- Academic Editor
†These authors contributed equally.
Background: Rotational atherectomy (RA) is the major tool used to treat
severely calcified lesions in patients with coronary artery disease (CAD). The
relationship between coronary microvascular dysfunction and RA remains unknown.
Therefore, we attempted to explore the predictive implications of the coronary
angiography-derived index of microcirculatory resistance (angio-IMR) in CAD
patients undergoing RA. Methods: This retrospective study included 118
patients with severe coronary calcification who underwent a successful RA from
January 2018 to June 2021. The angio-IMR was calculated based on computed flow
and pressure dynamic principles to assess coronary microcirculatory function.
Follow-up was performed on all patients for major adverse cardiovascular events
(MACEs), including all-cause death, non-fatal myocardial infarction, target
vessel revascularization (TVR), and stroke. Results: The mean angio-IMR
for all patients was 25.58
Severe coronary artery calcification affects the outcome of percutaneous
coronary interventions (PCI), impairs stent expansion and causes dilatation
balloon rupture, increases the rate of stent target vessel failure and in-stent
restenosis, and increases cardiac death [1]. Rotational atherectomy (RA) is a
technique used to treat calcified lesions in coronary arteries, which can
increase the rate of procedural success and improve patient prognosis, especially
with the use of drug-eluting stents (DES). RA for plaque modification has become
an important technique to improve the outcomes following PCI [2, 3]. The
principle of RA is to use a rotating diamond-covered burr to differentially crush
the stiff plaque and fibrous tissue into particles of 7.0–7.5
The coronary microcirculatory system is mainly composed of pre-arterioles
(
However, it is unknown as to whether the formation of microparticles in severely calcified coronary lesions following RA increases the incidence of CMD, and the prognostic significance of CMD after RA. Consequently, in this study, we attempted to apply angio-IMR to assess coronary microvascular function in patients undergoing RA and follow these patients to assess their clinical outcomes.
This is a retrospective, observational study to determine coronary microcirculation and follow-up outcomes in 118 patients with severe coronary calcified lesions following RA at the Department of Cardiology, Shanghai Tenth People’s Hospital, Shanghai, China, between January 2018 and July 2021. All patient demographics, co-morbidities, examination results and angiographic characteristics, and key procedural details were recorded through the medical record system. Patients with acute coronary syndromes (including acute myocardial infarction and unstable angina), coronary artery bypass grafting, severe valvular heart disease, acute heart failure, malignant tumors with an expected survival of less than one year, and hemodialysis patients were excluded. The flow chart of the study is shown in Fig. 1. All procedures performed on patients followed the Helsinki Declaration. The study protocol was approved by the Ethics Committee of Shanghai Tenth People’s Hospital. Because data were collected retrospectively, informed consent on the use of coronary angiography was waived given the institutional ethics regulations with regard to observational study nature.
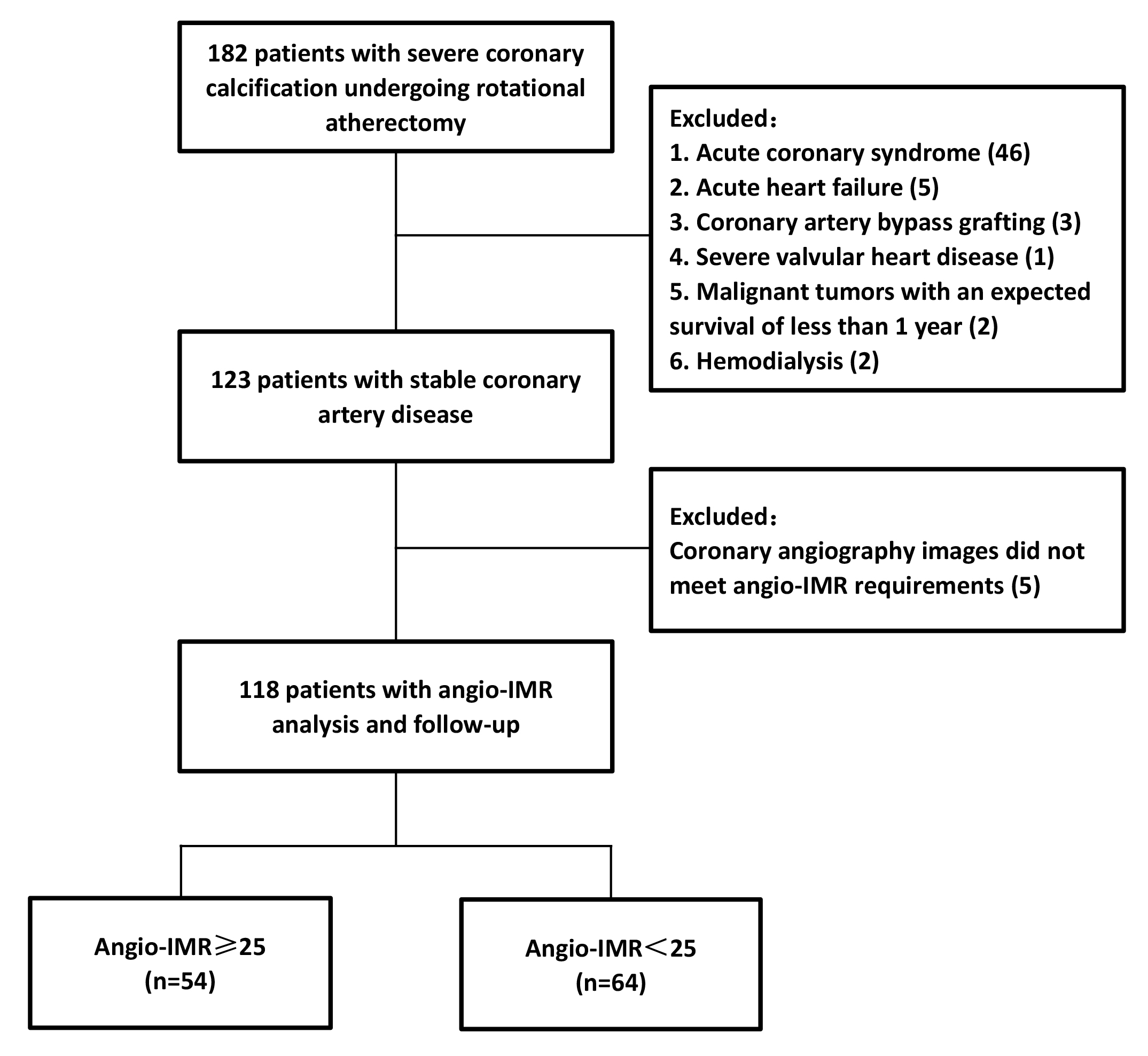
Study flow chart. Abbreviations: Angio-IMR, coronary angiography-derived index of microcirculatory resistance.
Coronary angiography and PCI were performed using standard techniques. RA was performed using the Rotablator™ Rotational Atherectomy System, Boston Scientific, USA. The rotational speed was between 120,000 and 200,000 rpm, and the rotational speed was not allowed to drop more than 5000 rpm. The burr moved in a pecking motion with a single run time of less than 20 seconds. Verapamil, nitroglycerin, and heparin continuously were infused intracoronary during the RA procedure. After RA, the lesion is adequately dilated using cutting balloons or dilated balloons at the operator’s discretion. When adequate pre-treatment results were obtained, one or more stents were implanted. All patients were pretreated with a loading dose of dual antiplatelet therapy before procedures. Angio-IMR and angiography-derived fractional flow reserve (angio-FFR) were calculated from post-interventional coronary angiography images.
The angio-IMR measurement was conducted using the FlashAngio software (Rainmed
Ltd., Suzhou, China). Details of the measurements and the angio-IMR procedures
have been previously described [12]. Briefly, digital imaging and communications in medicine images (DICOM) coronary angiography images
and the corresponding mean arterial pressure (MAP) were transferred to the
FlashAngio workstation, and the interrogated vessels were selected for
three-dimensional reconstruction. The estimated hyperemic Pa (Pa
The thrombolysis in myocardial infarction frame count method [14] was used to
calculate the mean flow velocity (V
K was a constant (K = 2.1) to adjust the difference between resting and hyperemic flow velocity [15]. The coronary angiograms were assessed by two trained cardiologists, and any disagreements were resolved by consensus. Fig. 2 shows a representative example of an angio-IMR analysis in patients undergoing the RA procedure.
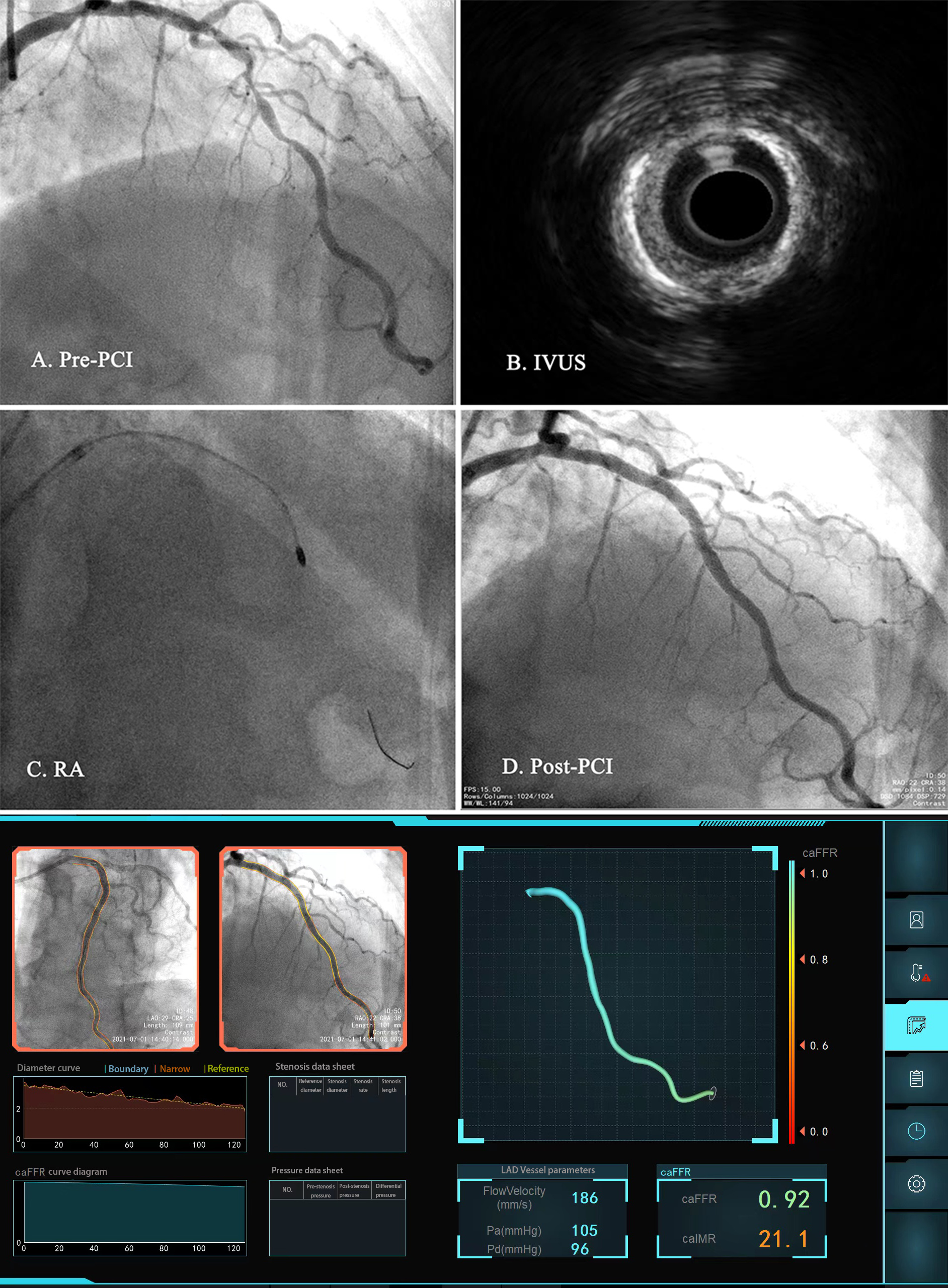
Representative cases of coronary artery disease patients undergoing rotational atherectomy. (A) Coronary angiography shows severe coronary stenosis and calcification in the LAD. (B) IVUS. (C) RA procedure. (D) Post-PCI. (E) Angio-IMR and angio-FFR calculation. Abbreviations: LAD, left anterior descending; IVUS, Intravascular ultrasound; RA, rotational atherectomy; PCI, percutaneous coronary intervention; angio-IMR, angiography-derived index of microcirculatory resistance; angio-FFR, angiography-derived fractional flow reserve.
Patients were followed for major adverse cardiovascular events (MACEs),
including all-cause death, non-fatal myocardial infarction, and target vessel
revascularization (TVR), by telephone interview or outpatient visit. All-cause
death was defined as mortality from all causes. Non-fatal myocardial infarction
was defined as ischemic symptoms and elevation of either troponin I
Continuous variables were expressed as mean
Clinical characteristics of the study population are presented in Table 1. A
total of 118 patients with severe calcification undergoing RA were enrolled
(72.39
Variables | Overall | Angio-IMR |
Angio-IMR |
p-value | ||
---|---|---|---|---|---|---|
(n = 118) | (n = 54) | (n = 64) | ||||
Demographics | ||||||
Age, years | 72.39 |
71.22 |
73.22 |
0.227 | ||
Male | 82 (68.9) | 42 (77.8) | 40 (62.5) | 0.073 | ||
Cardiovascular risk factors | ||||||
Hypertension | 93 (78.8) | 40 (74.1) | 53 (82.8) | 0.247 | ||
Diabetes mellitus | 58 (49.2) | 27 (50.0) | 31 (48.4) | 0.866 | ||
Hyperlipidemia | 13 (11.0) | 7 (13.0) | 6 (9.4) | 0.535 | ||
Smoking | 30 (25.4) | 12 (22.2) | 18 (28.1) | 0.463 | ||
Stroke | 26 (22.0) | 9 (16.7) | 17 (26.6) | 0.196 | ||
Prior PCI | 40 (33.9) | 16 (29.6) | 24 (37.5) | 0.368 | ||
LVEF, % | 57.98 |
58.70 |
57.45 |
0.443 | ||
Medication use | ||||||
Asprin | 113 (95.8) | 52 (96.3) | 61 (95.3) | 0.792 | ||
P2Y12 inhibitor | 117 (99.2) | 53 (98.1) | 64 (100) | 0.274 | ||
Statin | 115 (97.5) | 51 (94.4) | 64 (100) | 0.056 | ||
Beta-blocker | 76 (64.4) | 37 (68.5) | 39 (60.9) | 0.392 | ||
ACEI/ARB | 83 (70.3) | 35 (64.8) | 48 (75.0) | 0.228 | ||
Coronary angiography | ||||||
Target vessel | ||||||
Left main disease | 26 (22.5) | 9 (16.7) | 17 (26.6) | 0.196 | ||
RCA | 21 (17.8) | 14 (25.9) | 7 (10.9) | |||
LAD | 94 (79.7) | 39 (72.2) | 51 (85.9) | |||
LCX | 3 (2.5) | 1 (1.9) | 2 (3.1) | |||
Diameter stenosis | 86.86 |
87.93 |
85.95 |
0.174 | ||
TIMI grade | ||||||
0 | 5 (4.2) | 4 (2.3) | 1 (1.6) | 0.243 | ||
1 | 3 (2.5) | 2 (3.7) | 1 (1.6) | |||
2 | 8 (6.8) | 2 (3.7) | 6 (9.4) | |||
3 | 102 (86.4) | 46 (85.2) | 56 (87.5) | |||
Number of vessels | ||||||
1 | 18 (15.3) | 9 (16.7) | 9 (14.1) | 0.085 | ||
2 | 40 (33.9) | 17 (31.5) | 23 (35.9) | |||
3 | 60 (50.8) | 28 (51.9) | 32 (50.0) | |||
IVUS (n = 101) | ||||||
MLA, mm |
3.00 |
2.96 |
3.01 |
0.826 | ||
Calcification arc | 323.50 |
321.67 |
325.63 |
0.661 | ||
Plaque load, % | 80.99 |
81.09 |
80.89 |
0.884 | ||
RA | ||||||
Rotation speed | 161.7 |
161.39 |
162.03 |
0.673 | ||
Duration of single RA, s | 5.68 |
5.41 |
5.91 |
0.102 | ||
Frequency of RA, times | 2.65 |
2.61 |
2.7 |
0.599 | ||
Maximum burr size, mm | ||||||
1.25 | 20 (16.9) | 7 (13.0) | 13 (20.3) | 0.241 | ||
1.50 | 97 (82.2) | 46 (85.2) | 51 (79.7) | |||
1.75 | 1 (0.8) | 1 (1.9) | 0 (0) | |||
Cutting balloon | 9 (7.6) | 3 (5.6) | 6 (9.4) | 0.506 | ||
Number of stents | 1.58 |
1.48 |
1.67 |
0.198 | ||
Angio-FFR | 0.92 |
0.94 |
0.90 |
|||
Angio-IMR | 25.58 |
32.45 |
19.71 |
Values are mean
Fifty-four patients (45.8%) exhibited CMD by angio-IMR
Supplementary Table 1 shows the univariable and multivariable binary
logistic regression analyses used to predict CMD. By univariable logistic
regression analysis, variables with p
The median duration of follow-up was 21.7 months (IQR: 16.1–24.0 months), and
completed follow-up was obtained in all 118 patients. The follow-up results are
presented in Supplementary Table 2. There were 33 MACEs, including 11
all-cause deaths, seven cardiac deaths, six non-fatal myocardial infarctions, 15
TVRs, and one stroke. In the Kaplan-Meier analysis, patients with angio-IMR
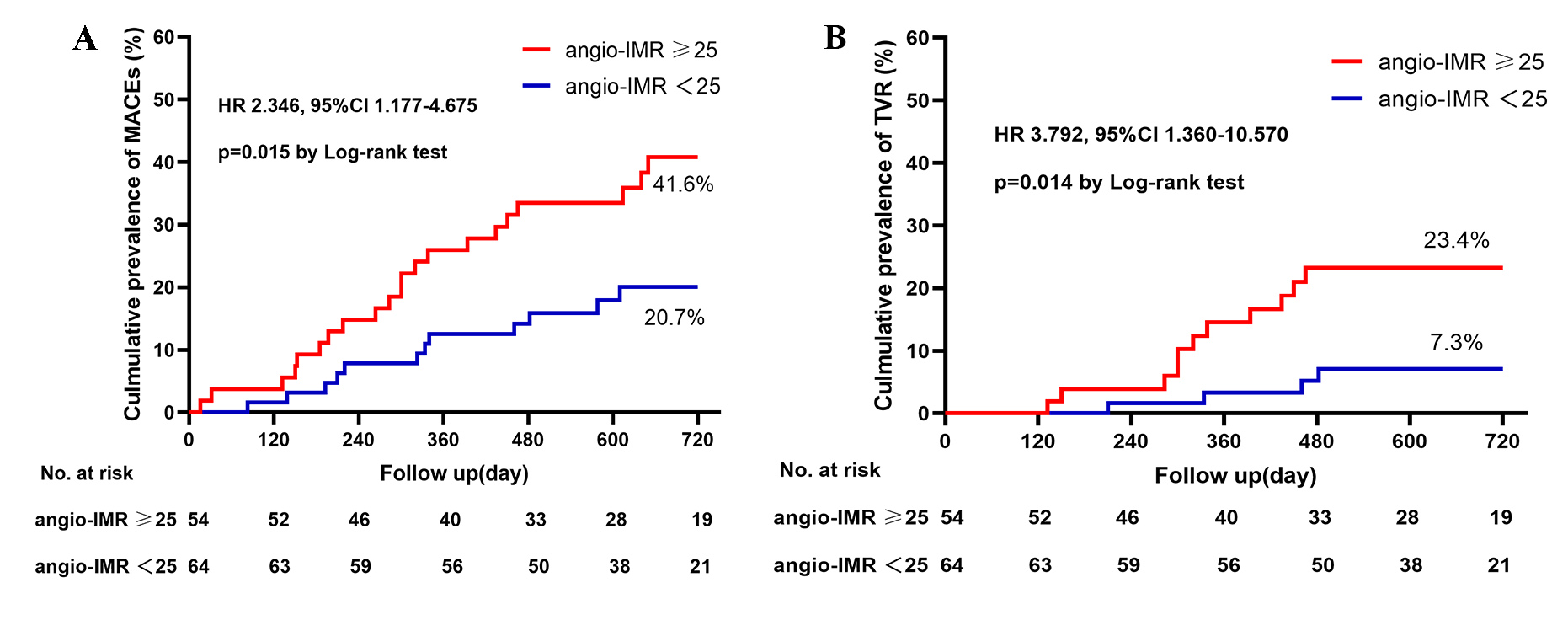
Cumulative prevalence of MACEs (A) and TVR (B) in patients with rotational atherectomy stratified by angio-IMR. MACE is a composite of cardiac death, non-fatal myocardial infarction, TVR, and stroke. Abbreviations: MACEs, major adverse cardiovascular event; TVR, target vessel revascularization; Angio-IMR, coronary angiography-derived index of microcirculatory resistance; HR, hazard ratio.
In the univariate and multivariate COX regression analyses for predictors of
MACEs, cardiovascular risk factors (sex, age, hypertension, diabetes mellitus,
hyperlipidemia, smoking, previous PCI), coronary physiological indices (diameter
of stenosis, left main stem lesion, triple vessel lesion), and coronary
procedural parameters (angle of calcification, MLA, plaque load, single spin
duration, and number) were first studied by univariate analysis. The variables
with p
Variables | Univariate | Multivariate | ||||
---|---|---|---|---|---|---|
p-value | HR | 95% CI | p-value | HR | 95% CI | |
Male | 0.099 | 2.003 | 0.887–4.573 | |||
LVEF, % | 0.041 | 0.969 | 0.940–0.999 | 0.039 | 0.969 | 0.941–0.998 |
Triple-vessel disease | 0.023 | 2.232 | 1.116–4.456 | |||
Diameter stenosis, % | 0.050 | 1.049 | 1.000–1.100 | |||
Plaque load, % | 0.051 | 1.047 | 1.000–1.096 | |||
MLA, mm |
0.040 | 0.739 | 0.554–0.986 | |||
Time of RA, s | 0.061 | 0.561 | 0.307–1.027 | |||
Frequency of RA, times | 0.077 | 0.610 | 0.353–1.056 | |||
Angio-IMR |
0.010 | 2.450 | 1.240–4.839 | 0.015 | 2.367 | 1.185–4.729 |
Abbreviations: HR, hazard ratio; CI, confidence interval; MACEs, major adverse cardiovascular events; RA, rotational atherectomy; LVEF, left ventricular ejection fraction; Angio-IMR, coronary angiography-derived index of microcirculatory resistance; MLA, Minimum luminal area.
Variables | Univariate | Multivariate | ||||
---|---|---|---|---|---|---|
p-value | HR | 95% CI | p-value | HR | 95% CI | |
Hypertension | 0.098 | 0.432 | 0.160–1.168 | |||
Angio-IMR |
0.022 | 3.373 | 1.188–9.580 | 0.035 | 3.166 | 1.083–9.255 |
Calcification arc | 0.073 | 1.012 | 0.999–1.026 | |||
Plaque load, % | 0.077 | 1.062 | 0.993–1.136 | |||
MLA, mm |
0.006 | 0.565 | 0.376–0.849 | 0.013 | 0.421 | 0.213–0.831 |
Abbreviations: HR, hazard ratio; CI, confidence interval; TVR, target vessel revascularization; Angio-IMR, coronary angiography-derived index of microcirculatory resistance; MLA, Minimum luminal area.
To the best of our knowledge, the present study is the first to focus on the
microcirculatory characteristics and prognostic implications after RA in patients
with CAD according to angio-IMR. The main findings of the current study are: (1)
The incidence of CMD in patients with CAD assessed by angio-IMR measured
immediately after the RA procedure was 45.8%. (2) Angio-FFR was independently
associated with the occurrence of CMD. (3) CAD patients undergoing RA with
angio-IMR
Coronary revascularization can restore epicardial vessel blood flow perfusion, but this may not be equivalent to increased myocardial blood flow perfusion. Myocardial perfusion is supplied by the epicardial macrovascular system in addition to the much larger microvascular system. It is well known that patients with a combined CMD have a worse prognosis [5]. There are several methods for evaluating the microcirculatory system, and noninvasive assessment techniques such as positron emission tomography and IMR are underutilized. In contrast, among the invasive assessment tools, wire-based IMR and coronary flow reserve (CFR) are the most reliable indicators for evaluating microcirculation [9], with CFR being relatively affected by hemodynamic and heart rate changes, while IMR is highly reproducible and specific for the microcirculation [17, 18]. Previous studies have shown that microcirculatory dysfunction assessed by IMR is independently associated with adverse outcomes in various cardiovascular diseases [19, 20, 21, 22]. However, the invasiveness of physiological assessments limits their application. The angio-IMR, a reliable alternative to wire-derived IMR, was developed based on the principles of computational flow and pressure dynamics, and has greatly simplified the assessment of IMR. Angio-IMR not only showed a high correlation with wire-derived IMR but also have prognostic implications [23, 24].
Studies have shown that 30.8% of patients with elective DES implantation have moderate to severe coronary calcification and that severe calcification is associated with an increased incidence of MACE resulting in adverse patient prognosis [25]. Severe coronary artery calcification can compromise coronary interventions by increasing the incidence of stent delivery failure, impairing stent expansion, and even causing dilatation balloon rupture [26]. More tools are now available to tackle severe coronary artery calcification, such as cutting balloons, intravascular lithotripsy, orbital atherectomy, and laser, but RA remains the predominant technique to treat calcified plaques [27, 28]. The plaque modification role of RA can effectively reduce calcification and fibrous plaque volume, broaden the lumen, and enhance stent apposition [4, 29, 30]. In addition, plaque modification by RA has been shown to lower the incidence of stent restenosis and malposition [31, 32]. Nevertheless, TVR and target vessel failure (TVF) rates remain high in patients with severe coronary calcium lesions; hence, the need to explore the association between RA procedures for severe coronary calcification and microvascular function.
In our study, the incidence of CMD after RA was 45.8%, traditional cardiovascular risk factors are independent of CMD. In a 120-patient study of CFR after chronic total occlusions (CTO), CMD was diagnosed in 46% of patients [33]; A multicenter, prospective study by Kobayashi et al. [34] reported that 59.1% of patients with suspected myocardial ischemia had no CMD and 40.9% of patients had CMD in single or multiple vessels. Univariate and multivariate logistic regression analysis showed that clinical factors and coronary severity did not predict CMD [34]. This is similar to our findings in that the incidence of CMD in patients with severe coronary artery calcification assessed immediately after RA was consistent with that in patients with CTO and slightly higher than that in patients with stable suspected ischemia, and that clinical factors and RA procedural parameters were not predictive of CMD. However, the angio-FFR was independently associated with the occurrence of CMD, an increased angio-FFR increased the risk of CMD, which suggested a link between coronary blood flow and microvascular function after RA. The exact mechanism of this is currently unclear and needs to be confirmed by further studies.
The prognostic significance of IMR after PCI for stable CAD has been
demonstrated [19]. Recent studies have shown that angio-IMR can predict the
outcome of patients with acute myocardial infarction and after PCI [24, 35]. In
the present study, we found significantly higher MACEs in patients with angio-IMR
In our study, angio-IMR
This study has important predictive implications for patients with severely calcified coronary artery lesions. Interventionalists now have more tools to deal with various complicated coronary lesions. However, while anatomic obstruction is relieved, the recovery of CMD is essential. Angio-IMR is a simple and convenient index to assess the state of microcirculation after RA, and we have found that elevated angio-IMR increases the risk of MACEs; therefore, we can use the strategies guided by angio-IMR to improve the prognosis of patients with microcirculatory dysfunction. Studies have shown that statins, angiotension converting enzyme inhibitors, beta-blockers and anti-anginal treatments such as nicorandil and trimetazidine help to improve the prognosis of patients with CMD [9]. However, to date, there are no specific treatment strategies for CMD that have been validated in scale randomised clinical trials, and therefore treatment of patients with CMD should be targeted at risk factors. We will also further explore the role of angio-IMR in guiding the treatment of microcirculatory dysfunction in the future.
This study has several study limitations. First, the study’s sample size was small, it was a retrospective study, and the findings need to be confirmed by a prospective study with a larger sample size. Second, we only analyzed the target vessels in which the RA procedure was performed and did not include reference vessels for comparison. Third, the study used angio-IMR to assess microcirculatory function, and the analysis results were affected by the contrast images. Thus, five patients (4.2%) were excluded because the images did not meet the requirements.
The incidence of CMD in patients with severe coronary calcification after the RA
procedure was 45.8%. Angio-IMR
RA, rotational atherectomy; CMD, coronary microvascular dysfunction; Angio-IMR, angiography-derived index of microcirculatory resistance; CAD, coronary artery disease; MACEs, major adverse cardiovascular events; TVR, target vessel revascularization; PCI, percutaneous coronary intervention; DES, drug-eluting stent; MAP, mean arterial pressure; MLA, minimum luminal area.
The data that support the findings of this study are available from Shanghai Tenth People’s Hospital Medical Record System but restrictions apply to the availability of these data, which were used under license for the current study, and so are not publicly available.
YWX and YZ designed the research study. BW, YG, YFZ, PJ, JH and HLL performed the research. BW and YG analyzed the data. BW wrote the manuscript. All authors contributed to editorial changes in the manuscript. All authors read and approved the final manuscript. All authors have participated sufficiently in the work and agreed to be accountable for all aspects of the work.
The study was approved by the Ethics Committee of Shanghai Tenth People’s Hospital (approval number: SHYS-IEC-5.0/22k148/P01). Because data were collected retrospectively, informed consent on the use of coronary angiography was waived given the institutional ethics regulations with regard to observational study nature.
Not applicable.
This research was funded by Clinical Research Plan of SHDC (NO. SHDC2020CR1040B).
The authors declare no conflict of interest.
Publisher’s Note: IMR Press stays neutral with regard to jurisdictional claims in published maps and institutional affiliations.