Cardiovascular diseases including myocardial infarctions, myocarditis, strokes, coronary artery disease, chronic granulomatous disease, atherosclerotic cardiovascular disease, etc. can be regarded as the severe health trouble round the globe. The reasons behind the heart related complications have been well chalked our so far. Interestingly, along with the non-infectious reasons, an array of bacteria, fungi, parasites and viruses is known to cause different types of heart complications. Unfortunately, the role of microorganisms in inducing heart diseases is not that much known by the mass community in the underdeveloped and even in the developing countries over the world. However, among the microorganisms causing heart diseases, the multifaceted bionetwork by the gut microorganisms especially drew the interests of microbiologists. The impairment of cardiac membrane, the metabolic malfunction of heart, and imbalance in the functionality of the immune cells by the alternation in the composition of gut microorganisms are currently not unknown. Present review outlined the onset of heart diseases caused by the gut microflora in a simple way which would be important in public health regard.
Cardiovascular disease (CVD) is one of the leading causes of mortality and morbidity worldwide, claiming approximately 17.7 million life per year [1]. Despite making major advancement in the cardiovascular treatment arena, cardiovascular diseases such as heart failure, stroke, myocardial infarction, my ocarditis are still on the rise. Myocardial infarctions, strokes, peripheral vascular disease, heart failure and other CVD’s possess a massive burden on public health.
The presumed cost of alterations in the gut microbiota and the progression of CVD and the cardiometabolic diseases is well known. Though the effects of gut microbiota metabolism of a protein-rich diet have been well studied, however the association of these microorganisms with cardiovascular risk is debatable. The complex ecology of the gut microbiota and its biochemical impacts or the metabolic functionality is a topic of interest for the clinicians, cardiovascular researchers, and food professionals. The present review attempts to demonstrate the link between gut microorganisms and CVD. In addition, we also proposed couple of mechanistic models to explain the gut microorganism’s mode of action in CVD pathology.
Both infectious and non-infectious agents have been implicated to CVD [2]. Blood culture-negative endocarditis (BCNE), accounting for almost 70% of the endocarditis cases [3], has been reported to be diagnosed in the patients previously treated with antibiotics [4]. In addition, presence of slow-growing or intracellular microorganisms like Bartonella quintana, Staphylococcus aureus, Streptococcus equi, and Streptococcus oralis may also cause BCNE [4, 5]. Helicobacter pylori, Mycoplasma pneumonia, Bacillus typhosus and Chlamydia pneumonia, and viral pathogens including fowl adenovirus serotype 4 (FAdV-4), parvovirus B19, Hepatitis C Virus (HCV), Enterovirus (Coxsackie Virus), thecytomegalovirus and herpes simplex viruses have long been known to cause coronary artery disease, myocarditis and pericarditis [6, 7, 8, 9]. Myocarditis caused by Staphylococcus aureus often results into sepsis (bacteria growing in the blood) and may form abscesses in the heart [6]. Among the blood born parasites Borrelia burgdorferi, Ehrlichia and Babesia spp. have been found to be associated with myocarditis [10]. Fungal populations are also known to cause heart diseases. Among them, Aspergillus fumigatus, A. flavus and A. nidulans have been frequently reported [11].
Enteric bacteria Staphylococcus aureus, and Mycobacterium as well as fungal pathogens are well documented to cause CVD [12]. Genetic mutations may decrease the intracellular superoxide production by the immune cells like granulocytes (neutrophils and eosinophils). Such response by the granulocytes have been found to trigger the chronic granulomatous disease (CGD), an inherited primary immune deficiency disorder resulting in life-threatening recurrent fungal and bacterial infections of the skin, lungs and bones with associated chronic inflammation or granulomas (Fig. 1). Patients with CGD have been reported to suffer from continual bacterial and fungal infections throughout their life. In CGD, granulocytes, monocytes and macrophages reduce phagocytosis due to the lack of synchronization in the production of reactive oxygen species (ROS) which is required to eliminate the pathogenic microorganisms [11, 13, 14]. Therefore, this is suggestive that CVD inducing microorganisms mainly manifest the opportunistic mode; and CVD is unfortunately caused by the recurrent and/or invasive infections, especially by the catalase-positive bacteria and fungi [11, 15].
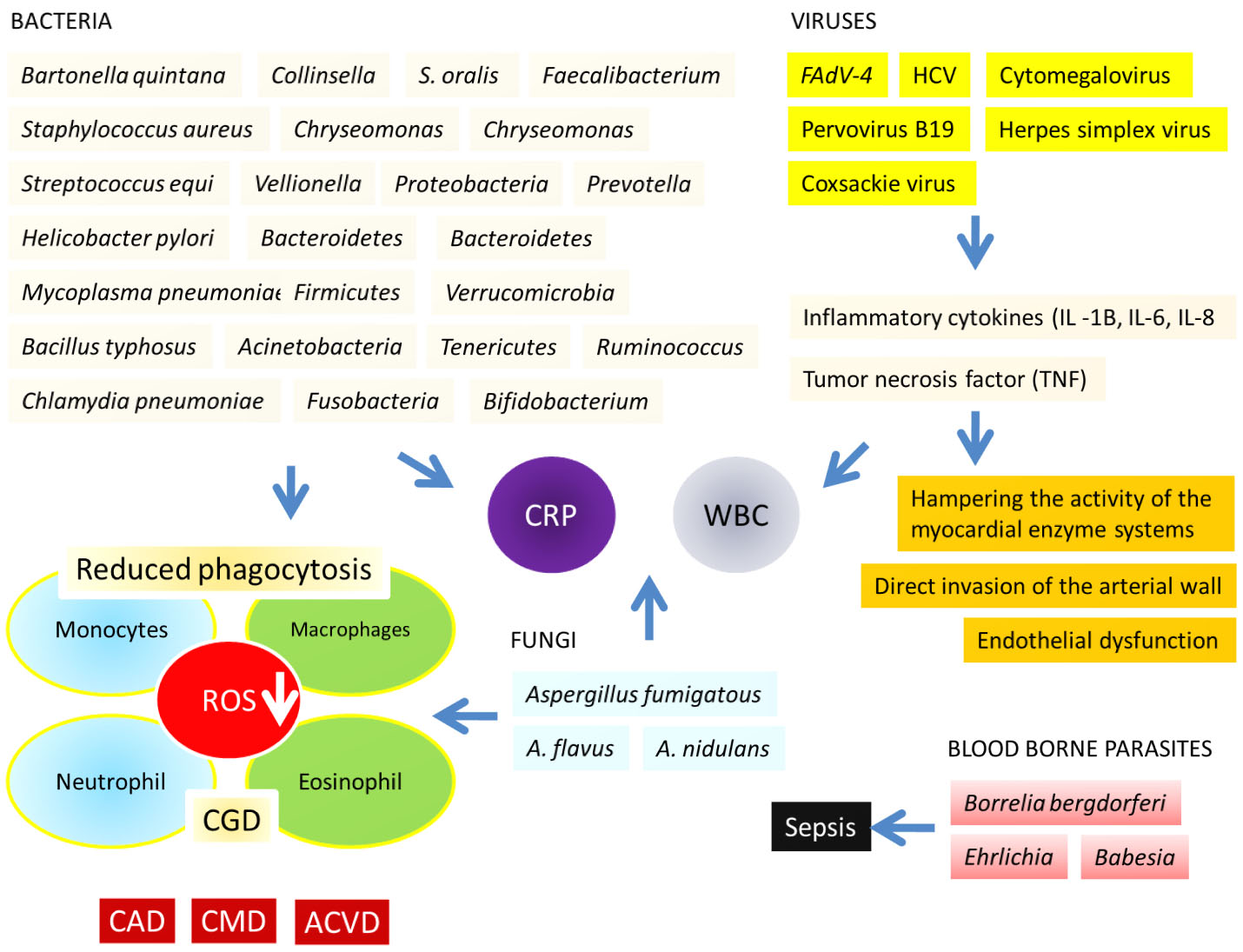
Cardiovascular diseases (CVD) in the forms of chronic granulomatous disease (CGD), coronary artery disease (CAD), cardiometabolic diseases (CMDs), atherosclerotic cardiovascular disease (ACVD) triggered by a vast population of bacteria, fungi, viruses especially the adenovirus serotype 4 (FAdV-4) and Hepatitis C Virus (HCV); by the blood borne parasites. Roles of the inflammatory cytokines; i.e., interleukin (IL) 1B, IL-6, IL-8, and the tumor necrosis factor (TNF) have been shown in this figure. Details are described in the text.
Extensive studies on FAdV-4 have paved the way of understanding the mechanism of virus-induced CVD. It is suggested that, viral infection compromises cardiac integrity and function through the inflammatory cytokines such as interleukin (IL) 1B, IL-6, IL-8, and the tumor necrosis factor (TNF) [16]. In addition, it also hampers the activity of the myocardial enzyme cascade [17]. Moreover, HCV virus may cause myocardial complications through endothelial dysfunction and direct invasion of the arterial wall. Another important pathology of the heart disease is the autoimmune (non-viral) myocarditis, whereby autoantibodies may influence the disease progression [2, 18].
The gut microbiota is a complex ecosystem of symbiotic bacteria. It contributes to the mammalian host physiology and metabolism by (i) aiding the processing of the apparently indigestible nutrients, (ii) supplying the necessary metabolites, and (iii) activating the host’s protective immune system to some extent [19, 20]. Macrophages, neutrophils, monocytes, innate lymphoid cells, and bacterial metabolism products like short-chain fatty acids (SCFAs) are known to inhibit the growth of pathogens by producing primary and secondary bile acids, and butyrate [21]. Bacteria and viruses directly produce microbe-associated molecular patterns (MAMPs) on the epithelial cells or on the dendrites [21]. It is followed by the production of interleukin (IL) -22, which in turn promotes the secretion of the antimicrobial peptides (AMPs) and elicit the host innate immunity. Eventually, B cells produce IgA and IgG antibodies that target the pathogenic bacteria in the lumen.
The gut microorganisms are well known for imparting the protective immunity for the host cells. In contrast, the changes in the gut microbial composition (principally causing the accumulation of the pro-inflammatory molecules) surprisingly plays a substantial physiological role between the host and nutrition. Gut microorganisms also synthesize several metabolites that impair the host metabolic homeostasis and the protective immunological processes, and trigger cardiometabolic diseases (CMD) [20, 22]. CMD has been reported to be caused by the gut microbiome [23, 24].
An array of the gut microbiota including Collinsella, Chryseomonas, Veillonella and Streptococcus has been reported in the coronary artery disease (CAD) patients [25]. Microorganisms like Bacteroidetes, Firmicutes, Actinobacteria, Proteobacteria, and Verrucomicrobia, Fusobacteria, Tenericutes, Spirochaetes, Cyanobacteria, Bifidobacterium, Faecalibacterium, Ruminococcus and Prevotella may also be responsible for the CVD [26, 27]. CAD is linked with gut microbiota alterations in different populations, and gut microbe-derived metabolites may serve as markers for major CVD. A metagenomic study of stool samples from 218 individuals with atherosclerosis (ACVD) and 187 healthy volunteers were compared. Samples from atherosclerotic individuals gut microbiome deviated from the healthy status and was found to contain increased quantity of Enterobacteriaceae and Streptococcus spp. [23].
Besides bacteria, fungal population such as the most common fungal genus Candida, genera of Saccharomyces, Mucor, Fusarium, Malassezia, Penicillium, Aspergillus, Cryptococcus, Malassezia and Cladosporium are also prevalent in the resident gut microbiome [26, 28, 29, 30]. The gastrointestinal (GI) is also dominated by a very small proportion of Archaea like Methanobrevibacter, Methanosphaera, Nitrososphaera, Thermogynomonas, Thermoplasma, Methanomethylophilus alvus [31, 32]. Studies on gut viral population is limited; however, a metagenomic study unraveled the action of a bacteriophage, cross-assembly phage (crAssphage), which is associated with Bacteroides [33].
The gut microbiome functions like an endocrine organ, generating the bioactive metabolites that can impact host physiology. Changes in the composition of gut microbiome linked with disease, commonly referred to as dysbiosis, have been reported to be associated with atherosclerosis, hypertension, heart failure, chronic kidney disease, obesity and type 2 diabetes [34]. Alteration/changes within the gut microflora may pose significant impact on the regulation of host biochemical and metabolic design. The knowledge on (i) gut microflora, (ii) associations between the microbiome and the inflammasome, (iii) the innate immune system, (iv) role of bile acids, (v) the gut permeability may aid potential preventive strategy against CVD and coupling microorganisms to the pathogenesis of the autoimmune disease [19, 35, 36, 37, 38, 39].
Indeed, the gut microorganisms are one of the important main factors influencing systemic immune responses and metabolic dysfunctions among the individuals with obesity. It plays a significant role on the onset of the atherosclerosis [40]. Based on the capacity of converting common nutrients to metabolites, the resident microflora within the intestinal tract may act as filters of the diet. The specific microbial-associated metabolites like trimethylamine-N-oxide (TMAO), short-chain fatty acids (SCFAs) and secondary bile acids have been reported to influence CVD development [38]. While the lower gut microbial diversity may be linked with the elevated level of white blood cells and the C-reactive protein (hsCRP) levels; the high loads of gut microorganisms are inversely interrelated to different markers of low-grade inflammation including hsCRP and interleukin (IL) -6 [22, 40].
The impact of gut bacteria on triggering the CVD has been rigorously studied using the advanced technologies till date [22, 41, 42]. One study showed that the products from a protein-rich diet like L-carnitine and phosphatidylcholine was converted to trimethylamine (TMA) by the gut bacteria Escherichia coli, which was further oxidized into TMAO in the liver promoting atherosclerosis and cardiovascular diseases [41]. Elevated level of gut bacterium Collinsella was reported in the carotid artery of symptomatic atherosclerosis patients. However, Lactobacillus rhamnosus GR-1, Bifidobacterium infantis, and Methanomassiliicoccus luminyensis B10 as probiotics were noticed to be effective in treating heart failure in mouse model. It further supports the central role of gut microflora in inducing the CVD [43]. Periodontal pathogens (causing periodontal disease, PD, an inflammatory disease of the oral cavity) such as Porphyromonas gingivalis, Prevotella intermedia, and Fusobacterium nucleatum heightened high-fat diet (HFD) -induced systolic and diastolic arterial pressure in diabetic mice. The lipopolysaccharide (LPS) from P. gingivalis was suggested to be responsible for the inflammation-induced CVD through the elevated oxidative stress (increased ROS) and mitochondrial dysfunction [42]. Another interesting study using mouse model showed the mechanisms by which the microorganisms may control the pathogenic inflammation in the heart and the novel innate immune responses [19]. A generalized model of heart diseases induced by the gut bacteria has been clarified in Fig. 2.
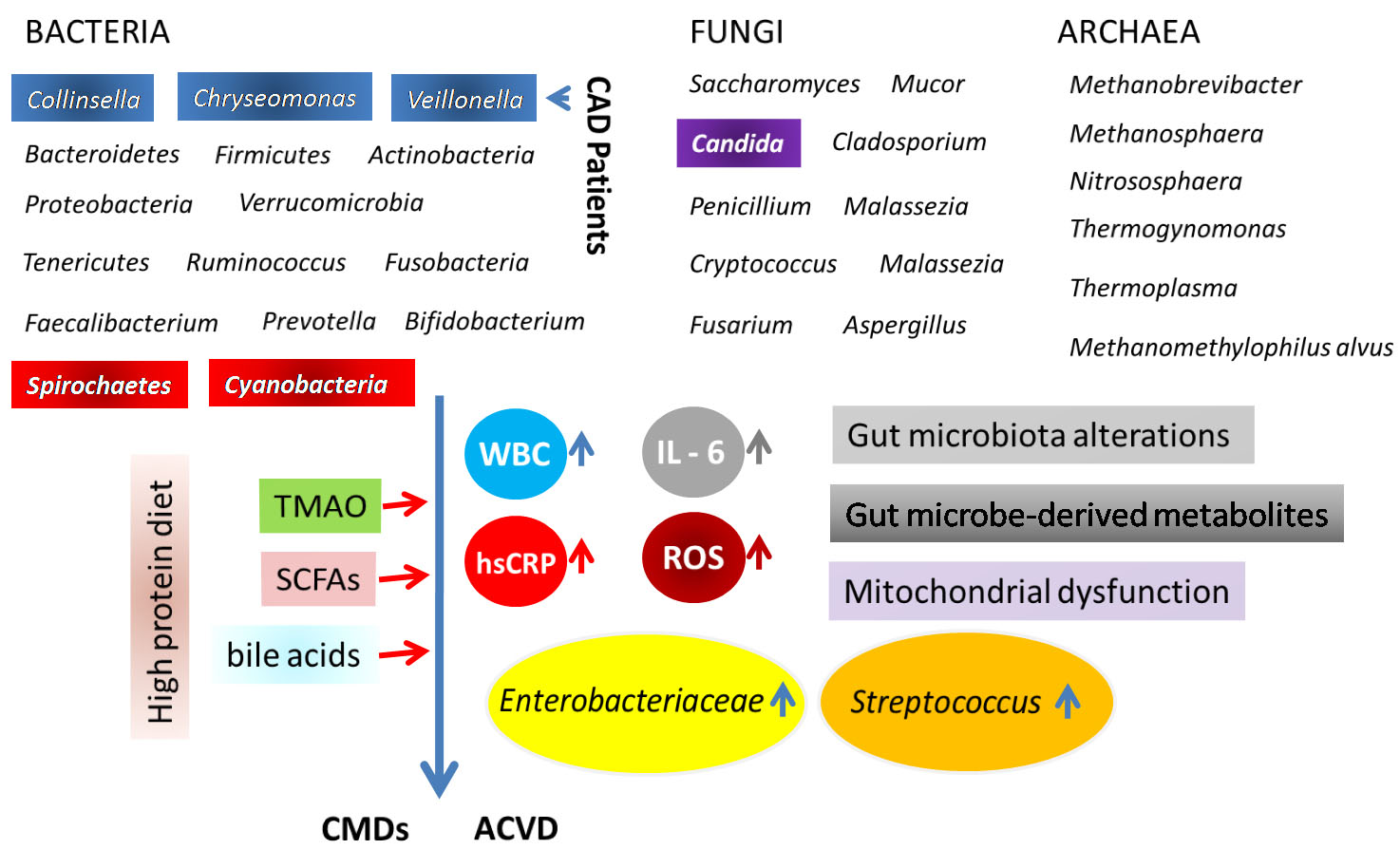
Generalized model of heart diseases induced by the gut bacteria. Details are given in the text.
The gut bacteria possess several receptors like Lipopolysaccharide (LPS) and
pattern recognition receptors (PRR) that stimulate and instruct host immune
response [44]. A number of pathways including trimethylamine (TMA)/trimethylamine
N-oxide (TMAO) pathway, SCFAs pathway, and primary and secondary bile
acid (BAs) pathways are well known interact with the host endocrine hormones
including ghrelin, leptin, and glucagon-like peptide 1 (GLP-1) [45]. In
perspective of linking adaptive immunity to the CVD, the suppression of immune
and inflammatory responses by the glucocorticoid-induced leucine zipper (GILZ)
protein has been reported to induce Myocardial infarction (MI) along with a
reduced level of Th-17 cells but an elevated level of the anti-inflammatory
cytokine IL-10 positive cells [46]. Cytokines, including interferon-
From the evidences of a range of clinical research as well as through a vast biotechnological application, a large number of microorganisms have been identified to provide both beneficial and harmful impacts to human health. While the advantageous effects are generated from the symbiotic relationship between the normal flora (microorganisms residing within the host as the first line of immune defense) and the host, the destruction of host immunity or the metabolic or genetic alternations among the microbiome may turn those into the opportunistic pathogens, causing infections including the systemic rheumatic diseases [48]. Interestingly, another recent study has shown that alteration in gut microbiome can trigger the CVD and autoimmune disease [49]. An important gut bacterium, Roseburia intestinalis, has been reported to trigger inflammation in patients with the antiphospholipid syndrome (APS) [50]. Surprisingly, their gut immune cells produce antibodies to kill their own cells [50, 51]. Probiotic treatment with Lactobacilli reversed the condition by restoring the composition of the gut microbiome [52]. Certain advanced microbiological methods and molecular biological techniques have been found to be very useful in the detection of microorganisms associated with heart diseases. Advanced molecular methods like the polymerase chain reaction, 16S rRNA sequencing, and next-generation sequencing have been shown to be effective for the direct detection of microbial pathogens in CVD including infective endocarditis [53, 54]. Such molecular tools are an excellent alternative to the culture-based methods, particularly in detecting the viable but non-culturable pathogenic microorganisms [53].
In our opinion, the proposed models presented in the current review would highlight the mechanism of bacteria-induced CVD. An idea on the alterations in the gut microbiota along with their metabolic strategies would be of interest both for the biochemists and the food microbiologists. Thus, the objectives of this very review are expected to meet the current knowledge demand for the linkage between the complex microbial ecology and the cardiovascular diseases. Further deciphering the molecular mechanisms of microbial attach of heart would be of great interest. Another important point to ponder is that the cardiovascular disease is progressively more imperative basis of clinical fatality in Bangladesh. The cases of coronary artery disease, hypertension, etc. are of current epidemic importance. Different environmental and dietary risk factors are commonly analyzed for heart diseases; however, the involvement of microorganisms triggering heart related complications are ignored. Therefore, not only in the general global aspect, rather, for the welfare of the mass public health, the knowledge from the current review would be useful in local perspective.
RN conceived and designed the research project and interpreted the data. RN, AN, SMM and MB contributed writing and editing the manuscript. AN, SMM, MB, NT, TasT, TahT and MAT conducted the literature survey and gathered the required information to write the review. MB, NT, TasT, TahT and MAT also helped in formatting the manuscript. All authors read and approved the final manuscript.
Not applicable.
Authors acknowledge the groups whose literatures have been cited to write this review.
This research did not receive any specific grant from funding agencies in the public, commercial, or not-for-profit sectors.
The authors report no relationships that could be construed as a conflict of interest.
ACVD, atherosclerotic cardiovascular disease; AMPs, antimicrobial peptides; APS, antiphospholipid syndrome; BCNE, blood culture-negative endocarditis; CAD, coronary artery disease; CGD, chronic granulomatous disease; CMDs, cardiometabolic diseases; CVD, cardiovascular diseases; FAdV-4, fowl adenovirus serotype 4; GILZ, glucocorticoid-induced leucine zipper; GLP-1, glucagon-like peptide 1; HCV, Hepatitis C virus; HFD, heightened high-fat diet; HPS, hydropericardium syndrome; hsCRP, C-reactive protein; IL, interleukin; MAMPs, microbe-associate molecular patterns; LPS, lipopolysaccharide; PRR, pattern recognition receptors; SCAD, stable coronary artery disease; SCFAs, short-chain fatty acids; TMA, trimethylamine; TMAO, trimethylamine N-oxide; UA, unstable angina.