Currently, there are few male contraceptive methods that are purely based on prevention of the entry of the sperm into the female reproductive tract. An alternative approach for designing reversible male contraceptive is achieved by transient testicular heating (TTH). This treatment, through massive germ cell apoptosis, causes reversible oligospermia or azoospermia. Here, we describe as how TTH causes DNA damage, oxidative stress, apoptosis, autophagy, sperm protein expression, and alters the biochemical components of seminal plasma. Further understanding of TTH will help design safe and reversible male contraception.
Ideal birth control methods for males must reduce the number of fertile sperm to reliably prevent fertilization, and such methods must be relatively long-lasting, reversible, easy to use and have no effect on the hormone secretion (1-5). Unfortunately, among currently available male contraceptive options, the reversible methods are not reliable, while the reliable methods are not reversible. Although female contraceptive methods (such as sterilization, intrauterine devices, or hormonal contraception) are very effective in preventing unintended pregnancy, some women cannot use them due to health conditions or side effects. In addition, many men wish to take active responsibility for family planning. As early as 1959, testicular heating is considered as a potential contraceptive method for men (2, 6). It is well known that functions of mammalian testes are very sensitive to temperature. Elevated testicular temperature detrimentally affects the spermatogenesis, leading to reversible oligospermia or azoospermia in animals and humans via germ cell apoptosis (7-10). The scrotum of most adult mammalian species, including humans, is a local thermo-regulator, and spermatogenesis optimally occurs at temperatures slightly lower than the body temperature (at least 2°C–8°C below core body temperature) (7, 8, 11-14). In general, the temperature of hot spring and transient testicular heating (TTH) is higher than the scrotal temperature (from 37°C to 45°C). Therefore, TTH exposure can reduce testis size, lead to spermatogenic damage, decrease sperm production, cause apoptosis of sperm and impair semen quality if the scrotal temperature is increased over time (15, 16). An elevated testicular temperature damages the seminiferous epithelium, resulting in germ cell loss as well as morphological and functional alternations in Sertoli cells (SCs) (17, 18). TTH can impair protein and RNA biosynthesis, cause oxidative stress and apoptosis, damage spermatocyte DNA, and disrupt chromatin packing in sperm nucleus (10, 12, 19). The mechanisms underlying molecular response of germ cells to TTH may involve heat-induced germ cell damage, including apoptosis, DNA damage and autophagy (13). TTH mainly leads to heat stress and affects the metabolism and apoptosis of spermatogenic cells, resulting in compromised sperm quality and impaired fertility of many animal models (20-22). These findings provide an important theoretical basis for designing male contraceptive methods (5).
The biochemical components of seminal plasma, such as fructose, neutral α-glucosidase (NAG), carnitine, nitric oxide (NO), nitric oxide synthase (NOS), macrophage migration inhibitory factor (MIF) and epidermal growth factor (EGF), are important for sperm motility, morphology and capacitation (20, 21). TTH may affect the testis and epididymis, thus reducing the semen quality, damaging the sperm membrane and nucleus, and interfering with sperm maturation.
In the present review, we aimed to give an update on the TTH–based male contraceptive methods, including the materials, devices and technologies as well as the molecular determinants of sperm quality and biochemical components in seminal plasma.
We conducted a systematic review of the literature concerning subjecting individuals to TTH, and its effect on semen quality, sperm protein and RNA biosynthesis, spermatocyte DNA, biochemical components of seminal plasma, and fertility or reproductive health (Figure 1). Regarding scrotal heating, we used comprehensive terms in order to optimize the search. Regarding the sperm quality, we selected a strategy that combined comprehensive terms and scrotal heat stress or hyperthermia in order not to omit any articles.
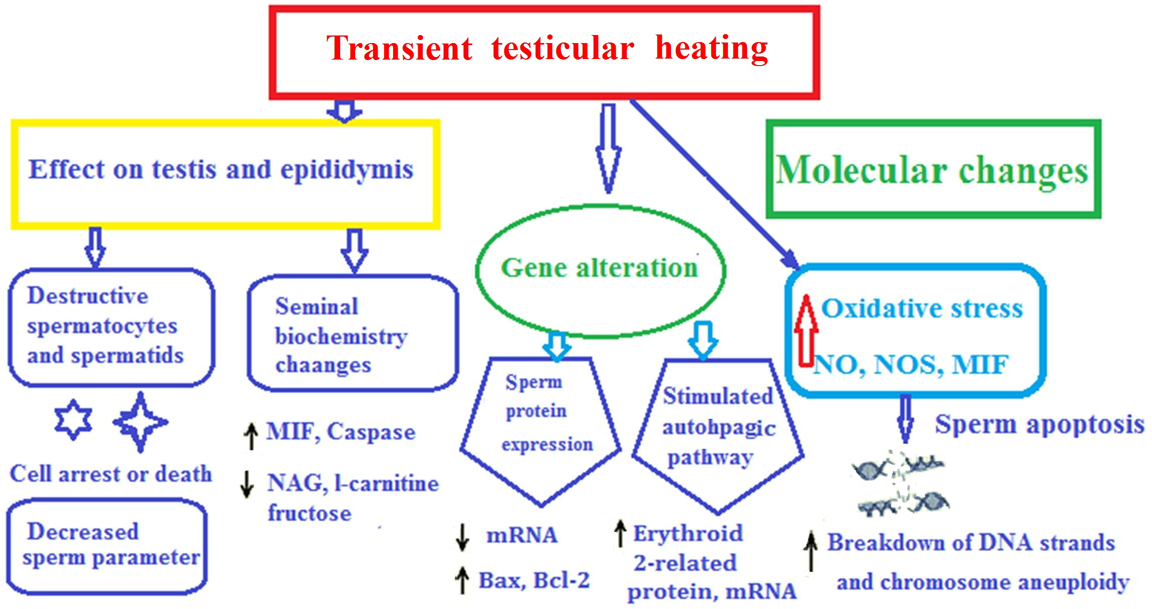
Review framework
At present, only few approaches other than condoms and vasectomy have been adequately developed for male contraception (5, 10, 23-26). Condoms and vasectomy cannot satisfy all users (27, 28). The major limitations of condoms for contraception are relatively high failure rates and interference with sexuality (29). As a quick, simple, highly effective and convenient method of permanent sterilization, vasectomy has been identified as one of the indicators of male involvement in family planning. However, the application of vasectomy has been limited due to fear of surgery and postoperative complications, such as pain, infection, sperm granuloma, epididymal and testicular damage, and its limited reversibility. Since male hormonal contraception may lead to other long-term health issues in men, it is not a preferred approach, resulting in limited experimental research and restricted clinical applications (24). A quarter of the world’s adult men are willing to share responsibility for contraception (30). Therefore, it is necessary to develop more effective and reversible methods for males in order to fulfill such greater participation by men in sharing the burdens and benefits of effective family planning. In recent years, as a potential male contraceptive method, TTH has been carried out in a large number of animal experiments and human trials, such as mice (14, 28), rats (18), rabbits (30, 31), sheep or goats (32), dogs (33), monkeys (5, 34) and humans (7, 2020, 21, 35). Each animal is anesthetized, and the lower third of the body (hind legs, tail and scrotum) is submerged in a water bath at 40°C or 43°C for 30 min (36-38). It is the most precise way to expose testes to an increased temperature for a short time by immersing the scrotum in a water bath at the desired temperature. This technique has been used in rats and monkeys (Figure 2) (18, 34). Local scrotal exposure to 42°C for approximately 1 h with a home-made electric blanket is used in boars (39). In rats, scrotal temperature is altered within the range of 13-43°C by a thermode (40). Insulated bag (with layers of nylon, cotton batting, mylar, and then canvas) covers the scrotum and inguinal region, and the temperature ranges from 34.9 ± 0.3 °C to 38.0 ± 0.1°C. In the scrotum of ram, an insulating bag is placed for 288 consecutive hours (32, 41, 42). TTH for men includes water bag and electric heating system (Figure 2). The electric warming bag or water bag can be attached to the underpants, and the temperature is controlled (20-22). For water bath, the scrotum is immersed in a water bathtub at 43°C for 10 times (30 min each time) (20-22, 35).
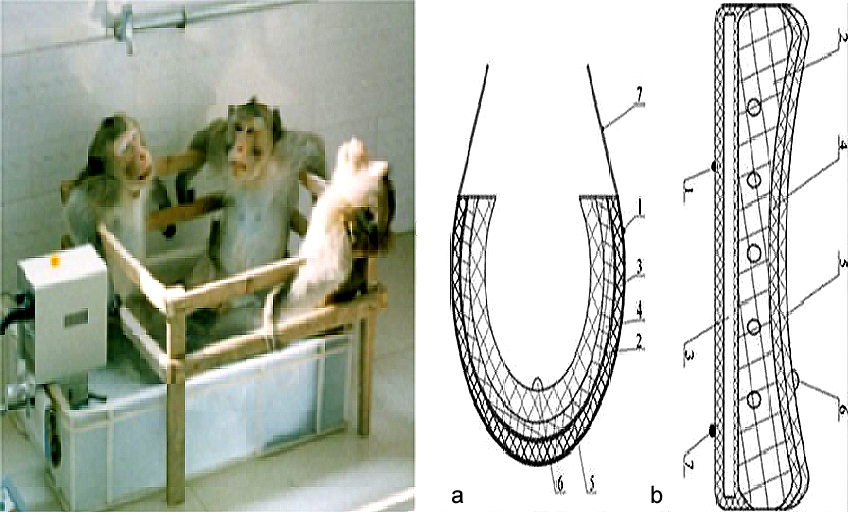
TTH for animals and humans. Left, water bath for monkeys. Right, designed sketch of scrotal heat stress device (heating underpants). No. 1 and 7 show the fixed belts, No. 2 shows the water bag, No. 3 shows the electric heating pad, No. 4 shows the protective layer of the water bag, No. 5 shows the thermal insulation layer and No. 6 shows the temperature probe which links with a digital display. (a) vertical section map of scrotal heat stress device, (b) cross-section map of scrotal heat stress device. This Figure is modified with permission from (21).
TTH can affect spermatogenesis and reduce semen quality. A large number of experiments on TTH have reported that local testicular heat treatment in water at 43ºC can induce reversible oligospermia or azoospermia in rodents and monkeys (5, 18, 34, 43-45). Short-term exposure of the testes to heat results in selective, but reversible damage to the seminiferous epithelium (18, 46). TTH may damage spermatogenesis and semen quality, leading to declined fertility of many animal models (18, 44-49). Exposure of the testes to TTH, cryptorchidism or varicocele can increase the death of germ cells. Accumulated evidence indicates that a single exposure of the rat (43 ºC for 15 min) or monkey (43 ºC for 30 min) testes to heat can result in selective and reversible damage to morphology, motility and concentration of sperm (43, 47, 50). When adult male mice are exposed to whole-body heat of 37-38°C for 8 h per day for 3 consecutive days, the sperm motility is reduced, and damage in sperm membrane is increased (51). In boars, a single heat exposure results in the decreased number of spermatozoa as well as the impaired motility and reduced proportion of normal spermatozoa (50, 52, 53). The motility and proportion of normal spermatozoa may be recovered in approximately 6 weeks after TTH in boars (53, 54, 55) and 4-8 weeks in human males (7, 20-22, 35).
TTH consequently changes the concentration, motility, morphology and membrane of sperm (Figure 3). The sperm count and motility can be affected by a sauna exposure from 37 to 45 °C (19). Ahmad et al. (56) have reported that TTH reduces the proportion of motile and viable spermatozoa and total sperm count of men. Oligozoospermic men with a varicocele have significantly higher scrotal temperatures than normozoospermic men (15). Galil et al. (57) have found that exposure of one or both testes of rats to heat at 43°C for 30 min results in a significant reduction in blood flow per testis. Testicular blood flow decreases along with testicular weight loss. Parameters of rabbit spermatozoa, such as metabolic activity and motility, are largely modified after a short exposure of spermatozoa to an environment of 42°C for only 3 h (31). Experimental TTH has also been demonstrated to severely suppress spermatogenesis in several studies on rats (58), mice (59), monkeys (60) and humans (7, 20-22, 35). Therefore, TTH may be used as the potential target of contraceptive development for men (10, 12, 61-63). Spermatogenesis and parameters of sperm concentration, morphology and motility in semen samples are adversely affected by TTH. In human, the minimum sperm concentration and total sperm count can be achieved during 6 or 8 weeks of TTH, and a good reversibility is observed in the recovery period (20, 35).
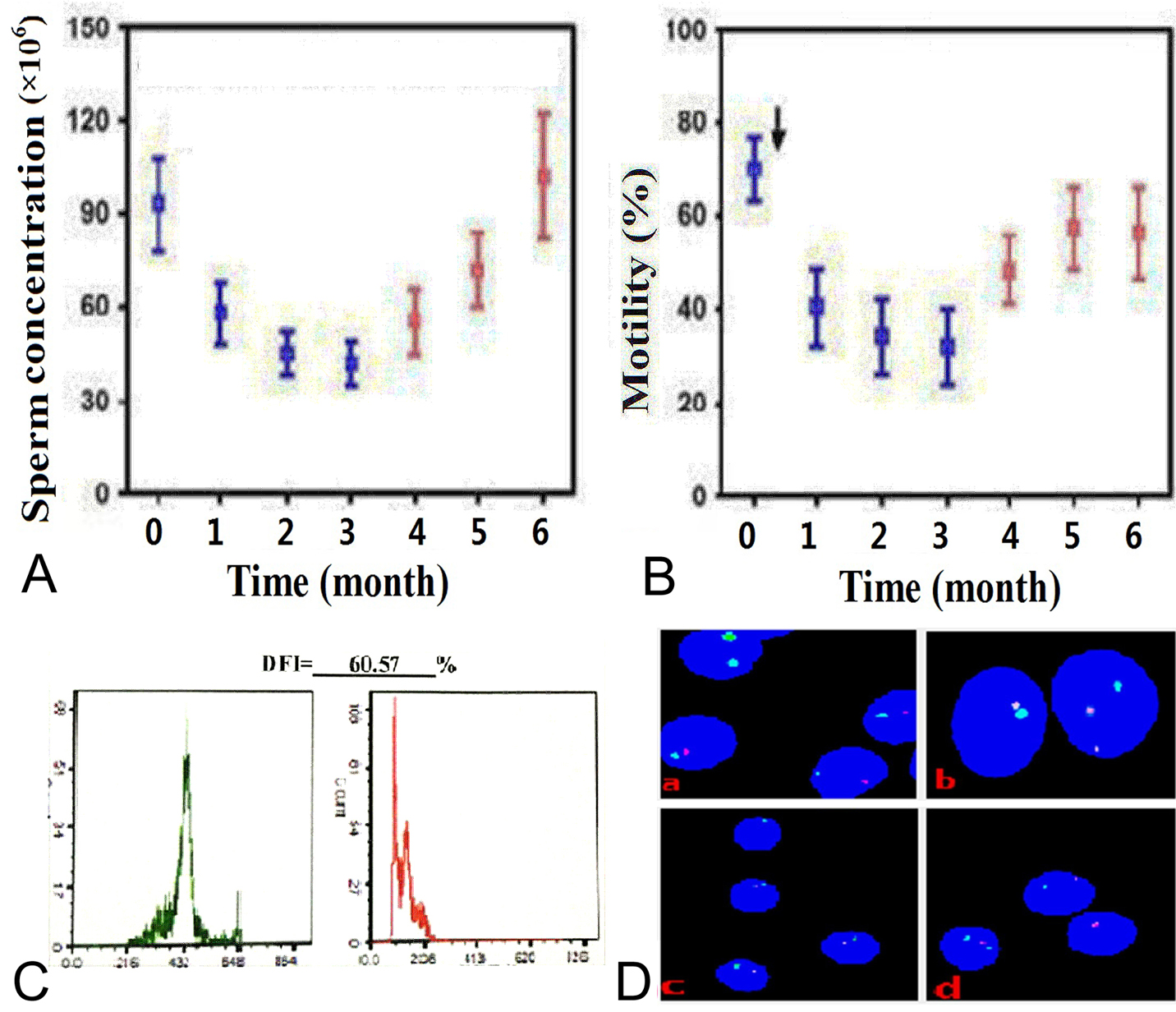
Changes in sperm parameters, DNA fragmentation and chromosomes under TTH. In addition to mice, primates such as monkey and humans are used to assess the role of TTH on spermatogenesis and male fertility. TTH leads to changes in sperm quality (e.g., sperm concentration, motility, morphology, and membrane characteristics), sperm DNA (e.g., chromosome structure, caspase activity, and acrosin), and several important biochemical substances in seminal plasma. (A) and (B) Sperm concentration (left) and motility (right) before, during and after scrotal heating. This Figure is modified with permission from (22). (C) DFI from SCSA under TTH. (D) Sperm FISH assay. (a) and (b), sperm chromosome X centromere (green), chromosome Y centromere (red) and chromosome 18 centromere (blue) specific probes. (a) Before TTH, spermatozoa with one chromosome Y (3 spermatozoa) or one chromosome X (one spermatozoon) and onechromosome 18. (b) during TTH 3 months, a spermatozoon with 2 chromosomesY and one chromosome18 (the right). (c) and (d), sperm chromosome 13 centromere (green) andchromosome 21 centromere (red) specific probes. (c) before TTH, spermatozoa with one chromosome 13 and one chromosome 21. (d) during TTH 3 months, a spermatozoon with one chromosome 13 and one chromosome 21 (the top) and a spermatozoon with two chromosomes 13 (the bottom left) and a spermatozoon with one chromosome 21.
Testicular thermoregulation is important. Shortly after heat exposure, a loss in testicular weight occurs. This reduction in testicular weight can be ascribed to loss of germ cells. Although the testicular weight may be partially regained several weeks after heat exposure in the rats, the testis remains lighter than before heat exposure (64). Spermatogenesis is temperature-sensitive, and an increase of 1°C entails a 14% drop in spermatogenesis, consequently leading to poorer sperm production (65). However, elevation of testicular temperature causes testicular germinal atrophy and spermatogenic arrest in male subfertility (66). Exposure of testes to TTH can cause severe damage in the seminiferous tubules. Selective and reversible damage to the seminiferous epithelium has been found in mouse (10), rat (12), monkey (34, 65) and human testes (20-23) after exposure to 43°C. Liu et al. (14) have reported that after a 42°C heat shock for 30 min in mice, acidophilic, coagulated germ cells are found in the seminiferous epithelium, most spermatocytes are affected, and full of abnormal cells appear in the corpus and caput of the epididymis. Degeneration, vacuolation, disorganization, multinucleated giant cells, destructive spermatocytes and spermatids, and hyperplasia of Leydig cells are observed in a large proportion of seminiferous tubules in the atrophic testes in mice (67). In addition, 7 days after an exposure to a 43°C water bath for 20 min or after a 2-h 42°C heat treatment for 8 days, low testicular weight and severe vacuolization of seminiferous tubules along with loss of spermatogenic cells are observed in mice, and appearance of multinucleated giant cells can be found (58, 68-70). Hamilton et al. (42) have reported that in rams treated with insulating bag, initial testicular degeneration as well as mild and multifocal vacuolization of SCs are observed, exhibiting a small to moderate proportion of desquamated cells containing three or more nuclei (giant cells) inside the seminiferous tubules, desquamation of germ cells and mild multifocal interstitial fibrosis. In rhesus monkeys, after TTH at 43°C for 30 min daily for 6 consecutive days, the disrupted structure of seminiferous epithelium is found on day 8 after hyperthermia with some germ cells sloughing from SCs, and most germ cells are lost from the seminiferous epithelium at days 15 and 30 after treatment (68, 71). The structure of seminiferous epithelium starts to recover between days 45 and 75, and complete recovery is found on day 90 (71). In addition, spermatogenesis and seminiferous tubule diameter are decreased after the testosterone undecanoate plus heating in fertile males (7). Among the germ cells, the types that are most vulnerable to heat are the pachytene and diplotene spermatocytes as well as the early round spermatids in humans (72). Therefore, the effects of TTH on testicular weight, seminiferous epithelium, germ cells and spermatogenesis are evident. However, these alterations can be recovered after termination of TTH. Liu et al. (14) have reported that after 1-week recovery period of TTH, new spermatocytes can be found in injured tubules which do not contain spermatid cells, and spermatogenesis and the morphology of all seminiferous epithelium can be completely recovered at 6 weeks after termination of TTH.
The integrity of sperm and oocyte DNA plays a fundamental role in the development and quality of embryos. Spermatozoa deliver DNA to the oocyte at fertilization. Sperm DNA fragmentation (sDF) is the most common DNA abnormality presenting in the infertile males (71) and TTH treatment procedures (11-13, 20-23). Single and double DNA strand breaks in the sperm nucleus are the main types of sDF. Indeed, there is evidence that sperm DNA breaks may originate in the testis and epididymis during transit in the ejaculatory ducts, and following ejaculation. Pathways of DNA breaks may be the beginning of sperm apoptosis, leading to endonuclease activation and a direct attack to DNA by free radicals which produce both base oxidation and strand breaks. The apoptotic process mainly occurs during spermatogenesis by affecting the testicular function and derailment of the chromatin condensation. The germ cell damage by TTH includes sperm apoptosis (8, 17, 18), DNA damage (73, 74) and generation of ROS (75-77). Under oxidative stress, ROS can cause severe damage to cellular components and increase sDF. TTH has been associated with an increase in testicular oxidative stress (35). Several studies have reported the mechanisms underlying the heat-induced alteration of semen parameters and loss of germ cell DNA integrity (7, 8, 11-13, 23). TTH to temperatures higher than the normal physiological temperature can change the structure of sperm chromatin, resulting in the disruption of spermatogenesis (78, 79). In several rodent models, testicular heat stress, including TTH and surgical induction of cryptorchidism, results in reduced testicular weights, germ cell loss and increased rate of sperm apoptosis (12, 80-82). Oxidative stress directly or indirectly causes sDF. Excessive levels of ROS can damage normal spermatozoa by inducing lipid peroxidation and DNA damage. Some studies have found a clear relationship between heat and oxidative stress (83, 84). Other studies on rams have reported a reduced integrity of sperm DNA under heat stress conditions induced by testicular insulation (32, 42). Oxidative stress is described as the main cause of sDF and may be related to protamine deficiency and sperm apoptosis (85). As a major cause of impaired spermatogenesis, TTH is greatly associated with oxidative stress and germ cell apoptosis (86). Early studies of TTH have found that DNA fragmentation remains high in cryptorchid mouse testis, suggesting that male infertility is likely caused by high sDF (17, 87). An abnormal increase in ROS levels can overwhelm local antioxidants and lead to oxidative stress (11, 88). Kanter et al. (38) and Park et al. (68) have reported a significantly decreased expression of proliferating cell nuclear antigen and an elevated activity of terminal deoxynucleotidyl transferase dUTP nick end labeling (TUNEL) (TUNEL–positive apoptotic cells) after TTH in rats and mice. Another study has indicated that TTH can cause azoospermia and oligozoospermia in monkeys by increasing the germ cell apoptosis (44). A previous study has reported elevated sperm mitochondrial ROS generation, increased sperm membrane fluidity and DNA damage, and an activated oxidative stress cascade in adult male mice exposed to an elevated ambient temperature of 35°C for 24 h (88). A slight increase in scrotal temperature caused by occupational exposure, lifestyle or cryptorchidism can disrupt spermatogenesis and ultimately cause problems with fertility (11, 19).
TTH may contribute to sperm chromosomal changes by reducing antioxidant power and increasing DNA strand breaks. The study by Sailer et al. (78) has suggested that scrotal exposure to 40°C in mice decreases the number of testicular haploid cells but increases the diploid germ cells, and some thermal ranges above normal allow production of sperm with compromised nuclear chromatin structure. Heat shock to spermatozoa can distort the sex chromosome (X and Y) ratio (78). The proportion of sperm aneuploidy is correlated with that of apoptotic sperm and high frequency of abnormal sperm morphology (89-91). TTH can affect sperm chromosome (22) (Figure 3) and increase in the incidence of X and Y univalents at metaphase I (92). Chao et al. (93) have reported that when mouse sperms are heated in a water bath at 50-95°C for 30 min, the aberrant chromosome rate is increased from 16.3% to 100%. TTH at 42°C results in the reduction of pregnancy rate, decreased placental weight and litter size, and abnormal embryonic development (10).
TTH can cause a rapid and transient suppression of spermatogenesis, and damage to the sperm DNA and acrosin. As an acrosomal protease, acrosin is synthesized as a pro-enzyme and activated into beta-acrosin during acrosin release. TTH may decrease acrosin activity in sperm (22, 35). Oxidative stress can be deleterious for DNA fragmentation and acrosin release. Acrosin plays a key role in the fertilizing capacity of human spermatozoa, and its activity directly affects the fertilization rate (94, 95). Several human studies have found that poor semen quality of infertile patients is related to a high scrotal temperature, and thermoregulatory failure-triggered heat stress can damage acrosin and increase the risk of infertility (96-98). Acrosin may act as a matrix-degrading proteinase and play an important role in sperm penetration of the zona pellucida (99, 100). In many cell types, hypoxia and oxidative stress trigger apoptosis and cell death. Heat stress may induce oxidative damage to sperm plasma membrane, sperm parameters, germ cells, the cell division of SCs, and acrosin activity (20, 101-103).
Hyperthermia has detrimental effects on the testis, including DNA damage in germ cells and mature sperms, poor-quality spermatozoa, and a lengthy recovery period of the testis from TTH (10, 12). Some studies have provided evidence that the mitochondria-dependent apoptotic pathway is involved in the activation of the initiator caspase-9 and executioner caspase-3 in heat-induced germ cell death (51, 104-106). During apoptosis, caspase-3 cleaves the inhibitor, thereby allowing the nuclease to cut the chromatin. Caspase-3 is activated by cleavage of its proenzyme (32 kDa) to yield two active subunits p18 and p12, and the activation of caspase-3 is used as an important index of apoptosis (104, 105). In fertile males, during TTH treatment, the sperm motility, number and normal morphology are decreased, whereas the rates of sDF, abnormal chromatin condensation and the caspase-3 activity are increased, and these indicators are restored to the normal levels before the TTH in recovery period (20-22). The testis displays severe damage, and the number of cleaved caspase-3-positive germ cells per tubule is dramatically increased when mice are exposed to a single TTH treatment (42°C for 25 min) (10, 105). Wang et al. (105) have reported that after TTH (40°C for 2 h), the enzyme activities of caspase 3, 8 and 9 in newt testis are significantly elevated. In non-apoptotic cells, caspase-activated deoxyribonuclease is present as an inactive complex with inhibitor of caspase-activated deoxyribonuclease. Following a single mild TTH exposure (40°C or 42°C for 30 min), an increased expression of cleaved caspase-3 and a decreased expression of inhibitor of caspase-activated deoxyribonuclease are found in mouse testis. The reduced expression of inhibitor of caspase-activated deoxyribonuclease contributes to the increased activity of caspase-activated deoxyribonuclease, and this finding is consistent with the increased rate of DNA fragmentation (19). When 8-week-old mice are exposed to a single TTH (42°C for 25 min), the testes display severe damage with multinucleated giant cells, and the number of cleaved caspase-3-positive germ cells per tubule is dramatically increased (10, 12). After TTH (42 °C, 30 min) in male Balb/c mice, the number of TUNEL-positive cells (apoptotic index of spermatogenic cells) and expressions of caspase 3, 8, 9 are increased, whereas the expression of Bcl-2 AKT at the mRNA level is decreased (105). When adult mice are exposed to 37-38°C for 8 h, considerably more caspase-3-positive germ cells are found in heat-treated mice at 16 h after heat exposure compared with the controls left at 23-24°C (51). In mice treated with TTH at 43 °C for five cycles, the levels of cleaved caspase-3 and TUNEL-positive apoptotic spermatocytes are increased (12, 106, 107). However, histological examination of excised testes and epididymides for apoptotic (TUNEL and activated caspase-3) and proliferating cells (Ki-67 antigen) indicates only marginal effects of scrotal insulation on tissue morphology in adult beagle dogs (insulated with a self-made scrotal support for 48 h, 9 weeks later). It suggests that a mild TTH in dogs does not cause substantial changes in sperm quantity and quality, and canine testes and epididymides may have a higher competence to compensate such thermal stress (33).
Previous studies have reported the changes in sperm parameters (Figure 3), sperm apoptosis, and molecular mechanisms of TTH exposure (18, 31, 34, 108, 109). The protein expression and autophagy of heat-induced spermatogenic impairment play important and complex roles in maintaining a series of cellular functions. Within the testis, spermatogenesis is a strictly regulated, protracted and complex process, and it must, in part, be dictated by networks of many genes (110). These processes are particularly vulnerable to perturbation by genetic and environmental factors.
During the sperm development, correct timing of gene expression plays a fundamental role within biological processes. Gene expression during spermatogenesis is highly orchestrated and strictly regulated at the transcriptional and posttranscriptional levels, and the basis of male germ cell differentiation and male fertility depends on the correct gene expression patterns (111). Serial analysis of gene expression and microarray profiling of rodent testis samples and enriched cell populations have identified a large number of important genes for spermatogenesis (111-113). Spermatogenesis related factor-2 is one of the cloned genes involved in division and differentiation of germ cells (112). The occludin expression at the protein and mRNA levels is decreased in boars under local scrotal exposure to 42°C (39). Chihara et al. (109) have found that heat-induced testicular damage through the MRL/MpJ-derived locus on Chr 1 may play a pivotal role in the recovery from heat-induced testicular damage, especially via the inhibition of calcification. The microRNAs (miRNAs) are small, non-coding RNAs with approximately 17-25 nucleotides in length, which act as potent posttranscriptional regulators of gene expression, and they are involved in a variety of biological processes, including cell proliferation, apoptosis and tumorigenesis. Recent studies have reported the role of miRNAs in heat stress-induced spermatogenic impairment. TTH at 43 °C for 30 min down-regulates the relative expression of cold-inducible RNA binding protein at the mRNA level but up-regulates the expressions of transforming growth factor β2 and transforming growth factor β3 in mice (114), and some identified miRNAs, including miR-449a-3p, miR-92a-1-5p, miR-423-3p and miR-128-3p, have been closely correlated with apoptosis of germ cells (115). Heat shock proteins (HSPs) can protect cells from harmful stress and regulate cell apoptosis (8). Most proteins in spermatozoa are formed before spermatid DNA condensation, and mature spermatozoa exhibit almost no protein synthesis. Therefore, the alteration of protein levels in spermatozoa can largely reflect the effect of hyperthermia on spermatogenesis, not only on spermatozoa itself. These proteins modulate the correct folding of stress-induced misfolded proteins and protect germ cells and spermatozoa from apoptosis (8, 19). A sufficient increase in blood flow may not support an increased metabolism in the testes after heat stress, and the germ cells may be under relatively hypoxic conditions (12). Zhang et al. (73) have demonstrated that besides initiating apoptotic pathways, heat also induces autophagic pathways in germ cells. The expression of autophagy-related gene 7 is positively correlated with the level of autophagy in germ cells. TTH induces lipid droplet accumulation and up-regulates the expression of adipose differentiation-related protein (14). The expressions of Bax and Bcl-2 at the protein and mRNA levels can be increased after local testicular heating (42°C, 1 h) in boar testis (116). TTH can cause the apoptosis of germ cells by regulating the expressions of Bax and Bcl-2 at the protein level. In the mouse SC line, the expression of RNA binding motif protein 3 is increased or decreased within 12 h after temperature shift from 37 °C to 32 °C or 39 °C, respectively (117).
As a survival, lysosomal degradation and tightly regulated pathway for the clearance and recycling of damaged cytoplasmic organelles or cellular components, autophagy plays fundamental roles in cellular homeostasis (118-122). ROS can induce various cellular responses, including apoptosis, programmed necrosis and autophagy, depending on the cellular setting. During the response to sublethal stress and autophagy, cells undergo rapid changes to adapt their metabolism and protect themselves against potential damage. This is orchestrated through a multifaceted cellular program and evolutionarily conserved physiological process, and it can serve as a cell survival pathway, suppressing apoptosis (120-123). Autophagy has been shown to engage in a complex interplay with apoptosis and to promote cell survival because nucleotides, amino acids and free fatty acids can be generated during the degradation of cellular contents and then recycled and reused for macromolecular synthesis and ATP generation (73, 123). In the testis, autophagic pathway can be stimulated by multiple forms of cellular stress, including nutrient or growth factor deprivation, hypoxia, ROS, DNA damage, protein aggregates, chemical insults, withdrawal of gonadotropin or testosterone, heat, radiation, damaged organelles, or intracellular pathogens (69, 73, 123). Hyperthermia causes germ cell death by oxidative stress in testes, triggering antioxidant signals, including autophagy and nuclear factor erythroid 2-related factor 2. Under a 2-h heat treatment at 42°C for 8 days, heat exposure increases the expression of erythroid 2-related factor 2 at the protein and mRNA levels in male mice, and the testes may be protected by the autophagy inducer (69). Varicocele is in chronic state of heat stress and can lead to impaired spermatogenesis by apoptotic and autophagic pathways. Moreover, apoptotic markers (active caspases 3/7 and DNA fragmentation) and autophagic markers (autophagy-related gene 7 and light chain 3 proteins) are significantly up-regulated in infertile men with varicocele compared with fertile individuals (124). In heat-treated (water bath at 42°C for 15 min) mice, besides initiating apoptotic pathways, autophagic pathways are also induced in germ cells, and TTH results in several specific features of the autophagic process, including autophagosome formation and the conversion of light chain 3-I to light chain 3-II (73). Recently, Chen et al. (125) have reported that supplementation of methylmercury may induce germ cell apoptosis of rats through oxidative stress and autophagy. Horibe et al. (119) have observed high levels of autophagy in stages VII–VIII of the spermatogenic cycle, and autophagy is specifically up-regulated in SCs of adult rats under stress of ethanol treatment. The up-regulation of autophagy is a specific stage, and it may be related to androgen receptor suppression, mitochondrial damage, lipid accumulation, and phagocytosis of apoptotic cells. Autophagy and endocytosis can efficiently transport transcription factors, adhesion molecules, or secreted factors. Mitochondrial-ER contact sites are important in autophagosome formation and have been recently recognized as a major mechanism in the regulation of self-renewal and differentiation of stem cells as well as a regulator of cell migration (119). Scrotal insulation in boars can damage meiotic germ cells due to changes in the integrity of the blood-testis barrier (BTB), which induce apoptosis, autophagy and DNA damage in the germ cells (126).
Zhu et al. have reported the cellular and molecular mechanisms of TTH in murine testis (127), showing that 108 proteins are differentially expressed between the heat shock tissues and the control mouse testes, and 36 proteins are identified by comparing with the control reference map. Some of the proteins may be early molecular targets responsible for suppression of spermatogenesis induced by TTH in rhesus monkeys and humans (127). Another study has also investigated the molecular mechanisms underlying the heat-induced suppression of spermatogenesis in human testis, and found 32 and 26 differentially expressed proteins at week 2 and week 9 after TTH treatment, respectively (128). Heterogeneous nuclear ribonucleoprotein H1 is an anti-apoptotic protein that can regulate the expressions of other heat-induced proteins (128). After TTH, mice exhibit an increased expression of hypoxia-inducible factor 1 alpha (Hif1α) at the mRNA level and a translocation of Hif1α protein to the germ cell nucleus (12). TTH alters the expression levels of various genes in a complex manner, accompanied with post-translational modification and protein localization, and altered gene expressions will lead to changes in the protein composition of spermatocytes. However, another study has found an increased Hif1α expression at the mRNA level in spermatozoa after sauna exposure (19). Hif1α is a transcription factor, its target gene is involved in vasodilation, angiogenesis and glycolysis, and it can induce the expression of vascular endothelial growth factor. Hikim et al. (129) have found that the initiation of apoptosis in wild-type mice is preceded by a redistribution of Bax from a cytoplasmic to paranuclear localization in heat-susceptible germ cells. The relocation of Bax is accompanied by sequestration of ultra-condensed mitochondria into paranuclear areas of apoptotic germ cells, and cytosolic translocation of mitochondrial cytochrome C and Bax is associated with activation of the initiator caspase-9 and the executioner caspase-3 (129).
Sertoli-Sertoli and Sertoli-germ cells are the specialized junctions at contact sites of seminiferous epithelium, and they play pivotal roles in spermatogenesis. Cai et al. (102) have reported that after a TSH (43°C for 30 min) treatment for 24-48 h, the expressions of tight junction components of occludin, claudin-3 and zonula occludens-1 (ZO-1) are decreased, whereas the claudin-11 expression at the mRNA level is increased, and the protein localization of occludin and ZO-1 is lost from the BTB site. A transient induction of transforming growth factor-β2, -3 and p38 mitogen-activated protein kinase activation may lead to the reversible BTB disruption by TTH. In humans, HSPs (such as HSP70 and HSF1) and mitochondria functions are up-regulated, and Bcl-2, mitofusin-2 and spermatozoa-associated antigen 6 are down-regulated in TTH treatment (130). Chen et al. (34) have reported that the expression of androgen receptor in primary monkey SCs is dramatically decreased during TTH. The expressions of adherens junction-associated molecules of N-cadherin and beta-catenin, and tight junction-associated molecule ZO-1 are decreased in 24-48 h by TTH treatment, and the intermediate filament vimentin is up-regulated in 6-48 h. The expressions of androgen receptor and Wilms’ tumor gene 1 are dramatically decreased during TTH. Both proteins completely disappear immediately after termination of TTH treatment and begin to recover after 6 h (34). The androgen receptor plays a crucial role in the heat-induced reversible change in BTB via the partitioning-defective protein polarity complex, which may be the mechanism of TTH underlying a reversible disruption of the BTB (59). When the androgen receptor is over-expressed in SCs using an adenovirus, the heat stress-induced down-regulation of BTB-associated proteins of ZO-1, N-cadherin, E-cadherin, α-catenin and β-catenin is partially rescued. Ablation of androgen receptor by RNAi or flutamide treatment (an androgen receptor antagonist) in SCs can inhibit the recovery of BTB-associated protein expression after TTH treatment of 43°C. The recovery of BTB permeability induced by temporal heat stress may be regulated by the androgen receptor in mice (59). TTH can change the expressions of some protein components in the spliceosome and result in a reversible dysfunction of RNA splicing in its target pre-mRNAs in animal and human males.
As stress-responsive proteins, HSPs are the most prominent group of proteins involved in folding and unfolding of other proteins. The most important event in stressed cells is the synthesis of a highly conserved family of proteins, such as HSPs. HSPs play an important role in the homeostasis, apoptosis regulation and the maintenance of various physiological processes. HSPs are considered to be essential factors in animal reproduction and required for spermatogenesis, and they also protect cells from environmental hazards, such as heat, radiation, and chemicals (131, 132). The expressions of HSPs are regulated by heat shock transcription factors (HSFs) for their cytoprotective functions during cellular stress. Proper spermatogenesis can be maintained by cooperation of different HSFs. For example, knockout cooperation of HSF1 and HSF2 results in meiosis arrest and spermatocyte apoptosis (131, 132). Elevated testicular temperatures can induce activation of HSF1. Activation of HSF1 by heat shock initiates apoptosis of spermatogenic cells, leading to infertility of males. In the male germ cells (spermatocytes), caspase-3-dependent apoptosis is induced upon HSF1 activation, and spermatogenic cells are actively eliminated (133, 134). In addition to the up-regulation of HSF1, which in turn increases HSP production, other up-regulated genes include those responsible for apoptosis, cell adhesion and signal transduction during TTH. Rockett et al. (135) have used DNA microarrays to interrogate the expressions of 2, 208 genes and thousands more expressed sequence tags (ESTs) in mouse testis. Of these genes, 27 are up-regulated and 151 are down-regulated after TTH (135). TTH can reduce the expressions of antioxidant-related enzymes, spermatogenesis-related proteins and sex hormone receptors (androgen receptor, luteinizing hormone receptor and follicle-stimulating hormone receptor) in rats (136). Heat increases oxidative stress, affects mitochondria and results in DNA damage, eventually leading to spermatozoal apoptosis. The expressions of steroidogenic acute regulatory protein and HspB10 genes are decreased by long-term heat stress via the expressions of steroidogenic pathway and small HSPs in rat testis at 38°C for 9 weeks (137). TTH may adversely induce effects on sperm parameters and gene expression. TTH has effects on expressions of Bax, Bcl-2 and sperm parameters in male mice. Bcl-2 family members may induce sperm apoptosis under heat stress (138). Fas/FasL is another important cell death pathway. When Fas/FasL is activated and caspase-3 is cleaved, the cell apoptotic rate is decreased (139).
Spermatozoa are produced in the testis, which acquire the maturation ability in the epididymis. The caput and corpus of the epididymis as well as the epididymal fluids play a critical role in sperm maturation (140, 141). During the epididymal transit, spermatozoa undergo critical changes in structure and function. Some of biochemical substances and proteins in epididymal fluid, such as fructose, NAG, L-carnitine, MIF, EGF and dipeptidyl HSP, are the key factors for maturation and function of epididymal sperm (22, 23, 142-146).
Numerous studies have investigated the effect of TTH on spermatogenesis and sperm maturation (143, 144, 146). In epididymis, strong staining signal of HSP10 is detected in pseudostratified columnar epithelium (144). A total of 270 proteins may participate in epididymal sperm maturation, and 34 epididymal milieu proteins contribute to the composition of seminal fluid proteomes (146). Wang et al. (143) have identified the key heat stress-associated sperm maturation proteins in the rat caput epididymal fluids responsible for sperm maturation, and demonstrated 21 proteins corresponding to 29 differential protein spots, including 10 down-regulated and 11 up-regulated proteins in heat-treated rats. In ram epididymis, 17 seminal plasma proteins are affected by treatment, actin, albumin, HSP 70 kDa, protein DJ-1 and so on in seminal plasma are up-regulated, and some of proteins, such as dipeptidyl peptidase 3 and isoforms of HSP 90 kDa, are down-regulated after scrotal insulation. Expressions of most such proteins return to normal levels after scrotal insulation for 113 days (146). Changes in seminal plasma proteome by heat challenge may be correlated with variations in semen parameters, such as sperm protection, maturation and fertilization.
TSH can affect epididymal spermatozoa. The TTH-treated epididymal sperm has lower sperm-zona pellucida binding and oocyte penetrating capacity. These effects are first seen at 1 week, and then they become more prominent at 2 weeks after heat exposure (147). In another study, mice exposed to whole-body heat show lower motility and membrane changes of sperm obtained from the cauda epididymis (148). In a previous study, when TTH is applied to male mice at 42°C for 30 min, the sperm count, motility and viability are reduced, and a sex ratio distortion occurs in epididymal spermatozoa (149).
When the TTH causes sperm apoptosis and changes in the testis, the epididymal microenvironment is also destroyed in a short time, thereby altering the contents of semen biochemical substances (20). ROS are metabolites of NO, playing a role in many physiological functions of sperm. NO is a free radical produced by most cells, including human male and female reproductive tracts, and it is an important regulator in many physiologic processes and a well-known oxidative stress agent that directly inhibits mitochondrial respiration and DNA synthesis. Shiraishi and Naito (150) and Carreira et al. (151) have both reported that NO is synthesized through the enzymatic conversion of L-arginine to L-citrulline by NOS. Many previous studies have shown that mature human spermatozoa synthesize NO by up-regulating NOS which exists in at least three distinct isoforms, neural NOS (nNOS), endothelial NOS (eNOS) and inducible NOS (iNOS). Three distinct isoforms of NOS are localized in the sperm head and midpiece (152, 153). Cellular injury and apoptosis occur in various cell systems through the up-regulation of iNOS to increase NO synthesis (154-157). It has been reported that various physiologic activities via different signaling pathways, including the cyclic guanosine monophosphate (cGMP)-protein kinase G and mitogen-activated protein kinase pathway, can be regulated by lower concentrations of NOS (158, 159). At low concentrations, NO stimulates guanylate cyclase activity and triggers the formation of cyclic guanosine monophosphate. Higher concentrations of NO produced by iNOS interact with thiol groups (158). At higher concentrations of NOS, the molecule can promote the germ cell apoptosis through peroxynitrite, which is generated by a reaction between NO and superoxide (160). High concentrations of NO have injurious effects on sperm properties, such as motility, morphology, and DNA stability, which can increase apoptosis and block all sperm functions (161-163). Intracellular NO, a free radical, is a factor that physiologically regulates spermatozoa function and pathophysiology of male reproductive system (164, 165). Previous study has well documented the role of NO in multiple signal transduction pathways of male germ cells, such as capacitation and acrosome reaction (AR), and activation of apoptosis signaling is negatively correlated with NO production (166). The signaling for iNOS expression may be similar to that induced by cellular injury and apoptosis. This concept is supported by the evidence that a significant loss of germ cells is caused by up‐regulation of testicular iNOS and the seminiferous epithelium damage after treatment with endotoxin lipopolysaccharides (167-169). iNOS may play a functional role in spermatogenesis via apoptosis, leading to reduced sperm count in monkeys (170). Low concentrations of NO improve the motility of mouse (171), hamster (172) and human (173, 174) spermatozoa, as well as the zona pellucida-binding ability of human spermatozoa. Maturity of human spermatozoa has been correlated with active NO production by NOS and inactivated apoptosis signaling, indicating a rather anti-apoptotic effect of NO. NO may be enough to affect the fecundity potential in vivo (171, 175). A previous study by our group (21) has shown that the levels of NO and NOS in semen are negatively correlated with sperm concentration, motile and progressive motile sperm and normal morphological sperm, but positively correlated with sDF and caspase-3 activity during TTH in human.
NO, NOS and MIF are important regulators in many physiologic processes (21, 22). MIF is a well-known pro-inflammatory mediator with important functions in human reproduction and prostatic physiology. As a T-cell cytokine, MIF is present in large quantities in human semen and it is transferred to spermatozoa during the epididymal transit, which has been correlated with sperm maturation and stability (176-178). A higher level of MIF is found in epididymis and associated with spermatozoa, prostasomes as well as the soluble fraction (179). Eickhoff et al. (180) have suggested that MIF plays an important role in the maturation process of rat sperm during epididymal transit. Some studies have indicated that MIF is secreted by the Leydig cells of the testis, and it is a constituent of seminal fluid (177, 181, 182). Interestingly, the expression of sperm-associated MIF has been negatively correlated with the proportion of motility in different semen samples (183). Komori et al. (184) have reported that MIF in seminal plasma is higher in patients with abnormal AR. Therefore, MIF in seminal plasma may negatively affect sperm function. Our group has reported (21, 22) that the MIF level is negatively correlated with conventional sperm parameters and NAG in seminal plasma but positively correlated with sDF index.
Apart from proteins, seminal plasma also contains several small molecular substances, which are shown to play active role in sperm maturation/protection processes. Seminal NAG, L-carnitine and fructose are regarded as the reliable biochemical parameters to examine epididymal patency, ejaculatory duct obstruction, and prostate and seminal vesicle function or its hypoplasia (184-188). They are used for the diagnosis of sperm function and obstructive azoospermia.
Fructose in seminal plasma is synthesized and secreted by the seminal vesicles and accessory sex glands. It is the major carbohydrate source in seminal plasma and essential for normal sperm motility (189, 190). Rao et al. (35) have reported that fructose and zinc concentrations in TTH procedures show no significant differences compared with their baseline levels. Such finding may be explained by that during TTH, only the scrotum is immersed into the warm water, therefore the seminal vesicles are not affected by TTH.
Epididymis is the main source of NAG in semen. The neutral form of alpha-glucosidase is the main active ingredient. NAG is correlated with the location of epididymal obstruction in men with azoospermia, which helps to locate obstructive positions (22, 130, 186, 191). The NAG level remains very low in seminal plasma of epididymal duct obstruction or ejaculatory duct occlusion. NAG is considered as the best index of epididymal function, and it is correlated with human sperm concentration and motility (184, 192). In infertile oligoasthenozoospermic men with varicocele, the seminal alpha-1, 4-glucosidase activity is correlated with sperm count, sperm motility and serum testosterone (193). Varicocele causes a reduction in NAG activity by the epididymis, and the NAG level is correlated with the quality of the sperm membrane and nucleus (194). Watanabe et al. (195) have suggested that sDF index is correlated with neutral NAG activity, and the determination of NAG activity is a valuable tool in the diagnosis and would also aid in the prognosis.
Spermatozoa undergo post-gonadal modifications in the epididymis to acquire fertilizing ability. In epididymal plasma, high-molecular-weight proteins and small molecules, such as free-L-carnitine, convert the gametes into functional cells (196). As a small polar molecule present in high concentrations in mammalian seminal plasma, L-carnitine plays roles (only L-isomer) in acyl chain translocation and regulation of β-oxidation of fatty acid chains in mitochondria (197). More than 94% of the free carnitine originates from the epididymis, and free carnitine does not originate from seminal vesicles, but rather flows into the seminal fluid via the ductus deferens (198). The L-carnitine level in seminal plasma plays an essential role in maintaining male fertility, and it is correlated with sperm count, total motility and normal morphology, which can be taken as a biochemical index for the clinical treatment of male infertility as well as for the study on the mechanisms of male reproduction (198, 199). L-carnitine has effective superoxide anion radical scavenging, hydrogen peroxide scavenging, total reducing power and metal chelating activities on ferrous ions (200). Carnitine is effective in infertility treatment, and it improves male reproductive capacity in adulthood (201).
Our group (22, 142) has reported that during TTH, the NAG activity and L-carnitine level in the semen are decreased, whereas the levels of MIF and DNA fragmentation are increased. These findings indicate that TTH may affect the testis and epididymis, thereby reducing the seminal quality measures and impairing the quality of the sperm membrane and nucleus. An experimental trial consisting of 20 male volunteers reveals no significant variation in the three biochemical markers, seminal NAG, fructose and zinc concentration, before and after TTH (35). This finding may be attributed to that the functions of epididymis and accessory sex gland are not severely affected by TTH, or the number of the subjects in the clinical trial is small. Seminal plasma zinc, fructose and NAG are the functional indexes of prostate, seminal vesicle and epididymis, respectively. During TTH, prostate and seminal vesicles are farther away from heating bag or warm bath than testes and epididymis. The heat has less influence on the first two organs. Therefore, their functions are less affected.
EGF is a low-molecular-weight polypeptide that is mainly produced by submandibular and parotid glands (142, 202). It is known that EGF affects both male and female reproduction and may play some roles in testicular steroidogenesis (199). Some authors have observed that EGF has either no effect or an inhibitory/negative effect on the human sperm cell capacitation and/or AR, especially at higher concentrations (203, 204). In the semen, EGF is mainly produced by prostate (205, 206). Zhang et al. (142) have suggested that the content of seminal EGF is not changed in the TTH process, and it may be because TSH for men is placed to the scrotum and not to the prostate. Therefore, it is necessary to assess changes in EGF in semen upon TTH treatment in future studies.
As a male contraceptive method, TTH has potential advantages and feasibility. Obvious changes are observed in testis, epididymis, sperm DNA, chromosomes, mRNA and protein expression, autophagy, oxidative stress and apoptosis of germ cells, and seminal plasma biochemistry after TTH. Sperm concentration, motility, normal morphology and acrosin activity during TTH are decreased, whereas sDF and chromosomal aneuploidy are increased. TTH is correlated with the generation of oxidative stress and ROS. The rate of sperm chromosomal aneuploidy is correlated with sperm concentration, motility, morphology, membrane, and DNA integrity, caspase-3 and acrosin activities. The alteration of sperm DNA, chromosomes, mRNA and protein expression, autophagy, oxidative stress, apoptosis and molecular biology after TTH may be caused by increased levels of NO, NOS and MIF or reduced levels of NAG and L-carnitin in seminal plasma. Given that TTH is a reversible process, and further studies are needed to design contraceptive methods based on TTH.
Zhen-Ya Fang, Wei Xiao and Su-Ren Chen contributed equally to this work. This study was approved by the national “12th Five-year Plan” of Science and Technology Support (No: 2012BAI31B08). The authors would like to thank Doctor Zhi-Da Shi for their help in English language of this paper.