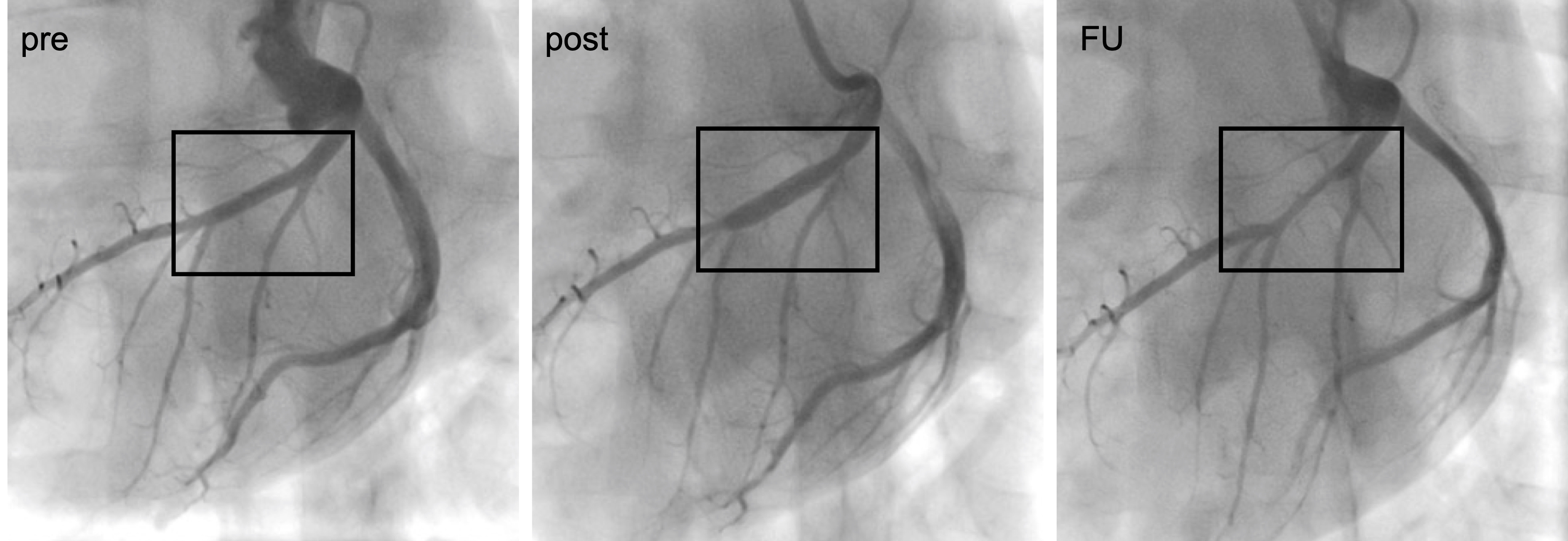
Angiograms showing a coronary vessel pre- and post treatment with a DCB and at 4-weeks follow-up (FU).
The target segments of treated coronary arteries were embedded in methyl-methacrylate, cut with a coping saw, polished, and glued on acrylic plastic slides. Cross-sections from proximal, mid and distal portions of the stent were stained with hematoxylin and eosin (H&E) and/or Masson-Goldner and digitalized. From these images, histomorphometric measurements of lumen and vessel wall area (defined as vessel area – lumen area) were recorded. Injury scores were assigned as described by Schwartz et al. [44], and inflammation was graded individually for each stent strut using the score introduced by Kornowski et al. [45]. The injury score and the inflammatory score for each cross-section were calculated as the sum of the individual injury and inflammatory scores divided by the number of struts in the examined section.
QCA-derived parameters were compared across treatment groups. Histomorphometric
variables of the three cross-sectional planes were averaged to obtain a mean
value per stent. Continuous variables were compared by Kruskal-Wallis one-way
ANOVA test using Graphpad Prism (Version 6, GraphPad Software, San Diego, CA, USA). Data are presented as mean
Compounds were completely dissolved in the aforementioned mixtures and coated on
plain 3.5 mm
On the basis of the in vitro and in vivo studies, the following drugs were selected to be tested with respect to their efficacy in reducing in-stent stenosis in the porcine coronary overstretch and stent implantation model: arsenic trioxide, betamethasone dipropionate, bortezomib, epigallocatechin-gallate, fantolon, methotrexate, and thalidomide. Both uncoated and PTX-coated balloons, for which inhibition of restenosis is known from controlled clinical trials [47, 48], served as controls. Outcome of balloon treatment in the porcine in-stent stenosis was assessed from in vivo coronary angiography and ex vivo histological analysis. First, pooled QCA data at 4-week follow-up for uncoated balloons from multiple independent studies were compared with data for the three different PTX coating formulations, namely Paccocath, InPact, and experimental amorphous PTX (see Table 2). All three PTX formulation variants significantly reduced angiographic in-stent LLL compared to the pooled uncoated balloon group (Fig. 3). LLL reduction was not significantly different among the three PTX variants.
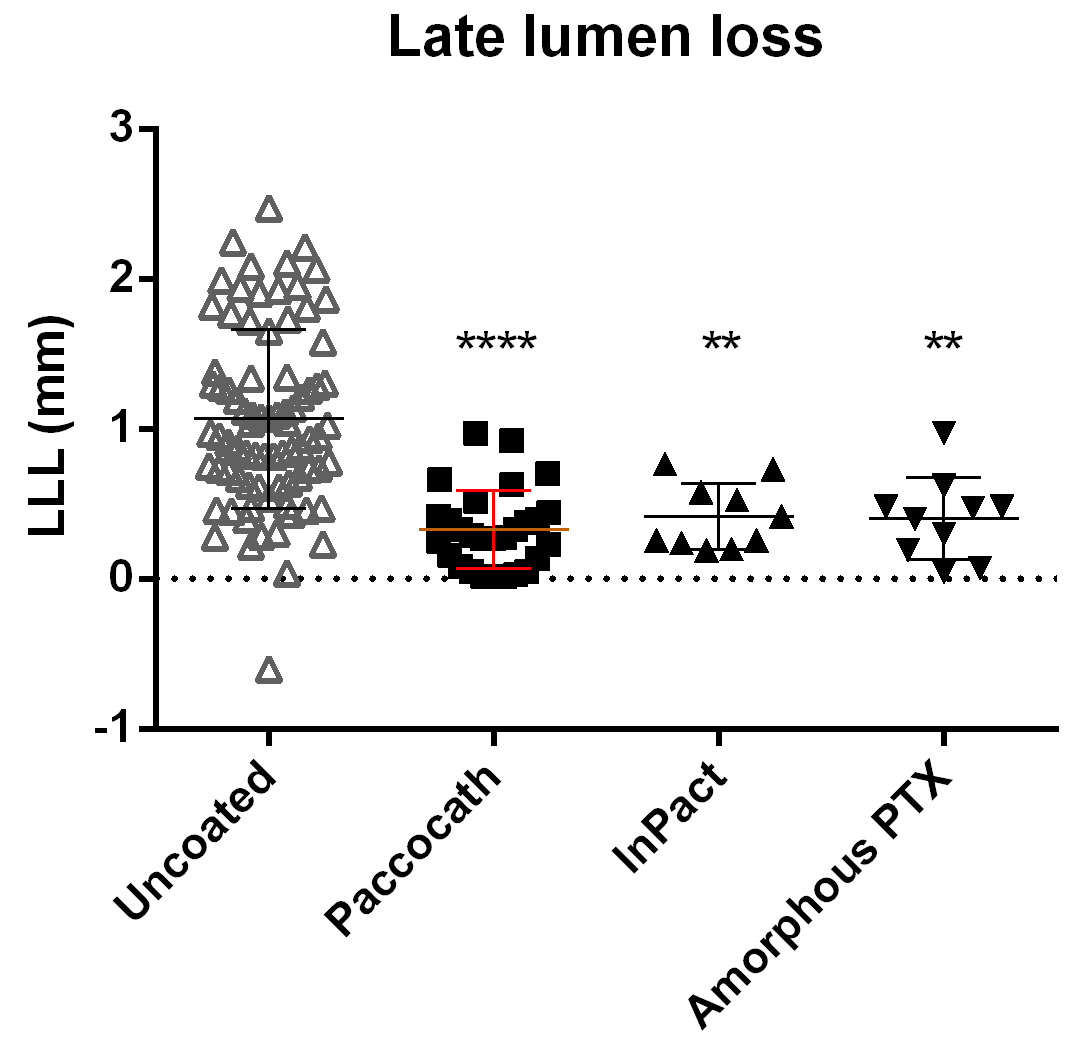
QCA in-stent late lumen loss (LLL) at 4-week follow-up. Balloons
coated with different PTX formulations significantly reduce LLL compared to
uncoated balloons. Data are shown as mean
To elucidate a potential inhibition of neointimal proliferation of the different novel balloon coatings, we compared data points from multiple experiments investigating the drug candidates with the pooled results achieved with uncoated control balloons and PTX formulation variants. For all studies, quantitative coronary angiography revealed similar angiographic baseline parameters in each treatment group within each study versus the uncoated control. Except for treatment with PTX-coated balloons, angiographic parameters at 4 weeks after implantation did not show a significant reduction of in-stent stenosis in any of the tested drug-coated balloon groups compared to uncoated controls (Table 3, Fig. 4). Unlike treatment with PTX-coated balloons, none of the new coatings we investigated inhibited neointimal proliferation, i.e., QCA showed no decrease in LLL or in-stent stenosis or increase in minimal lumen diameter compared to treatment with uncoated balloons.
Treatment group | QCA baseline | QCA at 4-week FU | |||
RFD (mm, n) | MLD in-stent (mm, n) | MLD in-stent (mm, n) | LLL in-stent (mm, n) | Stenosis in-stent (%, n) | |
arsenic trioxide | 2.51 |
2.78 |
1.91 |
0.87 |
26.8 |
BDP | 2.32 |
2.57 |
1.57 |
1.00 |
33.8 |
bortezomib | 2.56 |
3.01 |
1.27 |
1.74 |
54.1 |
EGCG | 2.39 |
2.38 |
1.13 |
1.24 |
51.8 |
fantolone | 2.81 |
2.81 |
2.25 |
0.86 |
20.2 |
methotrexate | 2.78 |
3.01 |
1.86 |
1.09 |
32.1 |
thalidomide | 2.18 |
2.67 |
1.08 |
1.59 |
53.5 |
uncoated | 2.41 |
2.75 |
1.71 |
1.07 |
34.4 |
PTX | 2.71 |
2.95 |
2.61 |
0.36 |
6.21 |
n, number of treated arteries; RFD, reference diameter = mean lumen diameter
before treatment; MLD, minimal lumen diameter; LLL, late lumen loss = MLD –
MLD FU; Stenosis%, (1 – MLD FU / RFD) |
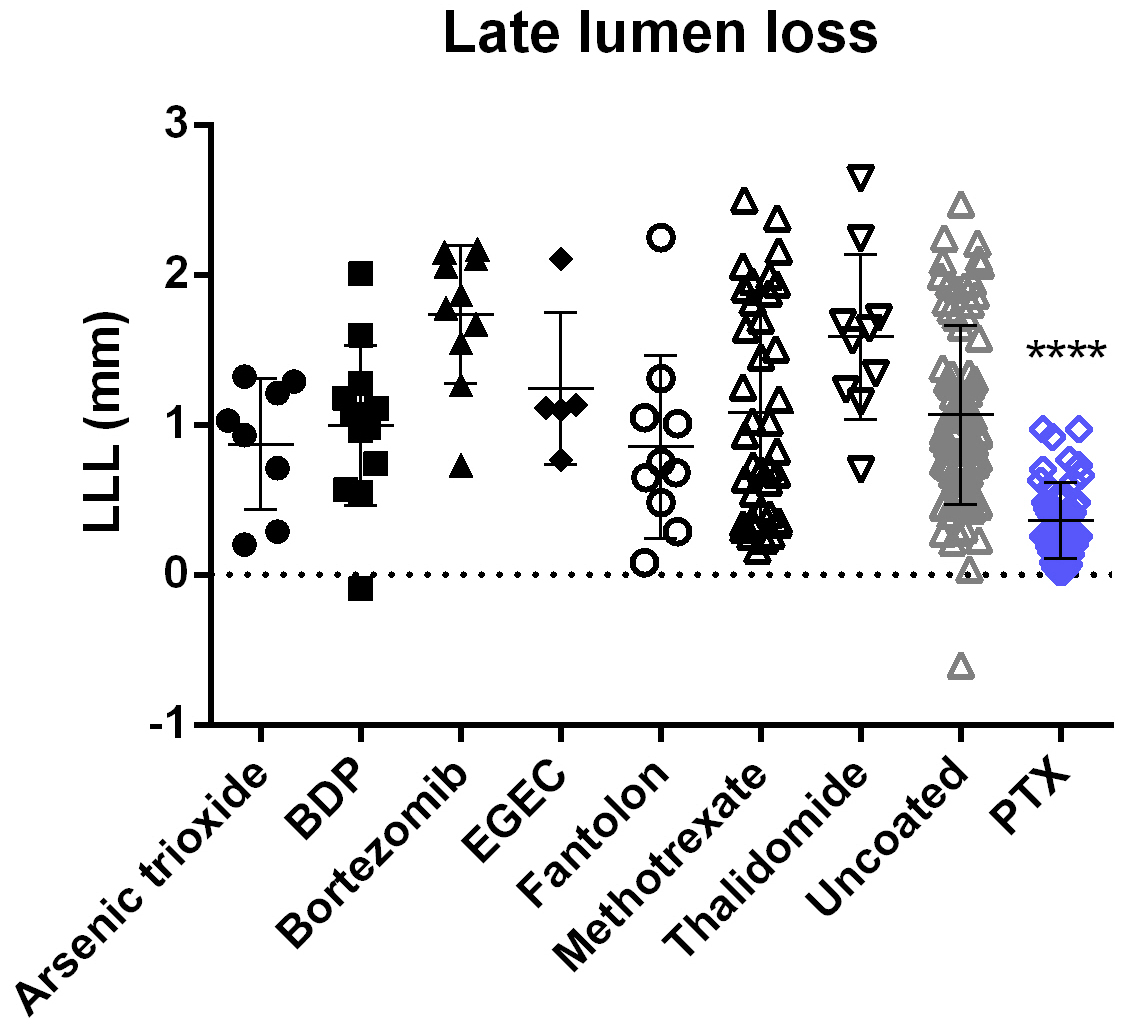
QCA in-stent late lumen loss (LLL) at 4-week follow-up.
Following treatment with PTX-coated balloons, LLL is significantly lower compared
with the pooled results achieved with uncoated balloons. For all other coatings
tested, results are not significantly different from those of the uncoated
control group. Data are shown as mean
Histological analysis of stented cross-sections showed no reduction of neointimal area for any balloon coatings except PTX compared to the uncoated control group (Table 4, Fig. 5). These results confirm the ineffectiveness of the tested balloon coatings with respect to inhibition of neointimal proliferation.
Treatment group | Lumen area (mm |
Vessel wall area (mm |
Injury score (n) | Inflammation score (n) |
arsenic trioxide | 4.27 |
4.09 |
2.53 |
2.64 |
BDP | 3.52 |
4.70 |
1.67 |
2.03 |
bortezomib | 4.12 |
5.76 |
1.75 |
2.05 |
EGCG | 2.04 |
5.39 |
2.14 (1) | 3.00 (1) |
fantolon | not done | not done | not done | not done |
methotrexate | 3.66 |
4.40 |
1.27 |
1.56 |
thalidomide | 4.34 |
4.67 |
not done | not done |
uncoated | 4.12 |
5.03 |
1.94 |
2.04 |
PTX | 5.86 |
2.92 |
1.75 |
2.30 |
n, number of treated arteries available for evaluation; BDP, betamethasone dipropionate; EGCG, epigallocatechin-3-gallate; PTX, paclitaxel formulation variants. |
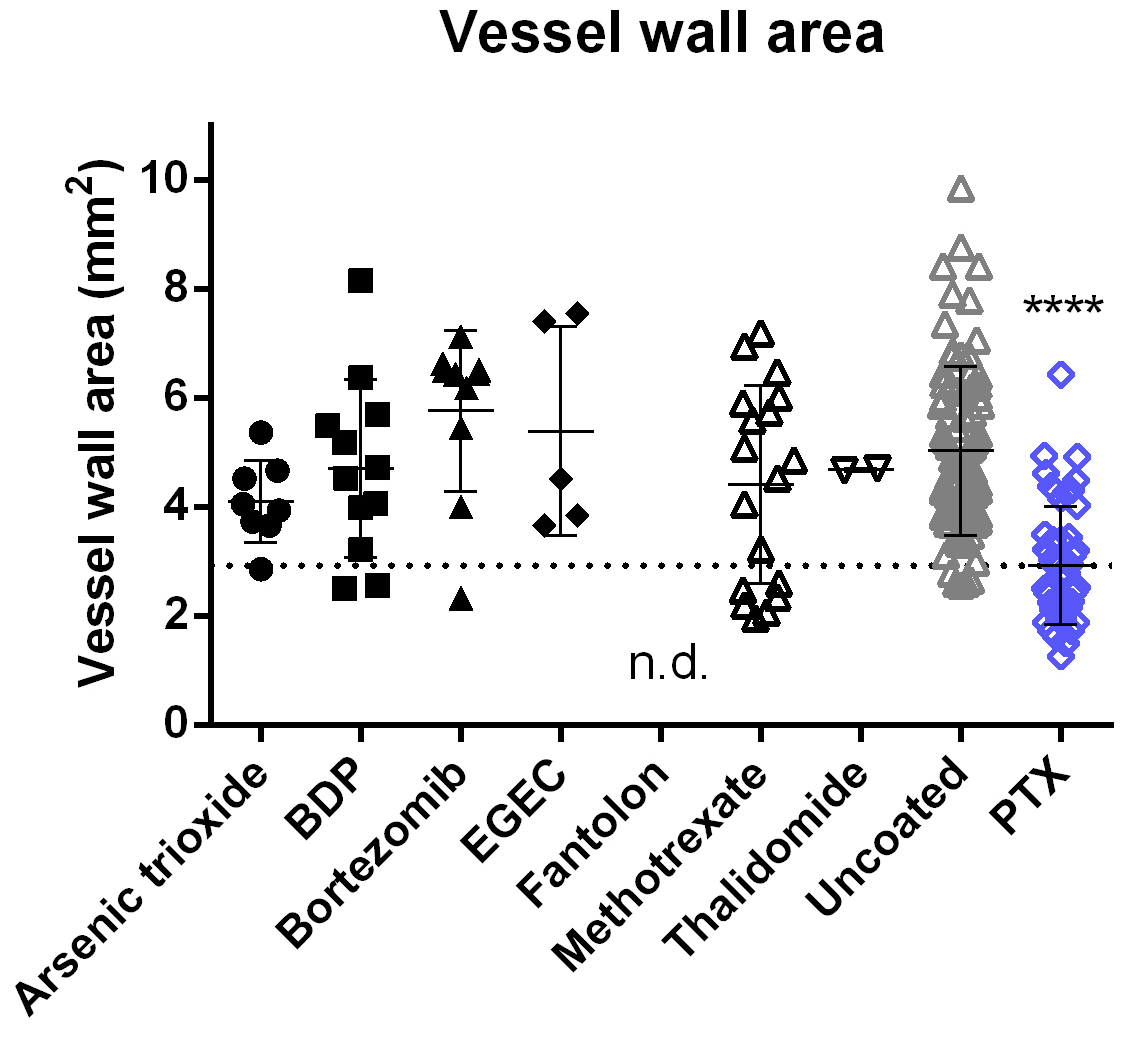
Histomorphometric in-stent vessel wall area at 4-week follow-up.
Following treatment with PTX-coated balloons, the vessel wall area (including
neointima) is significantly smaller than after treatment with uncoated balloons.
For all other coatings tested, results are not significantly different from those
of the uncoated control group. Data are shown as mean
Cross-sections of stented artery segments showed significant neointimal thickening and lumen narrowing for all balloon coatings investigated except for the PTX group. Nearly all sections from arteries treated with PTX-coated balloons exhibited only a thin cellular layer covering the stent struts (Fig. 6).

Representative H&E-stained histological cross-sections of stented artery segments at 4-week follow-up. BDP, betamethasone dipropionate.
None of the tested devices, coatings, or applications caused any signs of obvious toxicity during the experiments. None of the drugs had to be excluded from further testing because of recognizable local or systemic toxicity.
Despite the antiproliferative and/or anti-inflammatory effects of the drugs in
the coatings investigated here (for details please see introduction), the results
of our experiments were disappointing. The coating formulations were adjusted to
accomplish a transfer similar to that published for paclitaxel (i.e.,
While LLL following treatment with PTX-coated balloons was consistently low in
our studies (with means of individual studies ranging from 0.22 to 0.42 mm LLL;
see Table 3; Figs. 3,4), all investigated non-paclitaxel formulations showed no
effect in terms of inhibition of neointima formation compared to uncoated
balloons despite acute transfer of a respectable percentage of the dose into the
vessel wall (e.g., thalidomide coating: about 30% of dose recovered in the
arterial wall and LLL of 1.59
In our experiments, dichloromethane formulation yielded an amorphus paclitaxel coating that showed an inhibition in in-stent stenosis similar to other paclitaxel coatings. This is in contrast to the amorphus paclitaxel-iopromide coating of Granada et al. [50], which seemed to be ineffective in the porcine in-stent stenosis model. Even for PTX-coated balloons, it has long been known that there is no class effect and that inhibition of neointimal proliferation depends critically on the coating composition and method rather than the active drug alone [46, 51].
Although paclitaxel coated balloons were developed in the porcine in-stent stenosis model, it may not be the appropriate model for testing these novel drugs. In porcine studies, a significantly stronger reduction of neointima formation by paclitaxel compared with sirolimus was seen [43]. These findings are in contrast to coronary clinical data where Limus stents exhibit equivalent restenosis inhibition and dominate the market. The differences may be related to the fact that the animal model (juvenile animals, no atherosclerosis, and artificial overstretch) does not fully reflect the situation in humans. Furthermore, different biological responses may be postulated for pigs and humans [3].
Our current study shows that the findings for paclitaxel coatings do not seem to be easily transferable to other agents and give an insight into the difficulties of developing new drug coatings.
Also, in response to frequent criticism of PTX, we systematically investigated several drugs to explore their potential use as balloon coatings for inhibiting neointimal proliferation in the animal model which led to the discovery of clinically proven DCBs. Despite potent antiproliferative effects of some drugs and successful drug transfer into the vessel wall, their inhibitory effect on neointimal formation in porcine coronary arteries was far below that of PTX-coatings in our experiments. Reasons for the lacking efficacy may be the solvent compositions and/or additive used, the balloon surface, and the animal model. Better outcomes may be achieved with other coating formulations and/or different balloons.
BDP, betamethasone dipropionate; EGCG, Epigallocatechin-3-gallate; LLL, late lumen loss; MLD, minimal lumen diameter; PTX, paclitaxel; RFD, reference diameter; QCA, quantitative coronary angiography.
TH, US, BSchn designed the research study. TH, SBie, ML, NB, OG, DS, SBet, BK, BSche, BSchn performed the research and analyzed the data. TH, US, BSchn wrote the manuscript. All authors contributed to editorial changes in the manuscript. All authors read and approved the final manuscript.
The animal experiments were approved by the local animal ethics committee (Landesverwaltungsamt LVwA Saxony-Anhalt, Germany) with the approval number (42502-2-1508) and were conducted in accordance with European commission directive 86/609/EEC and the German Animal Protection Act.
The authors would particularly like to thank the IMTR for conducting the in vivo studies.
This research received no external funding.
US and BSche are shareholder of InnoRa GmbH. TH, SBie, ML, NB, DS, BK are employees of InnoRa.
Publisher’s Note: IMR Press stays neutral with regard to jurisdictional claims in published maps and institutional affiliations.