- Academic Editors
Despite significant progress in the field of therapy and management, chronic heart failure (CHF) still remains one of the most common causes of morbidity and mortality, especially among the elderly in Western countries. In particular, frequent episodes of decompensation and, consequently, repeated hospitalizations represent an unsustainable burden for national health systems and the cause of worsening quality of life. CHF is more prevalent in elderly women, who often have “peculiar” clinical characteristics and a more preserved ejection fraction caused by endothelial dysfunction and micro-vessel damage. At the moment, noninvasive technologies that are able to remotely monitor these patients are not widely available yet, and clinical trials are underway to evaluate invasive remote sensors. Unfortunately, implantable devices for identifying decompensation are not the most practical solution in the majority of of patients with chronic heart failure. In particular, they are hypothesized to have the possibility of monitoring patients by pro-B-type natriuretic peptide, ventricular repolarization variability, and bioimpedance cardiography at the first point of care, but new technology and clinical trials must be planned to address the development and spread of these emergent possibilities.
Chronic heart failure (CHF) is becoming a real social emergency both in Western
countries and in those with emerging economies. In fact, with the progressive
increase in population aging, the prevalence of CHF in elderly patients is higher
than 10% [1]. It is interesting to emphasize in the COVID-19 pandemic era that
re-acutizations in CHF are frequent and the deadliest comorbidities [2]. Although
enormous progress has been obtained in CHF treatments in the last two decades,
and despite optimal medical therapy or the use of the most modern implantable
devices, decompensated CHF is a critical health system issue. For example, the
in-hospital mortality rate is about 4%, and after discharge, mortality tends to
worsen; in fact, the 30-day, 1-year and 5-year mortality rates
are 10%, 22%, and 42%, respectively [3]. To stratify the mortality and mobility risks, the
research is currently focused on new, simple, noninvasive, easily repeatable, and
inexpensive markers of the decompensation of CHF, but above all, it is necessary
to validate them in homogenous groups of CHF patients. To obtain these data,
recently, the European Heart Society [4], following the indications of other
scientific societies [5], redefined this syndrome, historically based on systolic
function. From this point of view, it is possible to distinguish three
pathophysiologic, hemodynamic conditions with different systolic functions based
on the left ventricular ejection fraction (LVEF): symptomatic subjects with heart
failure with heart failure with reduced ejection fraction (HFrEF), those with heart failure with mildly reduced ejection fraction
(HFmrEF), and finally, symptomatic subjects with heart failure preserved ejection fraction
(HFpEF). The ejection fractions are
This redefinition aims to obtain more homogeneous data to improve
the risk stratification of mortality and morbidity and to promote comparable
therapeutic clinical trials able to induce an increased knowledge in this field.
In fact, almost all clinical trials have focused on treatment for patients with
systolic severe dysfunction, especially even with worse LVEF
Previously, four stages to classify the CHF was proposed as stages A, B, C, and D, from risk of heart failure (HF) (stage A), to advanced HF (stage D) [6].
Before this classification into four stages, the functional class of the New York Heart Association (NYHA) was introduced, based on the severity of symptoms [7].
Therefore, the aim of this review is to evaluate noninvasive markers that are able to identify early possible re-exacerbations in patients with advanced CHF.
As is known, CHF is a progressive disease characterized by a close pathophysiologic relationship between systolic and diastolic dysfunction because of the increase in the left ventricular end-diastolic pressure (LVEDP), also known as filling pressure. In HFrEF or HFmrEF, the increase in LVEDP originates mostly after an acute myocardial infarction or, less often, from other cardiomyopathies. On the other hand, the HFpEF is a direct consequence of left ventricular hypertrophy most frequently due to systemic hypertension or hypertrophic cardiomyopathy. In HFpEF patients, the left ventricular end-diastolic volume (LVEDV) can be normal; conversely, among HFrEF and HFmrEF patients, it is mildly or severely increased. The major consequences of an increase in LVEDP are atrial enlargement, pulmonary congestion, renin–angiotensin–aldosterone system imbalance and sympathetic activation. As result, fluid retention with edema, sinus tachycardia or atrial and ventricular arrhythmias (palpitations), and dyspnea (Fig. 1) could occur. Then, dyspnea and edema of the lower extremities are the direct consequences of pulmonary congestion and fluid retention, respectively; typical consequences of reduced systolic function and peripheral hypoperfusion are fatigue, weakness (asthenia), lightheadedness, and nocturia.
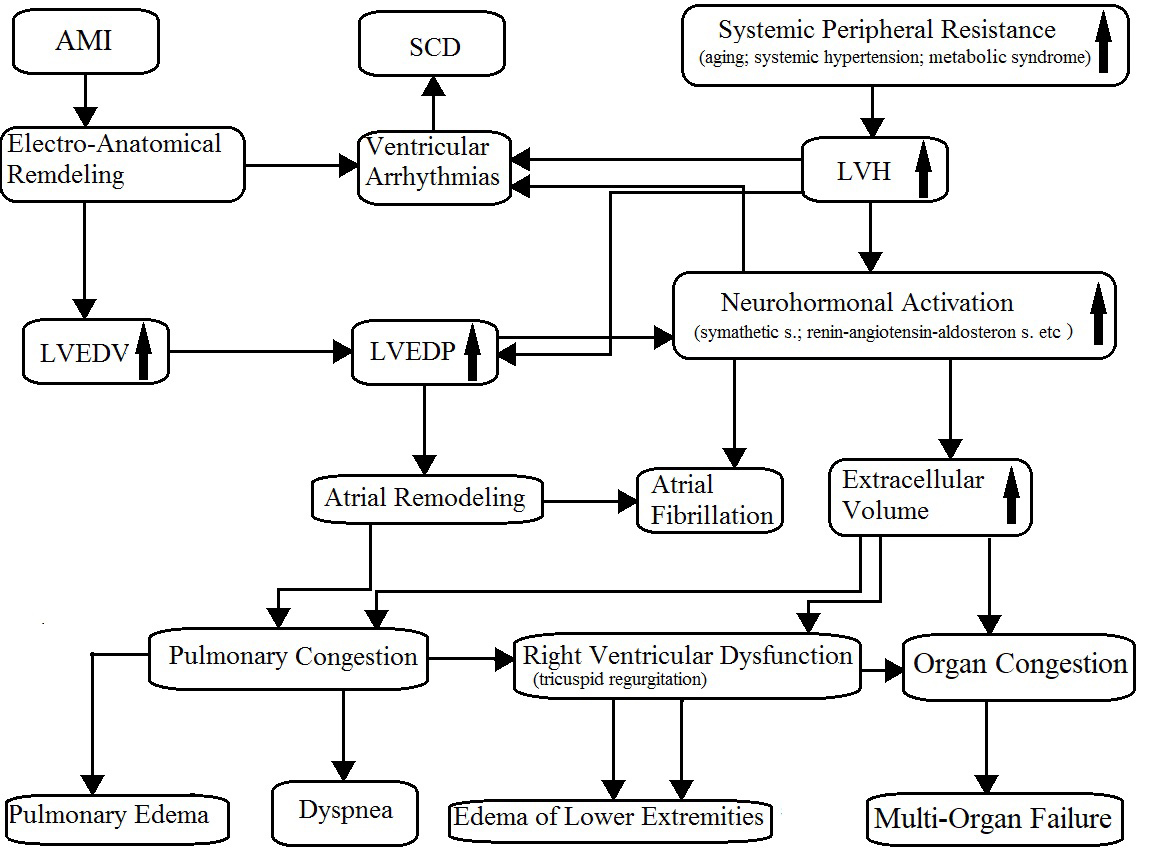
Pathophysiology of chronic heart failure. AMI, acute myocardial infarction; SCD, sudden cardiac death; LVH, left ventricular hypertrophy; LVEDV, left ventricular end-diastolic volume; LVEDP, left ventricular end-diastolic pressure. The arrows mean “increase”.
Notably, it should be emphasized that some authors have demonstrated that pulmonary artery wedge pressure (PAWP) is more closely related to outcome in HFpEF than LVEDP [8]. In the study by [8], the authors observed that the carbon monoxide-diffusing capacity (DLCO) was the only parameter that was independently correlated to the pressure difference between the PAWP and the LVEDP, concluding that both low DLCO and the pressure gradient between the PAWP and the LVEDP reflect thickening of the alveolar–capillary membrane due to chronic congestion. Therefore, according to the authors, both parameters are associated with the disease severity and should be addressed in future large-scale studies [8].
In this pathological situation, sympathetic overstimulation is not
counterbalanced by sufficient vagal activation. As a result, the vagal sinus
control is reduced and the baroreflex sensitivity is blunted [9, 10, 11]. This
phenomenon has been used to stratify the mortality risk in post-myocardial
infarction [12, 13, 14, 15, 16] and CHF [17] patients. In fact, it is possible to
observe a reduction in the heart rate variability in all of the
electrocardiographic (ECG) spectral components [9, 18, 19] and an increase in the
temporal dispersion of left ventricular repolarization in both post-myocardial
infarction and CHF [19, 20, 21, 22, 23, 24]. However, the final result was a
reduction in vagal sinus control, and the vagal nerve activity recorded directly
from the left thoracic vagal nerve increased in experimental CHF [25, 26], as
shown in Fig. 2. In this figure, changes in baroreflex sensitivity are reported
based on the phenylephrine method (BS
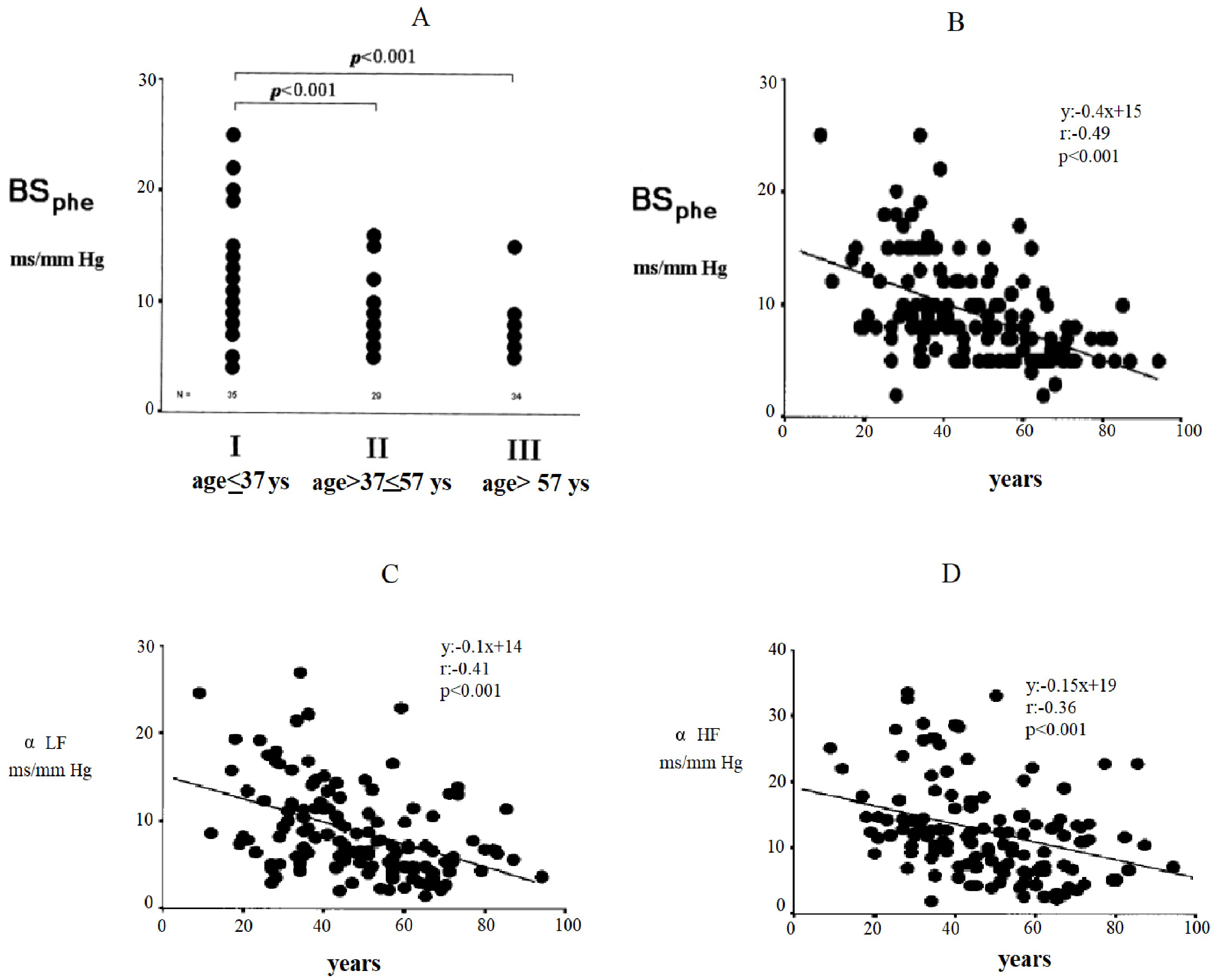
Changes in baroreflex sensitivity by the phenylephrine method
(BS
Probably, CHF prevents the conversion of vagal nerve activity in a reduced chronotropic response at the sinus node level [25, 27]. In other words, CHF reduces the vagal nerve capacity to modulate the respiratory oscillation of the heart rate at the sinus node level. Normally, in fact, the autonomic nervous system modulates the heart rate respiratory oscillation, also known as “respiratory sinus arrhythmia”. On the contrary, during CHF, the autonomic nervous system becomes unable to modulate the heart rate variability, and the loss of this physiological property is associated with the severity of symptoms, sympathetic hyperactivity, and an increase in cardiovascular mortality and the risk of sudden death.
Despite the progress of knowledge and the use of new drugs and devices, as previously emphasized, CHF remains a progressive, clinical syndrome with recurrent acute decompensations and severe prognoses. In fact, the hospital readmission rates of CHF patients are about 24%, 30%, and 50% within 30 days, 3 months, and 6 months, respectively. The major causes of these rehospitalizations are characterized by respiratory symptoms or fluid retention and pulmonary congestion, with the worsening of pre-existing dyspnea and/or systemic edema [3]. The precipitating factors of acute decompensated CHF are numerous, but the most important factors are concurrent infections, atrial fibrillation, uncontrolled arterial hypertension, acute coronary syndrome, and low compliance with the drugs or dietary prescriptions; however, at least 50% of cases remain unknown, and, in most cases, multiple factors can be identified [28, 29]. Some studies have reported that the mortality rate is related to the frequency of decompensated episodes [30, 31]. It was hypothesized that each acute episode increases the mortality rate because the pre-existent structural heart disease tends to worsen. This phenomenon seems to be associated with an acceleration of pathologic remodeling (Fig. 3), demonstrated by a transient increase in ultra-sensitive troponin I and extracellular matrix turnover (matrix metalloprotease 2, matrix metalloprotease 1, and procollagen type III N-terminal peptides) [32, 33].
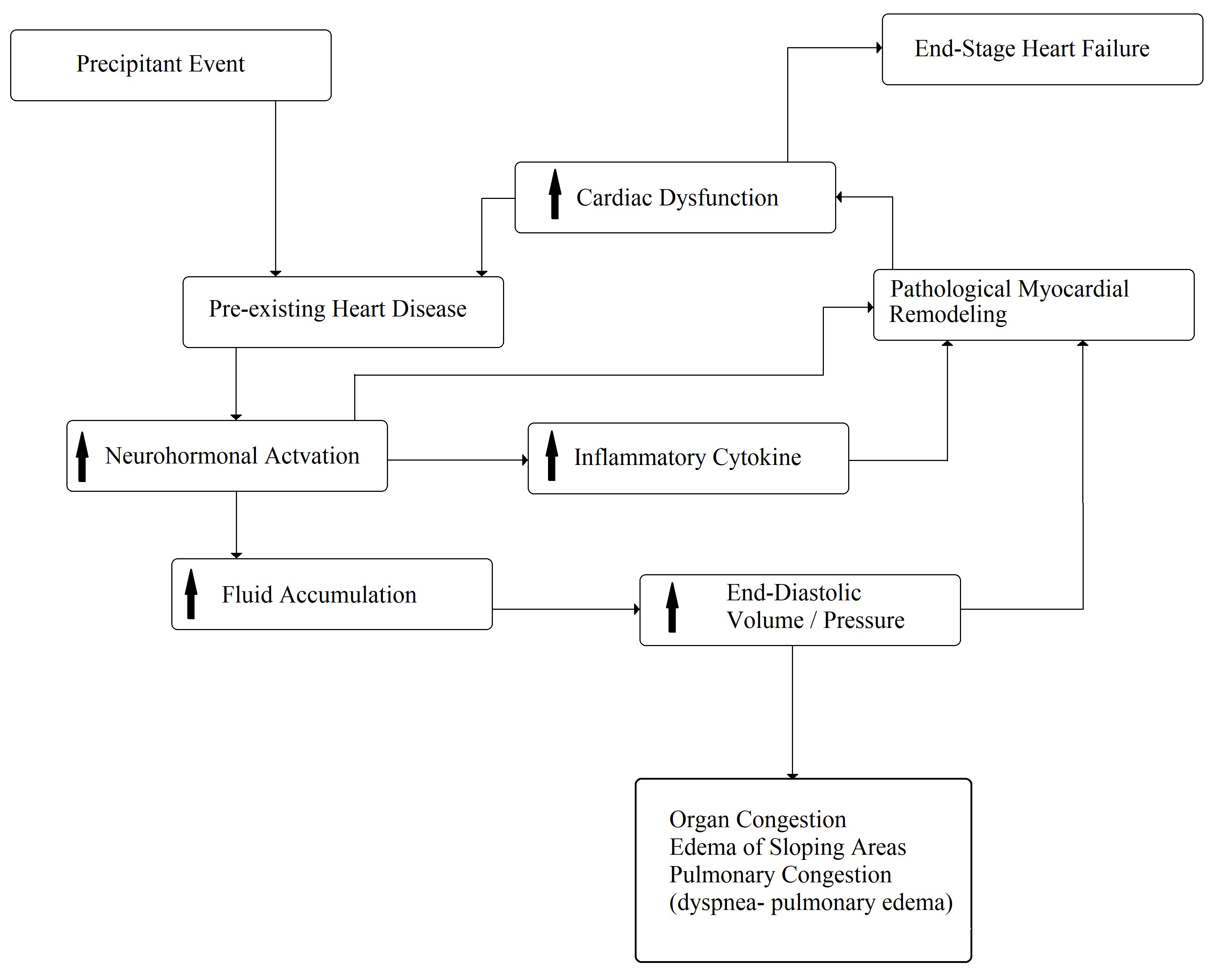
Pathophysiology of acute decompensated chronic heart failure. The arrows mean “increase”.
Especially in acute decompensation, a key role could be played by the
inflammatory state. For example, in some clinical trials on CHF, the prevalence
of inflammation was about 57% [34], which became more and more relevant with the
aging process [35], systolic or diastolic dysfunction [36], and decompensation
severity [35]. For instance, some authors recently reported that in a cohort of
in-hospital elderly (median age of 85 years) patients with decompensated CHF
[37], the prevalence of the inflammatory state was about 86% (median high sensitivity C reactive proteine (hsCRP): 3.46
mg/dL). Regarding the pathophysiologic mechanism, it was reported that the
pro-inflammatory cytokine interleukin-1 and tumor necrosis factor-
As already stated, HF is among the most frequent causes of hospitalization in the medical and cardiology departments in European Countries [39], presenting a mortality rate of 40% within 1 year after the first hospitalization and a 5-year survival rate between 25 and 38%, based on sex, age, comorbidities, and the severity of the HF itself.
Notoriously classified on the basis of left ventricular function [4], HF is often a consequence of coexisting ischemic, hypertensive, diabetic, or other heart disease, which has resulted in profound changes in the contractile capacity of the heart and in the patient’s hemodynamics [40, 41, 42, 43]. As evidenced by many recent studies, symptoms of HF in women are often vague and unfortunately not recognized as “typical” [44, 45]. The physiopathology of the damage is profoundly different; endothelial dysfunction, micro-vessel damage [46], and comorbidities (e.g., diabetes mellitus, systemic arterial hypertension, autoimmune diseases, and drug cardiotoxicity) are, in women, frequently associated with a more preserved ejection fraction than in men [40]. If the main cause of HF in men is ischemic heart disease caused by obstructive coronary artery disease, in women, significant microcirculation and small vessel ischemic damage (myocardial infarction with no obstructive coronary arteries, myocardial infarction with non-obstructive coronary arteries (MINOCA)) is more commonly observed [47, 48]. For this reason, symptoms, clinical course, and prognosis are peculiar, as is the therapeutic response [49]. Symptoms of HF in women are often vague and considered “atypical”, with frequent delays in diagnosis and belated arrival to the doctor’s attention. For this reason, women are often much older at the time of diagnosis, and they usually show a higher degree of co-pathology and polypharmacy than men, with a higher risk of the drug’s side effects and poor adherence to therapies [40]. In this situation, female patients are highly exposed to the risk of iatrogenic damage, poor compensation, and therapeutic failure. Age is also often a limiting factor for enrollment in clinical trials, which, therefore, frequently has a gender bias [40].
It has also been shown that female patients with heart failure, while significantly benefiting from the implantation of devices (pacemakers and implantable defibrillators) or resynchronization therapy, rarely receive an indication for this type of intervention [50].
Prognostic evaluation is also particularly difficult in the female population affected by heart failure. The Heart Failure Survival Score [51], Seattle Heart Failure Model [52], Meta-Analysis Global Group in Chronic Heart Failure [53], and Metabolic Exercise Cardiac Kidney Index [54] Score, used for the risk stratification of death or the need for the urgent cardiac transplantation of patients suffering from heart failure, do not allow for a sex-specific evaluation, even though substantial discrepancies between the two sexes have been identified in some studies [55, 56]. In fact, compared to scores describing severe disease, women often have better prognoses. This could depend on the underlying differences in the pathophysiology of cardiac damage that determine a serious and more disabling clinical presentation, which may not correspond to an equally poor prognosis.
However, it should be reiterated that HF in elderly women causes an extremely significant proportion of repeated hospitalizations (“revolving door”), with episodes of acute HF (dyspnoea, acute pulmonary edema) at increasingly shorter intervals, until death [57]. This not only results in high management costs but also has a severe impact on the quality of life.
Advanced age at diagnosis and the difficulty of stratifying the risk for the decompensated patient are reasons why female patients are historically less eligible for heart transplantation [58].
This evidence clearly demonstrates how a gender-specific approach to this particularly complex condition is absolutely indispensable and necessary [45] in order to guarantee target diagnoses, treatments, and therapies to reduce morbidity, mortality, and management costs (Table 1).
Specific characteristics of chronic heart failure in women | |
Different pathophysiology | Endothelial dysfunction; micro-vessel damage (diabetes, arterial hypertension, estrogen depletion after menopause) |
Most common symptoms | More severe weakness; reduced exercise tolerance; diaphoresis; more pronounced dyspnea; precordial palpitations |
Diagnostic delay determining a later-in-life diagnosis | High degree of polypathology, polypharmacy, and iatrogenic damage; reduced access to the heart transplant; exclusion from clinical trials |
Difficult prognostic evaluation | Scores/risk charts formulated on male models, and therefore, not designed and studied for women; no score currently takes into account sex-specific risk factors |
High “revolving door” risk | High costs for health systems; reduced patient quality of life |
Specific diagnostic and therapeutic interventions, together with an education program to enhance clinicians’ and women’s awareness, are desirable [59]. These would make it possible to reduce repeated hospitalizations in the health sector, reduce mortality and morbidity, and, at the same time, improve the quality of life in the female population, which, historically, has been and is considered protected from cardiovascular disease and its consequences.
All biomarkers should be designed, experimented, and implemented to simplify the diagnostic process. In fact, the essential features of biomarkers are the highest levels of specificity, sensitivity, and predictive values. Clearly, these characteristics are not enough nowadays. In fact, a specific and modern CHF biomarker should also present other features: rapid measurement, noninvasive, inexpensive, easily collectible, repeatable, and reproducible. Finally, it should be able to improve the diagnostic process, monitor the progress of the disease during treatments, and be a reliable prognosis tool at the same time.
Every possible biomarker must have a solid pathophysiologic basis and, as CHF is a complex syndrome, many biomarkers have been proposed and tested (Fig. 4) [60]. Although countless possible biomarkers based on different pathophysiologic substrates have been studied, natriuretic peptides have been the most extensively studied [60, 61]. Natriuretic peptides are a group of similar peptides of atrial, ventricular, and endothelial origin, but the most studied, as CHF markers, are B-type natriuretic peptide (BNP) and its prohormone, namely, N-terminal pro-B-type natriuretic peptide (NT-proBNP) (Fig. 5). The biological activity of natriuretic peptides is to increase diuresis, natriuresis, and vasodilatation and to inhibit the renin–angiotensin–aldosterone and the sympathetic system’s overactivity (Fig. 5). Both BNP and NT-proBNP are produced by myocytes as a consequence of myocardial wall stretch and are specifically induced by a pathological increase in LVEDV/P. NT-proBNP and BNP are cleaved from pre-proBNP, and NT-proBNP is biologically inactive but has a more stable status. In fact, the half-life of BNP is 20 minutes, while NT-proBNP has a half-life between 60 and 120 minutes [60, 61, 62], with a ratio higher than 1:6 [63]. The blood kinetics of BNP and NT-proBNP are influenced by CHF exacerbation but also by aging, gender, renal function, obesity, genetic factors, comorbidities, obesity, and LVEDV/P.
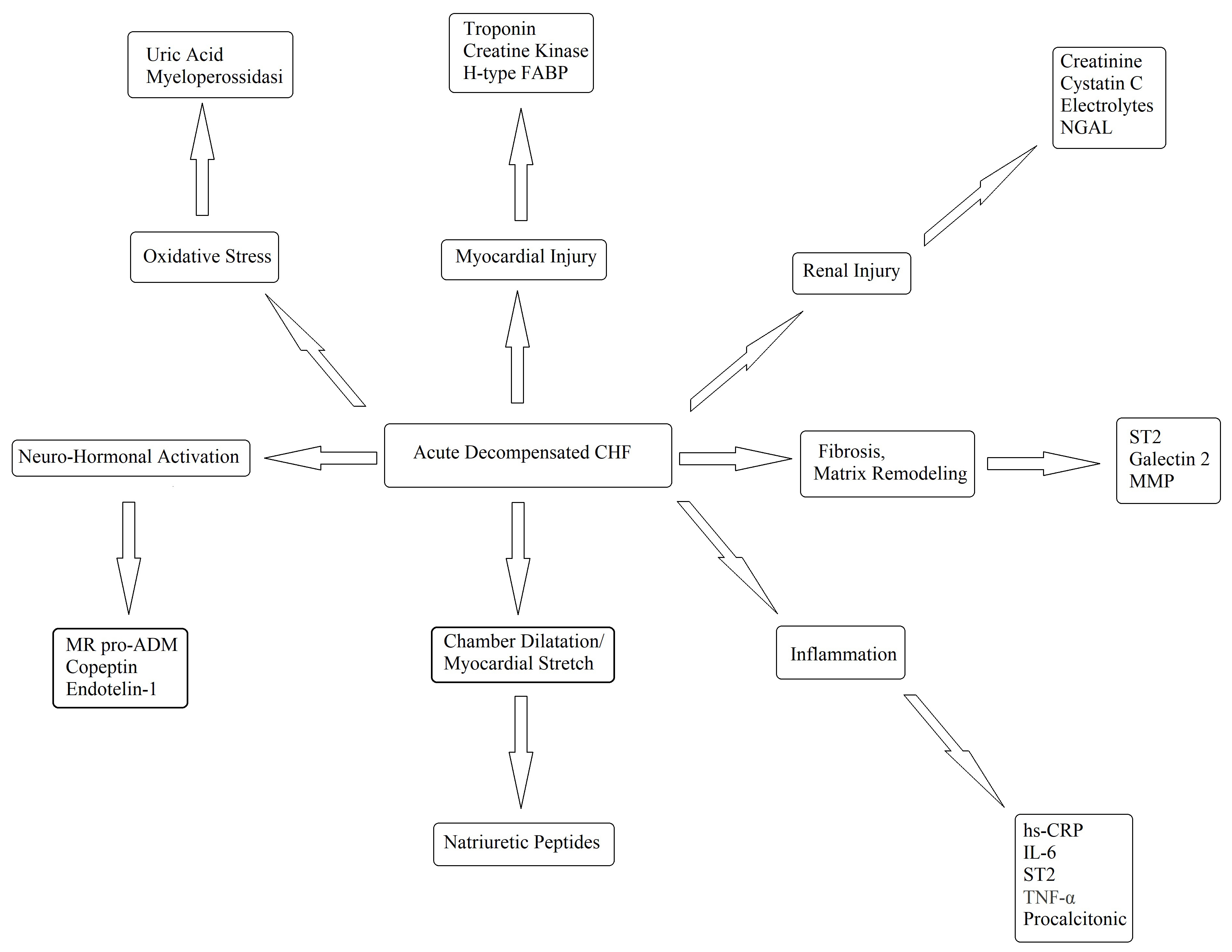
Potential biomarkers in the diagnosis and management of decompensated CHF. FABP, fatty acid-binding protein; hsCRP, high-sensitivity C-reaction protein; IL, interleukin; MMP, matrix metalloproteinase; NGAL, neutrophil gelatinase-associated lipocalin; ST2, suppressor of tumourigenicity-2; TNF, tumor necrosis factor; CHF, chronic heart failure; MR pro ADM, mid-regional proadrenomedullin; IL-6, Interleuki-6.
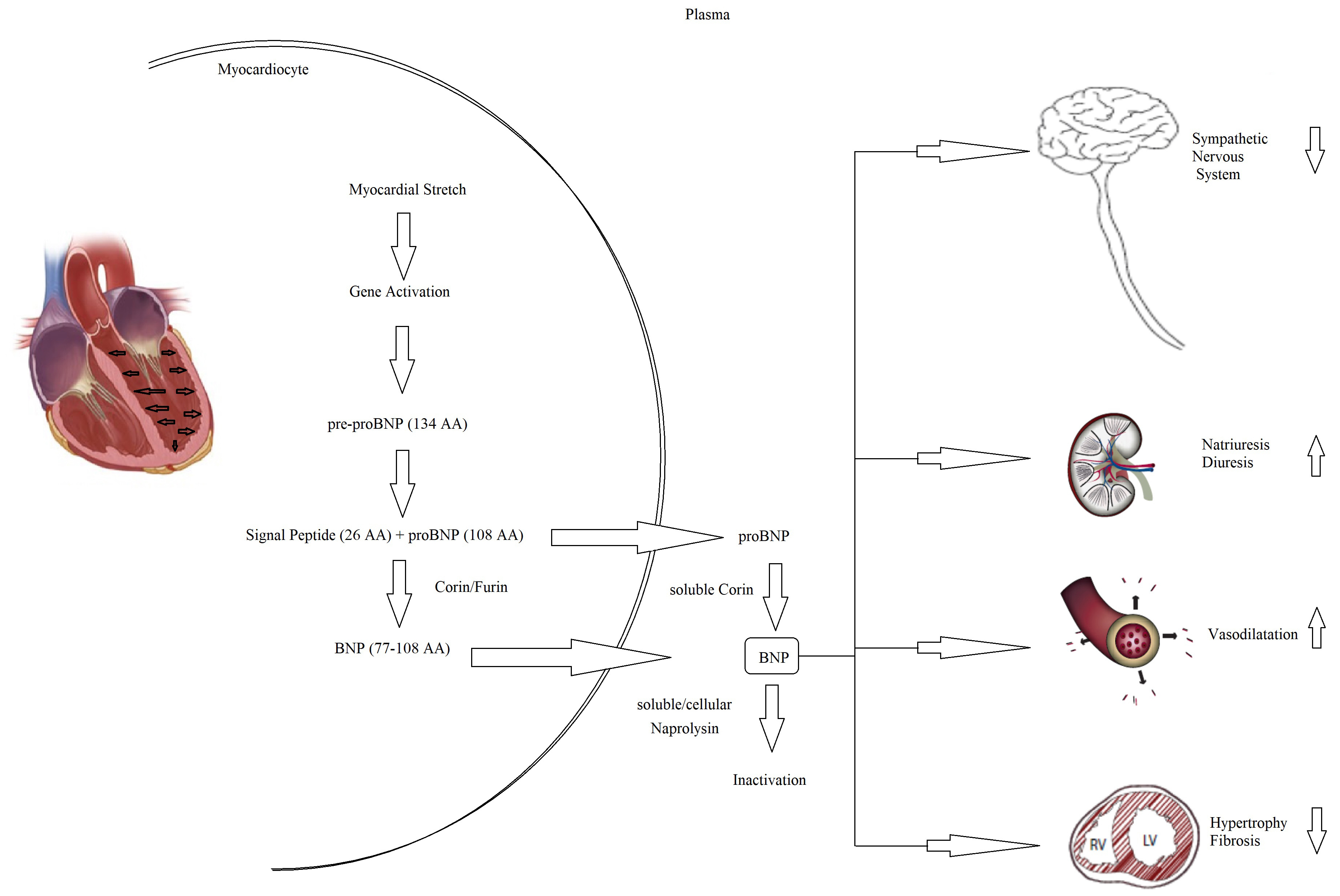
Pathophysiology of NT-proBNP and BNP. BNP, B-type natriuretic peptide; NT-proBNP, N-terminal pro-B-type natriuretic peptide; AA, aminacyd.
Iwanaga Y et al. [64] showed in a previous study that plasma BNP levels reflect left ventricular end diastolic wall stress (EDWS) more than any other parameter previously reported, not only in patients with HFrEF but also in patients with HFpEF. The relationship of left ventricular EDWS to plasma BNP may be an important parameter to consider in HFpEF patients, providing a better fundamental understanding of the individual heterogeneity among BNP levels and their clinical utility in the diagnosis and management of HF (especially in specific groups: e.g., elderly, women).
Many clinical trials have assessed the role of BNP and NT-proBNP in the
diagnosis, management, and prognosis of HF. The end-point of these studies was to
assess the correct HF diagnosis in patients with breathlessness. Particularly,
the first studies conducted on these issues were the Breathing Not Properly Study
for BNP and the ProBNP Investigation of Dyspnea in the Emergency Department
(PRIDE) for NT-proBNP [65, 66]. In both, the natriuretic peptides were the best
significant predictors of the final diagnosis [65, 66]. Although they have been
considered in the clinical practice guideline as Class I and level of evidence A,
some critical issues remain unresolved [4, 67]. In fact, as abovementioned, some
comorbidities (diabetes, atrial fibrillation, obesity, renal insufficiency),
gender, and age have a not negligible role on the plasmatic levels of both
natriuretic peptides. For example, the International Collaborative of NT-proBNP:
Re-evaluation of Acute Diagnostic Cut-Offs in Emergency Department Study
(ICON-Reloaded) tried to dampen the age influence on natriuretic peptides [38].
In fact, the authors stratified for age three different NT-proBNP cut-offs (
Another serum biomarker, specifically studied for HF diagnosis, was the highly sensitive troponin (hs-Tn). Troponins are proteins involved in skeletal and cardiac muscle contraction. Specific cardiac isoforms were found to be increased not only in myocardial necrosis but even in decompensated CHF, so they could be considered a marker of myocardial necrosis in acute myocardial infarction and also a marker of myocardial wall stress during acute decompensated CHF injury. In the Acute Decompensated Heart Failure National Registry (ADHERE), the authors reported an increase in in-hospital mortality risk associated with higher levels of hs-Tn [69]. These data have been confirmed in further trials on acute decompensated CHF, about the global [70, 71] and cardiovascular mortality [72]. Finally, other markers of hypertrophy, remodeling, and inflammation (Fig. 4) have been tested in many studies with different end-points, demonstrating more usefulness for interpreting the different pathophysiologic aspects than making a diagnosis and risking stratification, so they are not routinely used.
Finally, the possibility of obtaining the dosage of a marker from the capillary blood test is of great clinical relevance (as the glucose from the fingertip in diabetic patients). In other words, this could allow for the analysis of the biomarker in different settings: at the point-of-care or at the patient’s home, to avoid or choose hospitalization. In fact, nowadays, specific instruments are commercially available to measure NT-proBNP [73] and troponin [74, 75, 76], which are detected from a capillary blood sample using a single-use test strip. Similar to capillary glucose test, the blood drop test should be performed by the patients themselves. This hypothetical procedure could allow for the patient’s follow-up at home (Fig. 6).
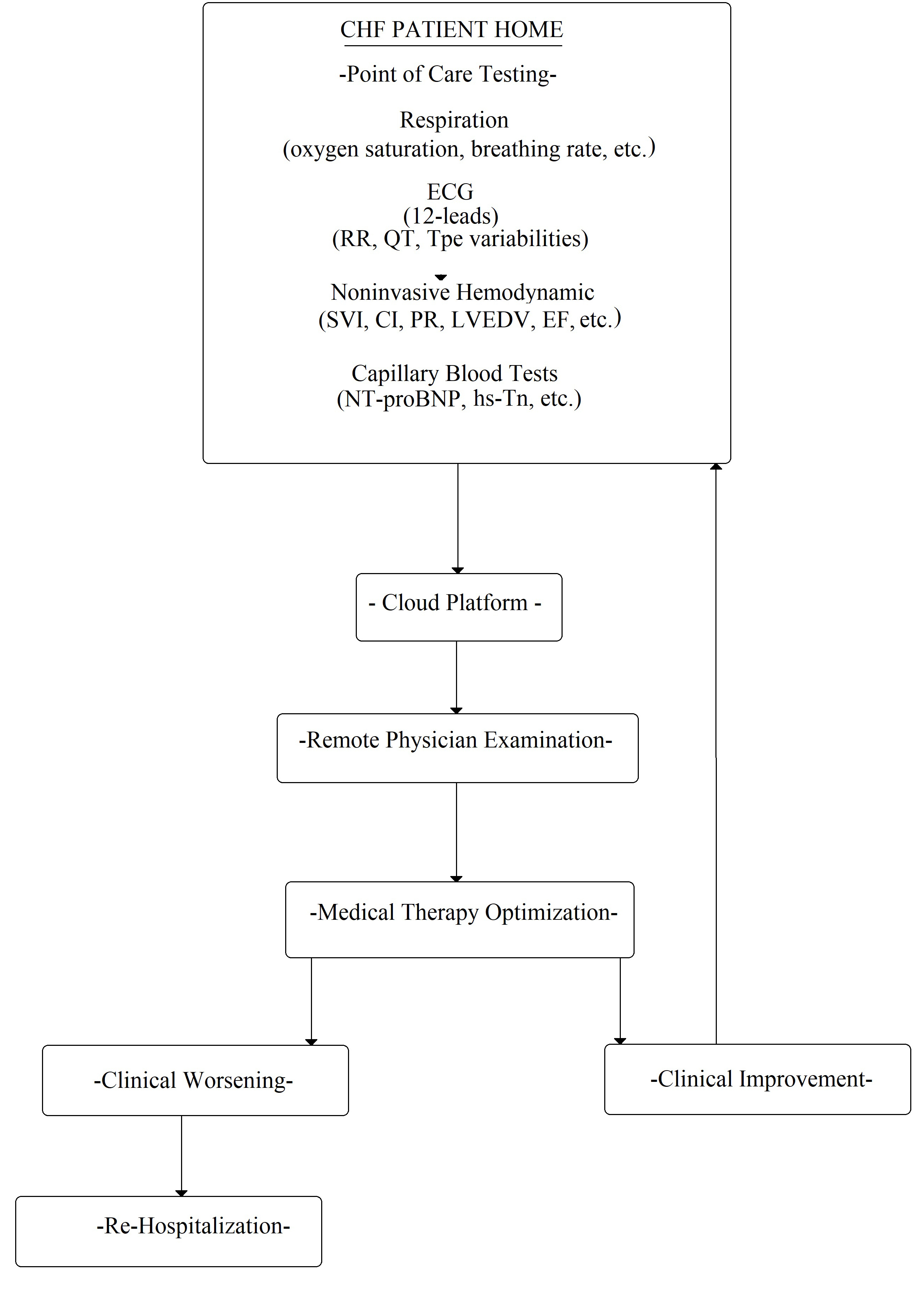
Flowchart of possible CHF patient management at home. ECG, electrocardiogram; RR, wave interval; QT, QT interval; Tpe variabilities, Tpeak-end variabilities; SVI, stroke volume index; CI, cardiac index; PR, pulse rate; LVEDV, left ventricle end dyastolic volume; EF, ejection fraction; NT-proBNP, N-terminal pro-B-type natriuretic peptide; hs-Tn, high sensitivity Troponine.
The greatest challenge in CHF in modern medicine is patient management. In fact,
the acute symptom re-exacerbations and the consequent rehospitalizations are a
serious concern for the health system. As CHF mortality has been reduced and life
expectancy has increased, the prevalence of failing to heal has grown. Moreover,
the more the survival rate increases, the more re-exacerbations,
rehospitalizations, and healthcare costs will do. At present, despite new
promising drugs and devices, acute decompensated CHF remains an unsolved issue.
Thus, to avoid repeated hospitalizations, telemonitoring CHF patients could be a
key point. This monitoring should be available especially at home, with possible
remote access by physicians. In this way, the drug therapy could be changed and
adapted, possibly avoiding re-exacerbation, rehospitalization, and mortality
(Fig. 6). The ideal candidates among noninvasive CHF markers are electrical
signals, offering standard electrocardiographic and hemodynamic (bioimpedance
cardiography) information with numerous advantages. In fact, they are simple to
detect, but also repeatable, transmissible, and inexpensive. It is possible to
distinguish two kinds of signals: those based on an ECG in twelve leads and those
based on the RR interval or repolarization variabilities. Obviously, a standard
ECG is essential in the early diagnosis of arrhythmias and myocardial ischemia or
necrosis, but it could offer other significant benefits [77]. All considered, an
ECG serial analysis based on the variability of different QRS intervals could
offer new insight into CHF exacerbation phenomena. In particular, short-period RR
variability [18, 25, 78], available with many interpretative algorithms and
methods, could be used to evaluate the changes in autonomic control during and
before a CHF decompensation, leading to drug therapy changes, in order to reduce
sympathetic overdrive [11, 20, 79, 80, 81]. A more modern alternative to RR
variability is represented by the QT variability. Obtained by different formulas
[20], this is a short-term marker of the temporal dispersion of left ventricular
repolarization. Calculated by 5-minute ECG recordings, it expresses the temporal
inhomogeneity of myocardial repolarization and, especially in post-ischemic [82]
or primitive dilative cardiomyopathy [20, 83], it is a marker of sudden cardiac
death or cardiovascular mortality [20, 21, 84]. In particular, the most used
repolarization marker is the QT variability index (QTVI). QTVI was introduced by
Berger in 1997; the author stated the QTVI as the logarithm of the ratio between
QT variability (QT variance/QT mean
A meta-analysis based on almost 2000 healthy subjects indicated that the normal
QTVI and QT
The possibility to remotely monitor the hemodynamic status of CHF patients was studied with different implantable devices, and it was also offered as an optional feature in pace-makers, resynchronization therapy, and implantable converter defibrillator devices. Nevertheless, the usefulness of these devices is still debated regarding the potential mortality risk reduction; on the contrary, they seemed more useful to individuate an increased risk of rehospitalization [99, 100].
Historically, many studies have investigated the possibility of using intrathoracic bioimpedance measurements as a method of evaluating patients at high risk for exacerbation of heart failure or cardiovascular events over time [101, 102, 103]. The fundamental assumption, underlying the remote monitoring of CHF patients, is that before decompensation and rehospitalization, a period from days to weeks occurs in which the left ventricular end-diastolic or filling pressure increases [104]. In other words, by optimizing the therapy, rehospitalizations and re-exacerbations could be avoided [105]. Thus, how to obtain a measure of the left ventricular end-diastolic pressure to anticipate the symptoms of decompensation is an open issue. At present, these parameters are invasively acquired. During the last two decades, three different possible invasive technological solutions have been developed. The first device was able to continuously monitor the right ventricular pressure with an implantable sensor; the pressure in this cardiac chamber is considered a surrogate for PAWP and LVEDP. This device, similar to a pacemaker and named the Chronicle implantable hemodynamic monitor (IHM) (Medtronic, Inc, Minneapolis, Minnesota), can be subcutaneously implanted in the pectoral muscle area. It is provided with a lead with a pressure sensor near the tip and placed in the right ventricular outflow tract or septum. A randomized prospective single-blind parallel trial was conducted to prove the efficacy of this device; this study was named the Chronicle Offers Management to Patients with Advanced Sign and Symptoms of Heart Failure (COMPASS-HF) [106]. The major aim of this study was to prove if the Chronicle IHM in CHF patients provided hemodynamic information useful for optimizing the therapy and reducing mortality and rehospitalization risk. The trial was conducted in 274 centers, enrolling III/IV NYHA class CHF patients, and the follow-up was a period of six months [106]. This trial reported a reduction of 21% of HF-related events (hospitalization, emergency, or urgent visits requiring intravenous therapy), but this result was not statistically significant in comparison to the control group. However, a retrospective analysis demonstrated a significant reduction of 36% of rehospitalization in the group with activated Chronicle IHM. The group with activated Chronicle IHM had 28% more adjusted therapies in comparison to the control group. We also believe that some severe limitations, such as, for example, the incompatibility with atrial pacing, cardiac resynchronization therapy, and magnetic resonance imaging induced early obsolescence of this device.
A second, more advanced device was developed to directly and remotely monitor
the pulmonary artery pressures and to guide the therapy; the
Cardio-Microelectromechanical system (CardioMEMS) (Abbott, Sylmar, CA, USA) can
be implanted via the femoral vein during a right cardiac catheterization [105, 106]. Once implanted, this pulmonary wireless sensor is externally powered by
means of radiofrequency energy, and it was designed to be permanently placed in
the pulmonary artery. CardioMEMS was studied in a large single-blind randomized
trial named the US CardioMEMS Heart Sensor Allows Monitoring of Pressure to
Improve Outcomes in NYHA Class III Heart Failure Patients (US CHAMPION). A total
of 550 III NYHA class patients were enrolled in this trial, and the primary
end-point was the rate of rehospitalization, checked at an 18-month follow-up.
Although the US CHAMPION did not demonstrate a reduction in mortality, the
rehospitalization rate was significantly reduced by 37% [107, 108]. Furthermore,
during the following open-label period, this device showed an additional
reduction in the rehospitalization rate (48%) [109]. Finally, the CardioMEMS was
approved by both the Food and Drug Administration and the European Conformity. US
and European authors are studying a new device similar to the CardioMEMS, but
with more hemodynamic parameters, named the Cordella
Obviously, pulmonary pressure is not only related to pulmonary congestion and the left ventricular end-diastolic pressure but also to the pulmonary pre-capillary resistance; for this reason, a sensor was designed to directly measure the pressure in the left atrium.
In a recent paper [112], the authors emphasized the need to study the longitudinal cardiovascular pressure–volume relationships in the dynamic clinical environment of HF. Their findings do indicate that pressure-based assessment of congestion in ambulatory HF patients does not accurately represent intravascular volume; nevertheless, they assumed that the pressure changes remain indicative of HF exacerbations. Additional volume-based phenotyping may be required to guide decongestion strategies in patients with HF. There is initial evidence that patients with a low or normal volume (independent of peripheral arterial disease (PAD)) are at the greatest risk for HF hospitalization [112]. Therefore, the third technology solution involves the implantation of a sensor in the left atrium directly. The HeartPOD system (Abbot, formerly St. Jude Medical/Savacor, Inc.Abbott Parl, IL, USA) is a sensor designed to be implanted in the left atrium and to measure its pressures [113]. This invasive device is implanted via the femoral vein and by trans-septal puncture. It is powered and measured by a wireless advisory module through the skin. The results on its safety were disappointing because the Left Atrium Pressure Monitoring to Optimize Heart Failure Therapy (LAPTOP-HF) study, designed to evaluate its efficacy in guiding medical therapy, was interrupted early due to an excess of procedure-related complications [114]. Despite this interruption, the results were evaluated in the remaining 486 patients during twelve months of follow-up, and the HeartPOD was capable of significantly reducing the rehospitalization rate by 41% [114].
All in all, the possibility of monitoring the pressure in the left atrium has certainly brought clinical advantages beyond the problems of implantation. In fact, the next generation of studied implantable devices is the V-LAP (Vectorious Medical Technologies, Tel Aviv, Israel), and it could represent a new tool for left atrium pressure monitoring [115]. At present, a trial on its safety, usability, and efficacy is still ongoing. Among the physiologic parameters, the V-LAP will take into account the heart rate variability and a new generation of decision-support software [116]. Obviously, the data unavailability in elderly and fragile patients, which is the category of subjects most affected by CHF, remains a critical issue. Thus, ethical or rational issues could arise proposing a diagnostic invasive device to a fragile patient with many comorbidities.
A second critical point is represented by the device costs. A cost-effectiveness analysis was published on the CardioMEMS using the CHAMPION trial data. The CardioMEMS showed an increase in the cost-effective ratio in comparison to a standard approach also using the five-year outcome data. In particular, the CardioMEMS cost-effective ratio was USD 44,832 per quality-adjusted life-year higher than the standard cure [117].
Currently, noninvasive measurement of the left ventricular end-diastolic
pressure is impracticable, but bioimpedance cardiography could allow the
noninvasive evaluation of hemodynamic parameters (stroke volume and cardiac
output) and tele-monitoring should be possible. The bioimpedance is based on the
calculation of the systolic and diastolic difference of electrical impedance by
cutaneous leads; in fact, the thoracic water volume changes during the cardiac
cycle and it is able to influence the electrical impedance. Obviously, the
electrical neck-thorax impedance difference is related to the systolic and
diastolic ventricular volumes, so, by appropriate algorithms, the stroke volume
(SV) and other hemodynamic noninvasive derived data could be collected [118, 119]. Nowadays, domestic hemodynamic self-monitoring by bioimpedance cardiography
devices is not possible and only available for medical clinical research purposes
[120]. In this era, the biomedical technology industries could easily develop a
simple device for the self-monitoring of hemodynamic parameters so that the
patients or their caregivers could easily learn to place six skin leads (two on
the left base of the neck—supraclavicular fossa, two at the midpoint of the
thorax, and two for the ECG), recording their own hemodynamic data via a
smartphone [118, 119]. For example, the PhysioFlow (Manatec Biomedical, Poissy, France)
is able to provide an interesting noninvasive systolic and diastolic hemodynamic
dataset [121, 122]. The systolic parameters are the SV, SV index (Svi), cardiac
output (CO), cardiac index (CI), systemic vascular resistance (SVR), systemic
vascular resistance index (SVRi), ejection fraction (EF), contractility index (CTI), left ventricular
ejection time (LVET), and left cardiac work index (LCWi). Moreover,
the diastolic parameters are the LVEDV and early diastolic filling ratio (EDFR).
Briefly, the second derivative of impedance (dZ/dt) measures the temporal
variation of impedance, and it is acquired for the calculation of the SV with the
following formula: SV = kꞏ[(dZ/dt
Finally, the diastolic parameters are the diastolic end diastolic volume (EDV) and the EDFR. The LVEDV is calculated as SV/EF (mL), and the EDFR is obtained by the dZ/dt as the ratio between the diastolic and systolic waves [118, 124]. The EDFR is correlated positively to the integral of the Doppler echocardiographic A wave and negatively to age [125]. Two examples of noninvasive monitoring by bioimpedance (PhysioFlow) in two HFrEF hospitalized patients are reported in Fig. 7A,B, at the time of admission (#1) and discharge (#2) to the hospital. The patient (Fig. 7A) reduced the LVEDV by 31%; this datum is confirmed by the reduction of the NT-proBNP by 55% (from 2910 to 1320 pg/mL). On the other hand, the same patient showed an increase in the SVRi and a decrease in the CI (Fig. 7A). The other patient (Fig. 7B) had an increased LVEDV by 41%, despite an increase in drug therapy, at the time of hospital discharge. The same subject did not have any changes in the NT-proBNP blood level in comparison to the moment of admission. Probably, this patient could have had a higher risk for future decompensation. Obviously, bioimpedance cardiography could never replace the more complex or invasive methods but could be used to monitor patients in remote positions (at home or in a nursing home) after discharge. For example, our preliminary data indicated that the LVEDV obtained in hypertensive subjects with a PhysioFlow and standard echocardiography are significantly related (n.: 19; r: 0.676; p: 0.003) [118].
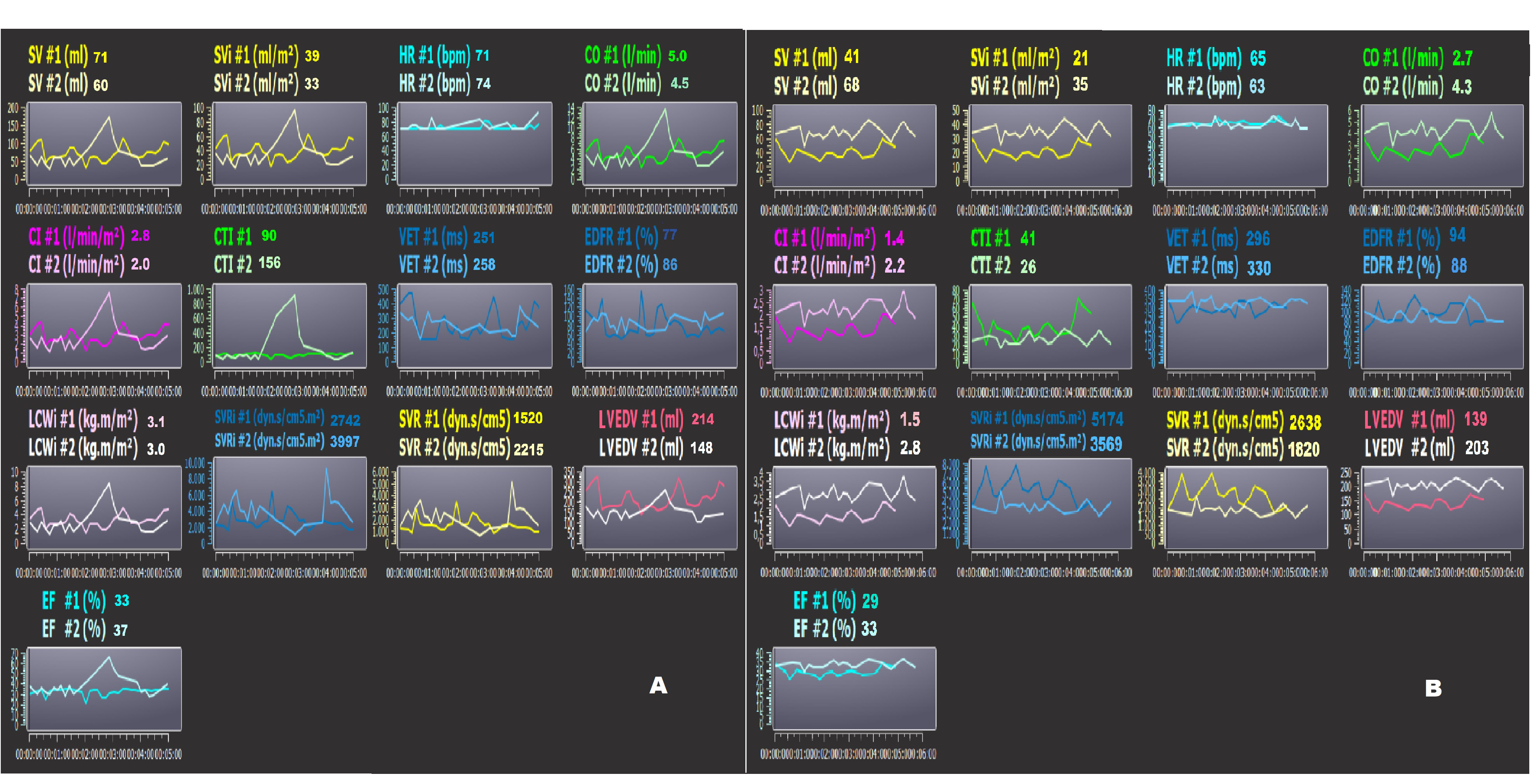
Bioimpedence PhysioFlow analysis. (A,B) two patients’ bioimpedance data (PhysioFlow) at the time of admission (#1) and discharge (#2) to the hospital. Note the patient in Panel A had decreased LVEDV, but the same subject showed an increase in SVRi and decrease in CI. The patient in Panel B had increased LVEDV at the time of discharge. In this subject, the risk of decompensation could be higher. SV, stroke volume; SVi, stroke volume index; CO, cardiac output; CI, cardiac index; SVR, systemic vascular resistance; EF, ejection fraction; CTI, contractility index; VET, left ventricular ejection time; LCWi, left cardiac work index; LVEDV, left ventricular end-diastolic volume; EDFR, early diastolic filling ratio; SVRi, systemic vascular resistance index; HR, heart rate.
Finally, some other techniques should be taken into account in order to better quantify the decompensation degree of CHF patients. Remote dielectric sensing (ReDS) has been proposed for the telemonitoring of CHF patients [126]. ReDS, which is a novel noninvasive wearable device to quantify the degree of pulmonary congestion easily and quickly within a minute, would be a promising supportive tool to guide titration of the dose of diuretics in patients with congestive heart failure in outpatient clinical follow-up. ReDS detects acute heart failure similar to the lung ultrasound score and primarily identifies the acute heart failure patients who have congestion on a chest Computer Tomography [126, 127].
The continuous aging of the population and the recent pandemic have highlighted the increasing need to promote health and quality of life, particularly for patients affected by chronic diseases. People and healthcare professionals should increase awareness and knowledge about chronic heart failure burden in the healthcare systems. An approach specifically tailored to the patient’s characteristics (age, sex/gender, comorbidities) must be improved. The possibility of remotely monitoring chronic patients should be applied to all chronic heart failure subjects in order to guide therapeutic choices and avoid exacerbations. Noninvasive technologies specifically suitable for elderly and fragile patients with CHF are not widely available yet. Detection at the point of care of humoral biomarkers and electrical signals able to select subjects with a higher risk of CHF exacerbations should be pursued. Future research should aim towards this new frontier and beyond the challenges of the coming years.
Conceptualization, GP, FM and DM; methodology, GP, FM SS and DM; validation, GP, FM, SS and DM; formal analysis, GP and FM; resources, GP, FM and DM; data curation, GP and FM; writing—original draft preparation, GP, FM and DM; writing—review and editing, FM, SS and DM; visualization, SS and DM; supervision, GP and FM; project administration, GP, FM SS and DM; funding acquisition, n/a. All authors read and approved the final manuscript. All authors have participated sufficiently in the work and agreed to be accountable for all aspects of the work.
Not applicable.
Not applicable.
This research received no external funding.
The authors declare no conflict of interest. Damiano Magrì is serving as Guest Editor of this journal. We declare that Damiano Magrì had no involvement in the peer review of this article and has no access to information regarding its peer review. Full responsibility for the editorial process for this article was delegated to Leonardo De Luca and Sophie Mavrogeni.
Publisher’s Note: IMR Press stays neutral with regard to jurisdictional claims in published maps and institutional affiliations.