Dendrimers are three dimensional, multifunctional polymeric structures which are composed of a core and branches emanating from these structures, resembling a tree. Here, we review the key highlights of the dendrimers for diagnostic uses as MRI contrast agents, X-ray computed tomography and particularly for cancer treatment as an effective carrier for drug delivery and in boron capture therapy. We will discuss how dendrimers represent a new class of carriers that are able to deliver drugs effectively and in a sustained manner to the target tumor sites. Dendrimers are being utilized to a variety of cancer therapies to improve the safety and effectiveness of anticancer therapeutics. This review will also investigates the benefits utilizing dendrimers for cancer diagnosis and therapy.
Both natural (Liposomes, SLN, chitosan, NLC) and synthetic nanocarriers (dendrimer, quantum dot, fullerenes) have found multiple uses in diagnostics such as use in bio-imaging for designing better contrast agents and in cancer chemotherapy for enhanced drugs, genes and macromolecular delivery to the tumor sites (1-2).
Dendrimers, as a unique class of synthetic polymers, play an important role in nanotechnology. The term dendrimer is derived from the Greek word “Dendron” which means trees and “Meros” means a branching unit, which has logical views of its typical 3D tree-like structure as shown in Figure 1. Dendrimers were first synthesized by two research groups; Buhleier et al. and Tomalia et al. during 1970 to 1990. The macromolecular structure of dendrimers has significant characteristic features, such as high branching unit, nano-metric size, three-dimensional shape, and monodispersity. Dendrimers are also known as cascade molecules, arborols, and nano-metric architectures. The dendrimers are synthesized from the simple monomer unit, in a distinct and controlled fashion, resembling a leaf (surface group) joined to the trunk (branching unit). Dendrimers are nano-sized drug delivery macromolecules constituted of three distinct domains: i) a core consisting of at least two identical chemical groups, ii) branches developed from the multifunctional core, and iii) large number of functional groups located on the surface of dendritic architecture. The properties of dendrimers such as nanometric size and controlled architecture with tailor made functionalities favor an edge over the linear polymer and polymer micelles. The availability of number of surface groups (-NH2, -OH, and –COOH), well-defined architecture, and globular shape provides a template for the bioactive delivery and biosensing applications (3). The characteristic features like chemical stability, self-assembly, polyvalency, low cytotoxicity, modest surface area, exterior functional groups, solubility enhancement, and host-guest supramolecular assembly are the outcomes of its chemical environment (hydrophobic interior and hydrophilic exterior along with low polydispersity index) (4). Each layer comprising of highly organized branching units in dendrimers is called as “generation”. Dendrimeric generation starts from the core itself considered as -0.5 generation up to 10th generation depending on different core and terminal surface group. Up to 5th generation, the particle size of dendrimers was observed to be less than 10 nm. The branches consist of repeated units having at least one branching spot, whose repetition grows exponentially. The properties of the dendrimers are determined by the presence of number of surface groups (5).
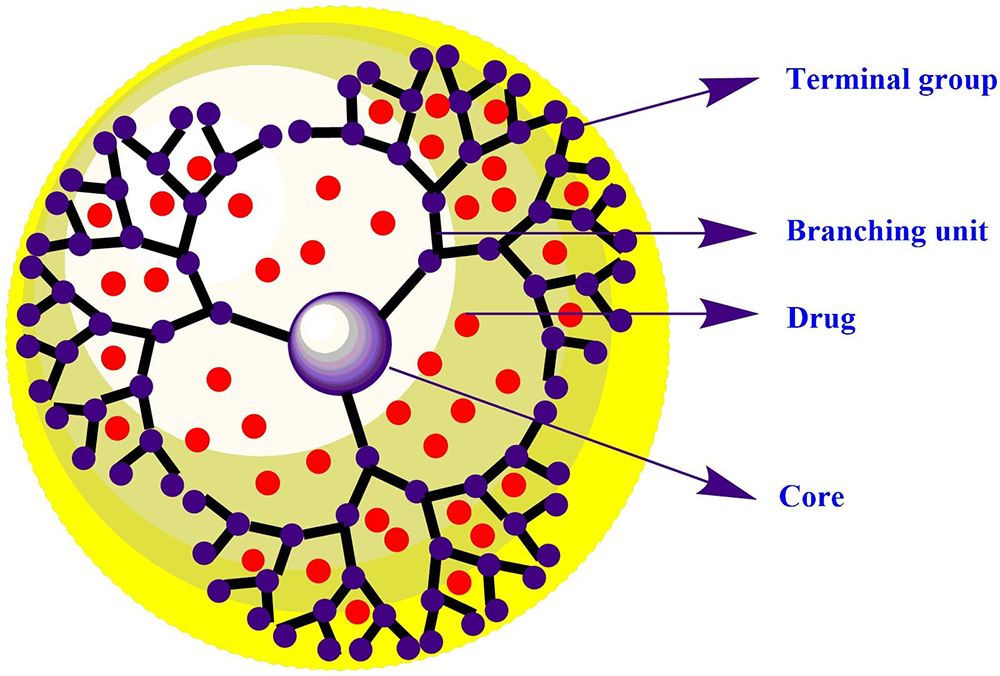
Schematic representation of drug loaded dendrimers.
The potential of dendrimers depends on electrostatic and hydrophobic host-guest interactions between dendrimers and drug molecules. Jansen et al. explained the concept of dendrimers as a dendritic box (6). The recent developments and availability of expanding number of publications in the field of dendrimers urged the exploration of diverse dendrimers for the delivery of bio-actives, genes, enzymes, and radiolabeled molecules. Applications of dual drug delivery systems is another aspect that is being widely explored by researchers for multifaceted delivery of medicinal and natural agents. Researchers also found that dendrimers could be the first choice for the dual targeting to the tumor site because of their characteristic features. Conjugation and encapsulation strategies have made it possible as depicted in figure 2 (2). Moreover, the molecular probe with the nanocarrier is stained with chemical moieties for the detection of intracellular species by means of its high sensitivity and actual-time imaging (7). These carrier systems penetrate the cell membrane through ligand mediated endocytosis. The presence of a versatile choice of groups allows the reduction in cytotoxicity and enhancement of trans-epithelial transport. Apart from biomedical field, dendrimers have demonstrated valuable contributions in the field of chemistry, material science, and electronics (8).
The diagnosis of cancer is the key step towards efficient treatment and possible cure of individual patient. Early detection of cancer increases the effective treatment options and chance of patient recovery. Current research has indicated that dendrimer-based nanocarrier might contribute to development of enhanced cancer diagnostics. There are numerous instances of radio-nuclei conjugated with dendrimers for the development of multifunctional nano-architectures to diagnose cancer. The molecular and cellular levels of biological processes can be described via in-vivo real time imaging. High fluorescence brightness, modest photo-stability, and easy modification of dendrimers are the backbones of tracking of nanocarriers using fluorescence microscopy. Dendrimer tethered fluorescent molecules and other probes help in successful real-time tracking of various biomolecules and biological events in live cells (9). Various molecular imaging modalities have been developed, such as magnetic resonance (MR) imaging, positron emission tomography (PET), and computed tomography (CT). These are regarded as the showcase of remarkable progress in biological imaging. The efficiency of in vivo imaging depends on selection of appropriate isotopes, efficient radiolabeling strategies, and beneficial pharmacokinetic profiles.
The dendrimer-based cancer therapies employ active cell-dependent tumor targeting strategies. Dendrimer-based nanocarrier utilizes well-established anti-cancer molecules in the effective management of cancer. The applicability of dendrimers is not restricted to the encapsulation or conjugation of drugs for chemotherapy. Gene transfection and novel applications of nitric oxide are exhaustively being investigated as possible dendrimer-based treatments. Boron neutron capture therapy (BNCT) is a new emerging cancer treatment modality that utilizes the neutron capture reaction of boron-10 (10B) to induce cell death. BNCT has the ability to provide the localized selectivity of radiation therapy with minimum toxicity to surrounding healthy tissues. Moreover, photodynamic therapy (PDT) is a persuasive and safe technique that is rapidly growing as a preferred treatment modality for cancer diseases. PDT requires a photosensitizer, light, and oxygen to generate reactive free radicals that oxidize biomolecules and cause cell death in the localized tissue. The key advantage over chemotherapy is the ability to localize the specific tumor treatment. US Food and Drug Administration has approved two porphyrin-based photosensitizers for PDT i.e., hematoporphyrin derivatives (Photofrin, Pinnacle Biologics, Chicago, IL, USA) and meta-tetra(hydroxyphenyl)chlorin (Foscan, Scotia Pharmaceuticals, Stirling, Scotland) (10). Photosensitizers have limited solubility in water and thus require a suitable pharmaceutical formulation for parenteral administration. The current review focuses on the recent advances in the diagnostic and therapeutic applications of multifunctional dendrimers in the effective management of cancer.
Dendrimers possess several characteristic features that make them unique among other inorganic nanocarriers. Few properties are highlighted below to showcase their versatile behavior in the diagnosis and treatment of cancer.
Dendrimers belong to the class of dendritic polymers that consists of a well-defined molecular structure, unlike the linear polymers. This offers scientists an opportunity to deal with a tool for a distinct and reproducible product development within a scalable size (2). The bimolecular dendrimers are generally obtained by the convergent synthesis method due to the frequent purification at each step. Also, the monodisperse dendrimers (G1.0-G5.0) are significantly produced by the divergent method. The incomplete removal of ethylenediamine is a major factor that may affect the degree of mono-dispersity of dendrimers. The subsequent generation of dendrimers functions as an initiator core to produce the next generation that may lead to polydispersity. There are various methods available to confirm the mono-dispersity, such as size exclusion chromatography (SEC), gel electrophoresis, mass spectroscopy, and transmission electron microscopy (TEM).
Dendrimers of different classes are synthesized by using different cores as well as branching units. The three-dimensional structure of dendrimers suggests that the lower generation dendrimers possess open and amorphous structure whereas the higher generation dendrimers possess spherical or globular conformation. This property of dendrimeric structures is the reason for higher drug incorporation in the void spaces of higher generation dendrimers. The nanometric size of dendrimers makes them promising to cross the cell membranes as well as lower the risk of clearance from the body. With the increase in their generation number, the size of dendrimers also increases. There are number of biological structures that are quite similar to the dendrimeric structure, e.g. the size of hemoglobin is similar to the size of G-5.0 dendrimers (11).
The dendrimer structure grows systematically with the increase in generation. Hydrophobic internal cavities allow the encapsulation of hydrophobic compounds in its internal structure. Solubility enhancement of hydrophobic compounds is an important property of dendrimers for which these are considered as one of the most useful nanocarrier in the drug delivery. The availability of free void spaces increases with the generation allowing the accommodation of various guest molecules in their structure. Although, terminal groups play a vital role in the solubilization of hydrophobic drug moieties. The solubility of drug moieties in the dendrimer is increased by several folds due to its hydrophilic nature.
Dendrimers are the smart nanocomposite that are capable of targeting a specific tumor sites, crossing the biological barriers, and increasing circulation time of the active moiety. The Cationic dendrimers especially polyamidoamine (PAMAM) and polypropylene imine (PPI) dendrimers consists abundant inherent terminal surface groups which are surface engineered with ligands, radiolabeled elements, and drugs as depicted in Figure 2. This modification makes the cationic or partial charged dendrimers as biocompatible dendritic nanocarrier. Various research groups reported that PAMAM dendrimers exhibited generation dependent toxicity as these nanocarriers adhere to the cell membrane and lead to the damage of the membrane resulting cell-lysis. The in-vitro and in-vivo studies revealed that surface modified dendrimers possess minimal toxicity towards the normal cells.
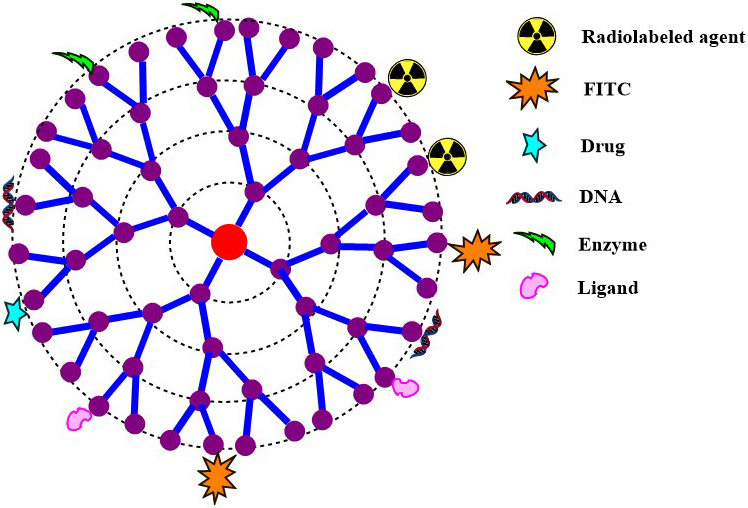
Schematic diagram representing the multifunctional surface modified dendrimers useful for their diagnostic prospective and anticancer activity.
Dendrimers consist of three structural units i.e. core, branching units, and number of surface functional groups. The peripheral groups play a vital role in the exploration of the dendrimer as drug delivery vehicles. The cationic dendrimers such as poly-l-lysine, PPI, and PAMAM can form complexes with DNA or RNA biomolecules in the disease prognosis. Polyamidoamine (PAMAM) and polypropylene imine (PPI) dendrimers have exhibited escalated concentration-dependent toxicity due to its cationic nature, unlike anionic and partial dendrimers which showed a lesser toxicity. Irrespective of the drug delivery efficiency of cationic dendrimers, there are several drawbacks, such as cytotoxicity and hemolysis. Fortunately, surface modifications with chemical moieties like carbohydrate, PEG and acetates overcome this inherent toxicity of cationic dendrimers.
Dendrimers consists of three major parts i.e. a core, the branching units and terminal surface groups. Dendrimers can be grown with varying functionality to provide distinct features, such as thermal stability, hydrophobic regions for solubility enhancement, etc. There are two different methods of dendrimeric synthesis, i.e. convergent divergent synthesis.
The convergent growth strategy was pioneered by Hawker and Frechet. In the convergent approach, the dendrimers are synthesized in a stepwise manner, growing from the terminal groups and progressing inwards. The growing polymeric branched arms, usually called “dendrons”, are attached to a core moiety. The intermediate structural unit before attachment to the core is called as “wedge”. Usually three to four wedges are attached to the core. The wedges consist different functional groups at the terminal surface groups. The purification can be done quite easily at each step of the synthesis. The superiority of this method includes lesser number of simultaneous reactions leading to less structurally deficit byproducts. Some of the additional distinguishing properties are the difference in masses of the products (desired and byproduct) and comparatively large size of the desired products. To reduce the number of synthetic and purification steps with escalated yield, alternative synthesis approaches like double-stage convergent growth method are developed.
In the early 1980s, two research groups, namely Tomalia et al. and Newkome et al. developed the divergent approach to synthesize dendrimers. This is the most used approach of dendrimer synthesis as fewer impurities are produced in the reaction steps. The synthesis process starts from the trunk towards the leaf, which leads to the construction of a controlled building block towards its periphery. This method of synthesis is based on layer-by-layer designing, which forms a new generation of dendrimers. The divergent approach depends some factors, like building block coupling and modification of the terminal surface group by deprotonation. The first generation of the dendrimers is developed by simple attachment of the branching unit to the core. Further, the next generation is built from the terminal groups of the former generation. The major advantages of the divergent approach are: i) each step of the reaction is controllable and ii) this offers protection to the other functionalities of the dendritic architecture. However, the synthesis of higher generation dendrimers is a difficult task to achieve as number of simultaneous reactions are needed to be performed within one molecule. The purification of the desired product is another hurdle in this synthesis protocol for higher generation dendrimers.
Polyamidoamine (PAMAM) dendrimers are generally synthesized by the divergent approach. This category of dendrimers consists of ammonia or ethylene diamine as the central core. PAMAM dendrimers are available in different generations (G 0-10) with different terminal functional groups. The unique properties like well-defined three-dimensional structure and mono-dispersity are the major reasons for their versatility in diagnostic and therapeutic applications. The drawback related to toxicity can be overcome by the surface modification with PEG, polyethylene oxide (PEO), carboxylic acid, and acetic acid. Currently, PAMAM dendrimers are the most studied one among dendrimers family as nanocarrier, and explored as a vector for drug targeting, gene delivery, and other biomedical applications. Vivagel® is the marketed formulation clinically (phase-3) approved by FDA in 2011, which consists of PAMAM dendrimers for the treatment of Bacterial Vaginosis (BV), HIV disease.
Polypropylene imine (PPI) dendrimers consists of poly-alkyl amine in its terminal periphery and abundant tertiary tris-propylene amines present in the interior structure. These dendrimers are available up to the fifth generation. There are evidences of their use in drug delivery as well as gene delivery. Many researchers concluded that the interior hydrophobic regions permit higher solubilization of lipophillic drugs in comparison to the PAMAM dendrimers. Jansen et al. reported that PPI dendrimers consist of dendritic boxes in which guest molecules, like drugs, genes, etc. get entrapped. The above mechanism restricts the diffusion of bioactive molecules from dendrimers. PPI dendrimers are commercially available as AstramolTM.
Peptide dendrimers are macromolecules consisting of peptidyl branching core and peptide chain at the terminal surface (12). These are subdivided into three different classes. i) When the peptide groups are present at the periphery surface, those are known as the “grafted” peptide dendrimers. ii) The second class includes the amino acids utilized at both branching core and terminal surface groups. iii) The third class involves the non-peptide branching core and peptide at the terminal functionalities. Peptide dendrimers have proven their utilities in the field of diagnosis, vaccine delivery, and gene delivery. These are also used as surfactants in the chemical industry (13). In addition, Darbre and co-workers have developed esterase catalysts utilizing peptide dendrimers (14).
Hybrid dendrimers are the hybrid building block in which linear polymers are grafted to dendrimers. The characteristic features such as globular shape and presence of numerous functionalities are supportive in the construction of hybrid dendritic architectures. One section of the dendritic architecture is coupled with the reactive end chain of the polymer, which is further utilized for the conjugation of the surface-active agent, biodegradable, biocompatible or bio-adhesive polymers (15). The hybrid dendrimers are found to have minimal clearance from the biological systems by crossing the reticuloendothelial system (RES). Surface modified hybrid dendrimers are also being explored for numerous aspects of drug delivery (16).
PAMAMOS dendrimers are also known as inverted unimolecular micelles. PAMAM (hydrophilic, nucleophilic) interior and organosilicon exterior are the characteristics constituents of the radial layer of PAMAMOS dendrimers. These dendrimers are utilized for novel applications, such as photonics, nano-lithography, and chemical catalysis. There are several advantages of PAMAMOS, like steadiness in their structure, nanometric size precision, complex formation ability, and entrapment of the guest molecules (17). These smart versatile dendrimers have broad applications inflating continuously in drug delivery.
Dendrimers have been explored for their application in the diagnosis of cancer. Dendrimers act as a carrier for different diagnostic agents, like PET contrast agents, X-ray contrast agents, MRI contrast agents, etc. as depicted in Figure 3.
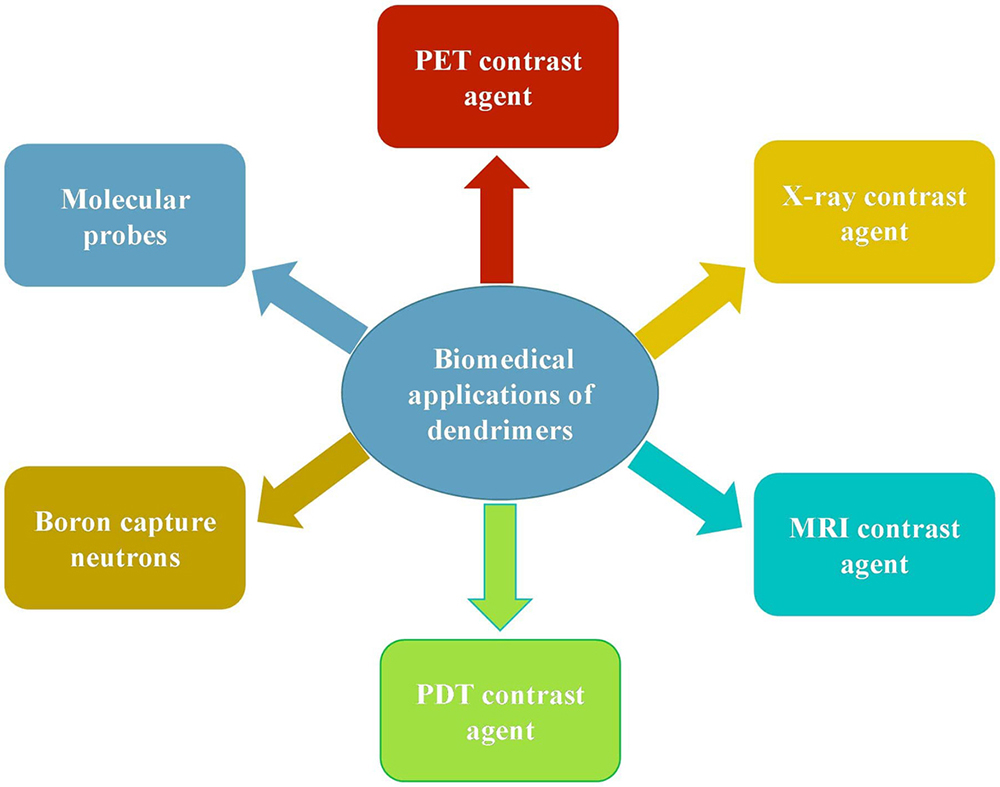
Various applications of versatile dendrimers as a diagnostic agents as well as therapeutic agents.
The X-ray imaging contrast agents play a vital role in the Magnetic Resonance Imaging (MRI) technique. MRI contrast agents possess characteristic features like biocompatibility, low toxicity, and high relaxivity. Clinically approved contrast agent such as gadolinium (Gd III) is extensively utilized as an MRI contrast agent for the diagnostic purpose. The strong affinity of Gd (III) is considered as its major drawback, which ultimately causes the serum protein toxicity during therapy. Another complex reagent like diethylene triamine penta-acetic acid (DTPA) is also clinically being utilized as a contrast agent (18). Researchers also suggested that MRI contrast agent of high molecular weight is always preferred for diagnostic purpose in comparison to the low molecular weight one due to the leakage of the later in the interstitial space through the blood vessel. To resolve this problem, Gd (III) is tethered or conjugated with high molecular weight macromolecules like polylysine, albumin, dextran, and dendrimers. (19). Smart nanocomposite like dendrimers are categorized into a new class of high molecular MRI agents. These molecular agent encapsulated dendrimers can be decorated with highly specialized active targeting molecule that will be selectively taken up by specific cells (Figure 4).
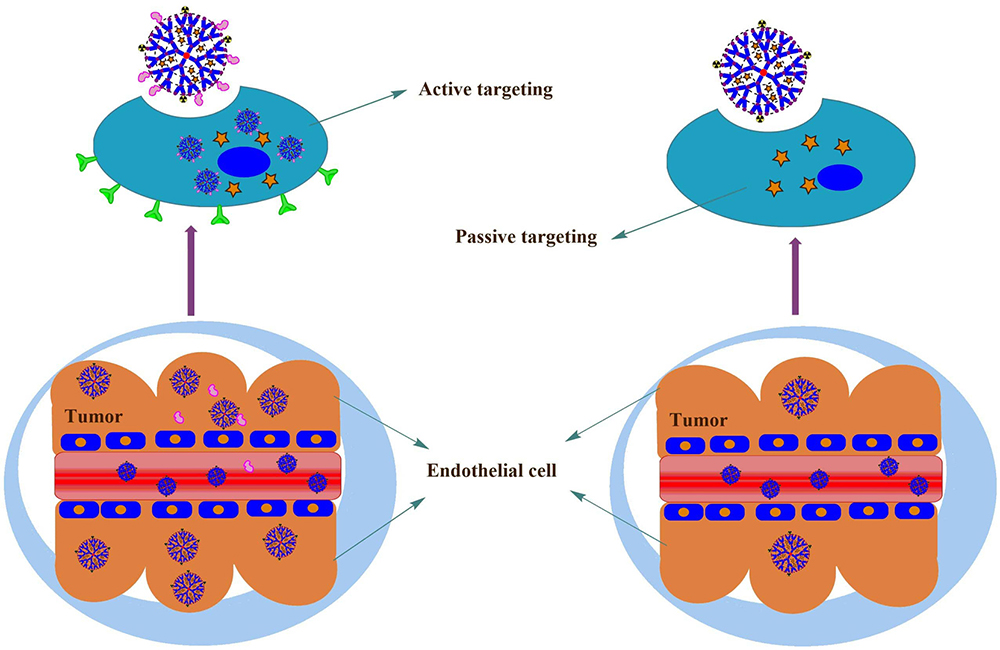
Schematic representation of active and passive targeting of dendrimers. Ligand tethered dendrimers exhibit the active targeting through the overexpressed receptors in the tumor sites, whereas dendrimers (without ligands) exhibit the lesser accumulation in the tumor sites.
In the early 1990s, researchers developed a dendrimer-based MRI contrast agent for the first time to be used in the in-vivo diagnostic imaging application. The diagnostic aspect of G4-PAMAM dendrimers tethered Gd (III) contrast agents were evaluated in animal models (figure 5). The chelate gadolinium attached to the dendrimer, namely Magnevist ®, exhibited excellent blood circulation properties and modest relaxivity value. Due to the unique magnetic properties of PEG grafted G4.5-Gd2O3 nanoparticles, the prepared nanoparticles exhibited significant T1−T2-weighted phantom MRI imaging ability compared to clinically used contrasting agents (Gd-DTPA or Gd-DOTA) as depicted in figure 5. The relaxivity was escalated by six folds in dendrimer tethered Gd (III)-DTPA complex in contrast to free Gd (III)-DTPA complex. The results of in-vivo MRI analysis indicated that the prepared nanomaterial is an ideal candidate for dual MR imaging (20).
Weiner et al. developed a unique class of dendrimers based Gd (III)-DTPA contrast agent as a diagnostic agent. Dendrimers of higher generation were linked to the ligand 2-(4-isothiocyanate-benzyl)-6-methyl-DTPA through thiourea linkage for the selective tumor targeting. In vivo studies concluded that i.v. injection to the rabbit enhanced the blood-circulation time (100 min) of conjugated dendrimer with former contrast agent. The result was visualized and confirmed by the MR images of blood vessels in the animal model. Kobayashi et al. found similar kind of favorable results in the other class dendrimers e.g. PPI dendrimers conjugated to Gd (III)-DTPA (21).
Researchers revealed that the relaxivity of the contrast agent depends on the size of dendrimers of the higher generation (sixth generation). The cationic poly-l-lysine dendrimers were conjugated with tetra-azacyclododecane-tri acetic acid, which is now used for the clinical blood pool imaging (22). The nanofabrication of the target-specific moieties to the dendrimer-based contrast agents improves their pharmacokinetic profile. Weiner and co-workers synthesized ligand-based Gd (III) contrast dendrimers, which increased the relaxation rate in tumor cells due to its high expression in specific folate receptors (23). Previously used MRI agents have shown the potential to improve in-vivo efficacy by complexing dendrimers with DPTA. Earlier, different generations of dendrimers were anchored with radiolabeled compounds and further determined the bio-fate of drug molecules in animal model. The outcomes revealed that dendrimers of higher generation had longer circulation time compared to lower generation, as the former had lower excretion through kidney. The larger size possessed by higher generation dendrimers allowed them to be retained in the vessels for a longer period. It also resulted in the highest signal: noise ratio in contrast to the GD-(DTPA)–Dimeglumine imaging agent. The deepest lymph nodes and the thoracic duct were easily visualized by three-dimensional micro MRI with PAMAM-G8.0 as compared to GD-(DTPA)-Dimeglumine (24).
Computed tomography (CT) is the analysis tool that permits researchers for capturing three-dimensional images of a specific section of the tissues. It is one of the most frequently utilized diagnostic tool in the health care sector. X-ray is the electromagnetic radiation lies in the range of 0.1 to 10 nm. In the diagnosis of several diseases like arteriosclerotic vasculature, tumors, infarcts, and kidney dysfunctions, acquired high-resolution images are captured with the assistance of X-ray contrast agents. Like MRI agents, the X-ray agents can also easily permeate through the blood vessels and reside between the extravascular and intravascular fluid components in the body. The dose of the X-ray contrast agents lies in nanomolar ranges to visualize the tissues in the body. The vascular imaging of the bloodstream is achieved by the non-ionic iodinated compound having concentration equivalent to half of the other contrast compound. Better quantitative detection of the disease lesions is achieved through the high molecular weight (less than 200 Da) contrast agents. Earlier, iodinated media was used as a contrast media and injected as a radiopaque agent in CT imaging diagnostic technique. The rate of excretion of iodine is quite high during treatment which is a major challenge in CT imaging.
There are numerous natural polymer-based nanocarriers like liposomes, micelles, and micro- and nano-emulsions which could tagged or complex with the CT imaging agent. Organic or inorganic nanoparticles (gold, dendrimer, fullerenes, and silver) mediated CT contrast agents can prolong their blood circulation time. Recent studies suggest that dendrimers have great potential as polymeric X-ray contrast agents due to their distinct characteristic features, such as high X-ray attenuation, excellent water solubility, lesser osmolarity, hydrophilicity, and good chemical stability. Dendrimers are also observed to have prolonged intravascular bioavailability in animal models. Dendrimers are the best nano-scaffold for next-generation iodinated contrast agents by virtue of the suitable numerous surface groups as well as safe use of iodine. PEGylated dendrimers tagged with iohexol exhibited better tumor angiogenesis as compared to pure iohexol in the animal models because of low toxicity and blood pooling. Dendrimers are the key players in the biomedical research due to their synthetic macromolecular globular shape even after those get coupled with the numerous ligands or radiolabeled moieties (25). In order to evaluate the ability of targeted dual mode CT/MR imaging of tumors, the targeted Gd-Au DENPs-FA and the non-targeted Gde Au DENPs ([Au] ¼ 0.1 M, 200 mL) were intravenously injected into two groups of nude mice bearing KB xenograft tumors via tail vein. CT images were captured before (0 h) and after injection (2, 6, and 24 h) using a Micro-CT system as depicted in Figure 6 (26). The radiopaque moiety, tetra-iodobenzene derivative was attached to the dendrimers moiety for the first time to visualize its blood circulation pool time. Other class of dendrimers, like starburst PAMAM dendrimers tethered with the tri-idobenzene derivative (water-soluble) exhibited its prolonged blood half-life (27). Moreover, poly-lysine dendrimers were tagged with iobitridol (trade name Xenetix) contrast agent at each terminal group of its dendritic architecture. The use of high M.W. imaging agent with dendritic structures has exhibited extended blood half-life and increased the peak X-ray attenuation at the time-interval of diagnosis. Their accumulation in liver and spleen were also increased by the reticuloendothelial system.
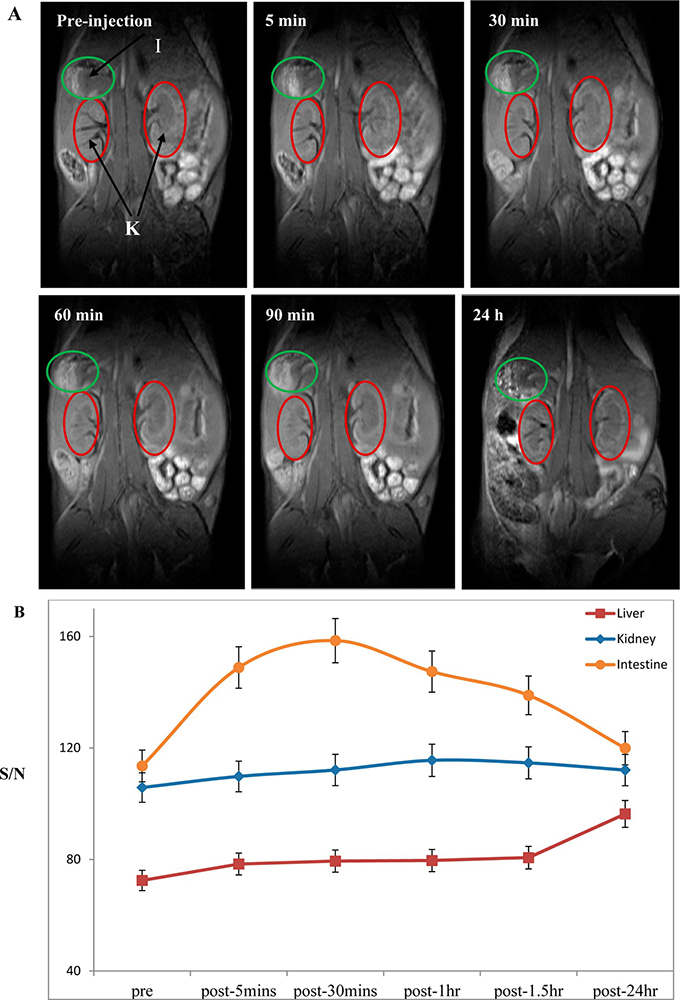
Coronal T1-weighted MRI images of mouse after intravenous injection of G4.5.-Gd2O3-PEG NPs at 0, 5, 30, 60, and 90 min and 24 h: (A) kidney (K) and large intestine (I) and (B) signal-to-noise ratio (SNR) of the regions of interest (ROIs) with respect to injection time. Error bars were based on triplicate measurements. Used with permission from (20).
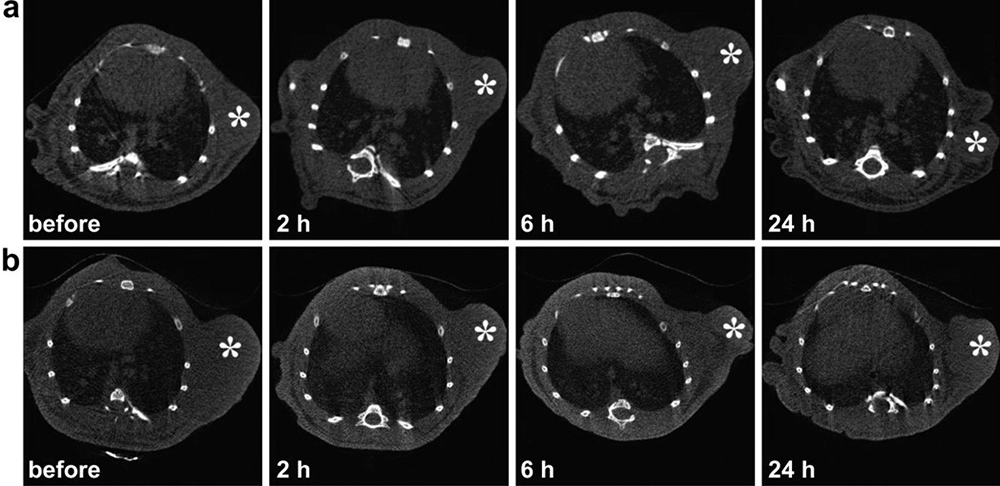
Representative transection CT images of mice bearing transplanted KB tumors after intravenous injection of non-targeted GdeAu DENPs (a) and targeted GdeAu DENPs-FA (b) for 0, 2, 6, and 24 h, respectively. The white star indicates the tumor area. Used with permission from (25).
Dendrimers hold immense potential that could be utilized in angiography. In X-ray computed tomography imaging, differentially attenuated X-ray beams are developed with the assistance of photoelectric effect in order to visualize, particularly tissues and organs throughout the body. Iodine and barium are capable of achieving the targeting attenuation in the diagnosis of diseases. Earlier in CT imaging, a higher concentration of iodine was required for the detection due to its sensitivity issues. The high dose requirement of the iodine (30-35 g for adults) may have a risk because of the rapid release of the small molecules from the blood vessels through kidney globules. The contrast agents consisting of 1,3,5-triidobenzene derivatives are available in two forms; either monomeric (e.g., iohexol and iopromide) or dimeric (e.g., iodixanol). The amide and alcohol functionalities make them biocompatible and water-soluble X-ray imaging agent. Gaikwad and co-workers reported that radiolabeled iodine tagged with different generations of dendrimers could be utilized for better visualization, which was helpful in identification of in-vivo pharmacokinetics, bio-distributions, and in tumor accumulation study (28). There are several drawbacks associated with this technique, such as disturbed structural arrangement in the physiological condition, expensive treatment, heterogeneity, and the intrinsic toxicity.
Dendrimers are utilized as fascinating molecular probe due to their specific features and precise morphology. The water solubility of cationic dendrimers like PAMAM, PPI, and Poly-lysine dendrimers prevents the interaction of biomolecules in the bloodstream due to encapsulation in its interior architecture. The high density and larger surface area allow immobilization of the sensor units on the surface of dendrimers which helps in designing of integrated molecular probes. High-sensitive sulfur dioxide was conjugated with different dendrimers in Pt-coordination complex fashion, which was reported by van Koten. The significant modifications on the surface of dendritic architecture defines their building block potential for the biomimetic artificial membrane and other electric devices. Astruc et al. reported the synthesis of amino-ferrocene dendrimers and described the surprising outcomes through the dendritic effect. The hybridization of signal amplification was accessed through the DNA and RNA fabricated dendrimers in the in-vivo model studies. The improvement in the DNA microarray technology is achieved by signal amplification caused by the fluorescent oligonucleotide dendrimers. Oligonucleotide dendrimers are approved to be the backbone of polylabelled DNA.
The presence of chiral amino alcohol groups recognized the signal amplification by utilizing the phenylene ethynylene-based fluorescent conjugated dendrimers. 1,1-bi-2-naphthol molecule significantly increased the fluorescent effect of the chiral organic molecules during microanalysis. PEGylated dendrimers were conjugated with tetrabenzoporphyrins to form stable Pd complexes (29). This surface engineered biocompatible dendritic approach is quite useful for in vivo oxygen imaging. The characterization of the former was analyzed through the phosphorescence emission at 800 nm depending on availability of oxygen. Thereafter, the pH sensing ability of the metal-free tatrabenzoporphyrin were used to produce a pH-responsive spectral by virtue of change in absorption and emission.
Cellular uptake imaging is usually performed using fluorescence detection approaches and can be analyzed qualitatively and quantitatively. This approach is quite helpful in overcoming drawbacks of non-biocompatible conventional techniques. The advantages of this technique are non-invasiveness, limited tissue absorption, scattering of the light by virtue of excitation of the fluorescent material, and localize the emitted fluorescence. In this in vitro technique, the detection system permits the quantification of fluorescent material with biosensor assistance to one-photon fluorescence. The one-photon and two-photon detection systems are utilized for the wavelength excitation of broad length for the detection of the simultaneous fluorochromes. Moreover, this technique is limited to only scattering of the light and absorption in the tissues.
Some of the investigators have reported that the two-photon optical fluorescence fibers (TPOFF) system uses single-mode fiber to transport femtosecond laser pulse for excitation as well as the collection of the emitted tissue fluorescence. The presence of abundant surface groups allows tagging or conjugating of the fluorescent dye to estimate the quantitative cellular internalization of smart nanocomposite dendrimers. The fluorescent nanoparticles are injected into the mice for in vivo real-time fluorescence measurement. The TPOFF probe for the fluorescent dyes is extensively used for cellular uptake study on various cancer cell lines. Researchers employed the 5.0 G dendrimer-folic acid conjugate along with fluorescent sensing 6-TAMRA (6T), which was intended to target xenograft tumors in the mice model. The TPOFF probe and a thin gauge needle were sufficient to quantify the uptake the fluorescent agent in the tissues. Iacobazzi et al. developed the asialoglycoprotein receptor (ASGP-R) targeted PAMAM G 4.0 dendrimer with the help of Lactobionic acid (La) conjugation followed by acetylation. The evaluation of synthesized conjugate loaded with sorafenib and grafted with FITC confirmed higher uptake ability in HepG-2 liver cancer cells (30). Uram et al. studied the glucohepto-amidated dendrimer cellular uptake by tagging FITC and DAPI dye in U-118 MG, BJ, and SCC- cell lines as shown in figure 7 (31).
Several common radionuclides, such as 11C, 13N, 15O, and 18F atoms are clinically utilized for PET imaging. The short half-life (2-20 min) of these radionuclides is the main reason for their least utilization. In addition, researchers reported only a few radiolabeled NPs. Dendrimers having pentaerythritol as a central core and modified with peripheral tyrosine groups can be coupled with radiolabelled 76Br. It is a method having a quick effect with higher sensitivity. Among all radionuclides, 18F is considered as a good positron emitter for PET imaging and has exhibited previously distinct clinical applications. There are numerous strategies developed for the successful synthesis of 18F labelled NPs. However, conjugating 18F to a macromolecule is still challenging in many ways. For the first time, Trembleau et al. reported a synthetic scheme of 18F-tagged dendrimers at room temperature (32). Biotin was anchored to the peripheral surface of dendrimers by disulphide bonds. The outcomes of in-vitro studies proved that biotin tagged with dendrimers has shown high selectivity and specificity towards HER-2 expressing cells. Researchers found that 18F and 76Br have relatively long half-lives and labelling the same with macromolecules can increase the selectivity, lessor toxic effects on normal tissues and organs. The chloramine-T method utilized for 76Br tagged antibody with higher yield outcomes is reported by numerous researchers (33). Almutairi et al. designed biocompatible dendrimer tagged 76Br for angiogenesis through PET imaging (34). Also, dehalogenation of dendrimers can be prevented by conjugating the former with polyethylene oxide (PEO) as they form a preventive shell to bypass RES uptake. Further, arginylglycyl aspartic acid (RGD) peptide with modified lysine was attached to the terminal groups to increase the specificity to the tumor sites. The dendritic targeting probes showed six times modulation in endocytosis through the αvβ3 receptor.
Due to their controlled half-lives and convenient radiolabeling protocols, 64Cu and 68Ga radiometals are exclusively researched and utilized for the development of radiolabelled NPs for PET imaging. A cyclotron accelerator is required for the production of 64Cu and 68Ga from the 68Ge/68Ga generator. Wang et al. reported that the dual-approach imaging of ovarian cancer developed a novel affibody anti-HER2 based dendritic probes used for diagnostic purposes. PAMAM dendrimers were conjugated with both 64 Cu and cy5 and in-vivo studies suggested higher tumor accumulation after administration of i.v. injection due to its favorable pharmacokinetic profile. It was confirmed through desired tumor imaging events observed within 20 h. In order to improve the structural stability of dendrimer in blood circulation, four cysteines were introduced to the oligolysine backbone of the telodendrimers and then crosslinked via disulfide bond. Due to the structure feature of porphyrin components, the self-assembled telodendrimers possessed an intrinsic ability to chelate 64Cu for PET imaging or Gd (III) for MR imaging (Figure 8)(35).
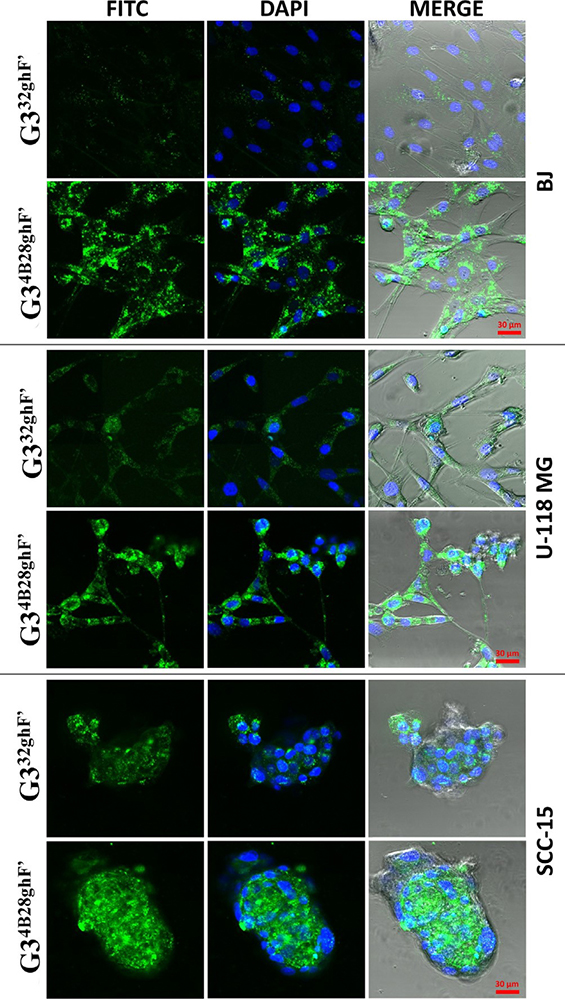
The cellular accumulation of 1.0 lM G332ghF0 or G34B28ghF0 dendrimer conjugates by BJ, SCC-15 and U-118 MG cells after 24 h incubation. Green signal is from FITC labeled G332ghF0 or G34B28ghF0 dendrimers (left column), blue comes from DAPI stained nuclei and merge additionally with contrast phase. Used with permission from (31).
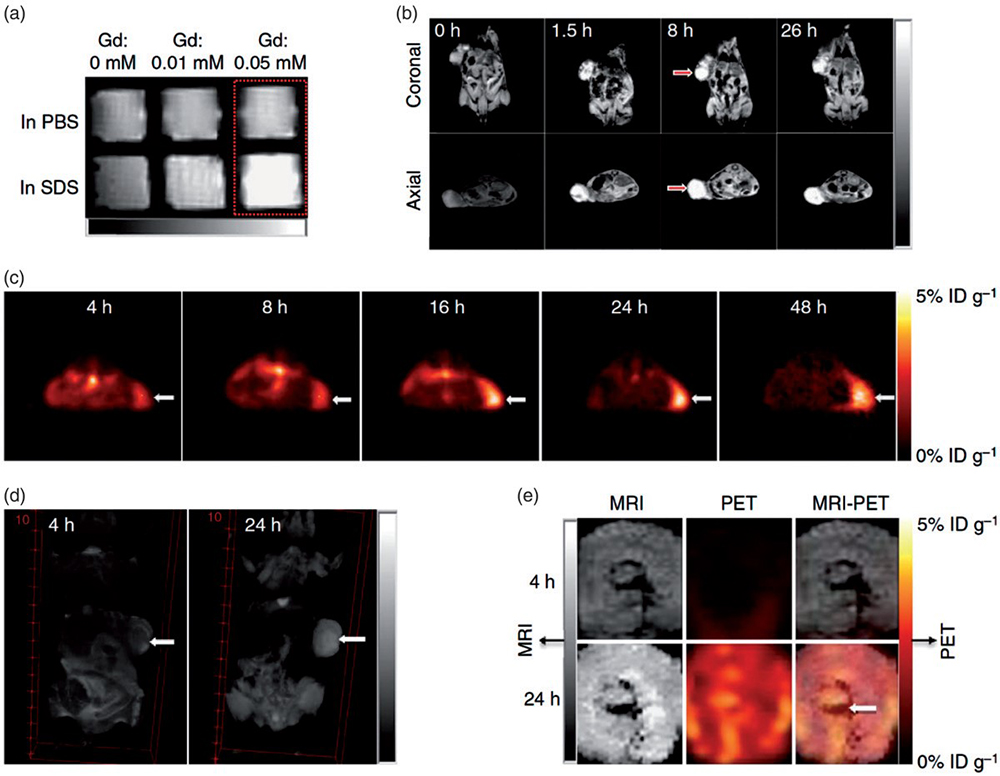
Nanoporphyrin-mediated MRI and PET imaging in animal models. (a) In vitro MRI signal of Gd-NPs in the absence and in the presence of SDS obtained by T1-weighted MRI on a Bruker Biospec 7 T MRI scanner using a FLASH sequence. (b) Representative coronal and axial MR images of transgenic mice with mammary cancer (FVB/n Tg(MMTV-PyVmT) using a FLASH sequence preinjection and after injection of 0.1.5 ml Gd-NPs (Gd dose: 0.0.15 mmolkg1 ). The white arrow points to the tumor site. (c) PET image of nude mice bearing SKOV3 ovarian cancer xenografts at 4-, 8-, 16-, 24- and 48-h post-injection of 64Cu-labeled NPs (150–200 ll, 64Cu dose: 0.6.–0.8. mCi). The white arrow points to the tumor site. (d) 3 D coronal MR images of nude mice bearing A549 lung cancer xenografts using a FLASH sequence at 4- or 24-h post-injection with 0.1.5 ml of 64Cu and Gd dual-labeled NPs (150–200 ml, 64Cu dose: 0.6.–0.8. mCi, Gd dose: 0.0.15 mmol kg1 ). The white arrow points to the tumor site. (e) PET-MR images of tumor slices of nude mice bearing A549 lung cancer xenograft at 4- or 24-h post-injection of dual-labeled NPs. White arrow points to the necrotic area in the center of the tumor. Used with permission from (35).
During the period of investigation, the tumor fluorescence intensity is escalated whereas, in the case of PET scan, radioactivity is observed at 4 h of post-injection. They utilized the same for diagnosis and treatment of diseases through photodynamic therapy (PDT), photothermal therapy (PTT), PET, and CT guided drug delivery. Other hybrid nanocomposites were designed using 64Cu metal and proceeded for PET as well as Gd (III) MR imaging. The addition of sodium dodecyl sulphate (SDS) to the telodendrimers shows strong red fluorescence emission at 680nm, which converts the light into photodynamic transduction. It is observed to have sustained release of chemotherapeutics from the tectodendrimers, which is confirmed in both ovarian xenograft and murine transgenic breast models. The significance of PET nanoparticles in the biomedical fields depends on the pharmacokinetics of NPs and half-lives of the positron-emitting isotopes. Hou et al. reported that 64Cu tagged supramolecular dendrimeric architecture could be used for targeted PET imaging (36). Trans-cyclooctene (TCO) can be conjugated with tetrazine DOTA-64Cu (64Cu-Tz) through the Diel-Alder reaction mechanism. TCO groups encapsulated in supramolecular dendrimeric structure helps in avoiding in-vivo degradation of NPs and accumulation of the NPs through enhanced permeation and retention (EPR) effect. The radiolabelled dendrimeric structure with targeting ligand were found to be more specific in tumor targeting as compared to pure radiolabelled dendrimer which exhibited lesser tumor uptake and accumulation in liver.
In addition to cancer imaging, PET contrast agents are also utilized for diagnosis purpose in cardiovascular and inflammatory diseases. Clinically proven PET contrast agent like the gold standard is helpful in the treatment of ischemic disorder and myocardial infarction. Laakkonen et al. reported that a 9-amino-acid peptide, LyP-1 attached to p32 proteins behaves as a biomarker in atherosclerosis (37, 38). The higher accumulation of 64Cu tagged dendrimers with LyP-1proteins was observed in the atherosclerotic plaque with increased blood ratio found in in-vivo studies. The research group Pant et al. developed 3H and 64Cu tagged dendritic polyglycerol sulfate (DPGS) for evaluating the same for in vivo pharmacokinetic study and PET scanning, they found that in comparison to neutral dendritic polyglycerol, sulfated compounds got eliminated at a slower rate and accumulated in liver and spleen. For different degrees of sulfation, the biodistribution profile and uptake by the reticuloendothelial system (RES) was similar (39).
The Boron capture therapy (BNCT) approach has gained much more attention to the treatment of cancer. This strategy is implemented for the manipulation of boron antibody conjugate to achieved higher specific tumor localization. Direct coupling with targeting anchor (boron) not only increases the yield of the desired conjugate, but also improves its solubility, therapeutic activity, and targeting efficiency (40). It took a lot of effort and time to unveil the full potential of the dendrimers. It is found that dendrimers have a promising application for boron neutron-capture therapy in the medical field (41).
BNCT involves the generation of radiation from low energy thermal neutrons by 10B atoms. 10B atoms contain 20% natural proton, recoiling the lithium-7 which yields α-particles. The tissues are destroyed successfully by radiation energy. This strategy is utilized for the development of new treatments for inoperable tumors. The efficiency of BNCT is measured through localization of large number of 10B atoms and subsequently, absorption of adequate number of thermal neutrons in the target (diseased) tissues. In this technique, the concentration of 10B atoms must be maintained 30-50 µg per g of the tumor for the selective delivery of active moiety to the target sites (42). 10B atoms should be selective otherwise they will be toxic to the normal cells. To overcome this problem, the researchers anchored both ligands and 10B to the dendrimeric structure, which expressed in particular cancer. The well-defined structure and multi-valency prove dendrimers as proper boron carriers. The first reported boron capture neutron containing dendrimers was synthesized by Barth et al. They conjugated second and fourth generation PAMAM dendrimers to the polyhedral borane (Na (CH3)3NB10H8NCO). PAMAM dendrimers were reacted with isocyanato-polyhedral borane to make them boronated (43).
Photodynamic therapy (PDT) is the novel approach to the tumor-ablative and abruption of the functions associated with oncologic medication. PDT associated treatment is a safe, secured, worthwhile, and non-surgical technique. Dougherty and co-workers utilized PDT for the first time for targeting tumor sites. In this technique, the administered photosensitizer (PS) is stimulated by the light of a particular wavelength as shown in Figure 9. Moreover, stimulated PS activates the reactive oxygen species (ROS) which affects the tumor cell ablation due to the oxidized cellular macromolecules. PS has the ability to accumulate in the tumor sites and easily cleared from normal tissues. Another key factor is the amphiphilic nature of the PS. The hydrophilic nature helps to target tumor site and lipophilicity helps in binding to the tumor site. PS is divided into three generations. The first generation of PS consists of hematoporphyrin and porfimer sodium. The second-generation of PS includes orphyrinoid anthraquinones, phenothiazines, xanthenes, cyanines, and curcuminoid etc., whereas the third generation embraces of modified coupled PS with the biological macromolecules such as peptide/ antisense and PS tagged/ encapsulated with nanocarrier. In diagnosing cancer, the foremost drawback associated with PDT, is its poor detection capacity in solid and bulky tumors. The Monte-Carlo equation also fails to detect the tumor recurrence and residual tumor growth associated PS dose in the diagnosis of disease.
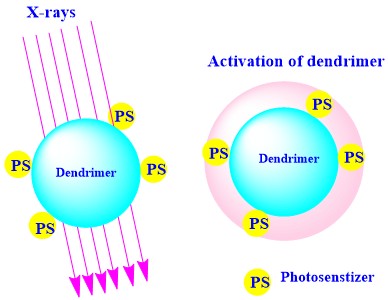
Schematic representation of the dendrimer-coupled photosensitizer (PS) activation under the influence of the X-rays, utilized for diagnosis of the site-specific tumor.
Dendrimers are the emerging vectors for the delivery of the PS. The properties like nanometric size and numerous functional groups permit the PS to get encapsulated or coupled with dendrimers. This helps to avoid the premature release and modulate the EPR effect at the tumor site (4). Klajnert et al. discussed the application of dendrimers in PDT to overcome the drawback associated with PS delivery because of the low illuminance in the tumor (45). The biofate of dendrimers are exhibited in cells, as well as tissues, is because of their globular shape even after conjugation (46). Battah et al. observed that released PS molecule led to photoexcitation, resulted in induction of 1O2 which cleaved the bond between PS and dendrimers (47). The photosensitizer conjugated with dendrimers like PPI restricted the premature release of the same in the biological system (48). Researchers found that PS tethered with nanocarrier like dendrimers form micelles through ionic interaction. Taratula and co-workers designed as an approach to encase the lipophilic silicon derived phthalocyanine into the interior of PPI dendrimers. Further, surface engineering of the dendrimers with PEG and ligand luteinizing hormone-release hormone (LHRH) peptide enhanced the specific targeting and biocompatibility (49). Dendrimer coupled PS shows the NIR absorption at 700 nm and fluorescence imaging observed at approximately 710 and 815 nm in the fluorescence emission (50).
Today cancer remains as one of the leading killers worldwide. Many promising methods for the diagnosis and treatment of cancer are currently presented in modern research, which improves the patient condition. While the primary goal of the therapies described in this review is to combat cancers. A very important secondary goal is to reduce the adverse effects that are inherent with current cancer therapies. Over the past two decades, dendrimers have been emerged as a novel class of polymers with a precise architecture and dynamic chemical structure. The recent advances in the controlled polymerization and synthesis methodology have led to the development of dendrimers with different surface functionalities. Dendrimers seem to be an effective vector for the specific delivery of cytotoxic treatments to cancer cells. Targeted therapy is an intelligent way to treat diseases that are detectable at the cellular level. The unique features result in fascinated outcomes that can deliver drugs, peptides, and diagnostic agents as depicted in (Table 1).
Type of dendrimers | Contrast agent | Diagnostic and therapeutic uses | References |
---|---|---|---|
PAMAM dendrimer | Photosensitizer agent | Dendrimer-coupled PS utilized for the target tissue site and cellular uptake | (44) |
PPI dendrimer* | Phthalocyanine | Determination of the biofate of dendrimers and their targeted delivery in specific tumor site | (47) |
Dendritic polyglycerol sulphate | 64Cu | Identification of inflammation site by utilization of the contrast agent | (41) |
PAMAM dendrimer | 18F | Selectivity and specificity towards HER-2 expressing cells proven in-vitro studies | (33) |
PAMAM dendrimer | 10 B | Potential delivery system for neutron capture therapy | (42) |
Folate-PAMAM dendrimers | TU-DTPA | Target-specific MRI contrast agent | (24) |
Tecto-PAMAM dendrimers | FITC | Site-specific imaging in the tumor cells | (50) |
PPI dendrimer | Gd (III)-DTPA | MRI images of blood vessels | (21) |
PAMAM | 76Br | Angiogenesis PET imaging | (34) |
PAMAM | PDT | Biofate of dendrimer | (46) |
Dendrimers are also the ideal nanocarriers for the delivery of ligand-mediated radiolabeled moieties to visualize real-time tissues and organs which might be used for diagnosis. Radiolabeled moiety gets accumulated in the liver and kidney due to the higher concentration, viscosity, and low osmolarity. The ideal contrast moieties are degraded in the biological systems within 24 h to reduce the toxicity. To overcome these problems, smart nanocarriers are developed to achieve specific targeting in both tissues and organs. Dendrimer mediated contrast agents helps in avoiding dose-dependent toxicity of the contrast moiety. The contrast moiety shows the sensitivity in the concentration dependent manner. The advantages of the radiolabeled dendrimers are selectivity and efficacy of contrast modalities in the biological systems. Several research groups explored dendrimers as promising nano-drug delivery systems and sophisticated smart nanocomposite used for radiolabeled tracking in anticancer diagnosis and therapies. Although issues related with toxicity of dendrimer tethered molecules and its in-vivo properties are challenges to address separately, there are considerable benefits on current applications of dendrimer in diagnosis and therapy. Proper evaluation of dendrimer-based diagnostics agent must be appreciated by virtue of three criteria: choice of contrast agent, the vector used, and the route of administration. The physiochemical properties of contrast agents are crucial but relative difference between vectors (ligand targeted vs untargeted dendrimer) are appreciated with targeting specific tumor sites. In fact, for medical applications with dendrimer-based contrast agent play the crucial role in confining contrast agents at the injection site, in order to reduce the associated radio toxicity on healthy tissues or organs.
PAMAM
Polyamido amine
Polypropylene imine
Magnetic Resonance Imaging
Boron Capture Therapy
Positron Emission Tomography
Photodynamic Therapy