- Academic Editor
†These authors contributed equally.
Background: Ischemic stroke is the most common form of stroke and the
second most common cause of death and incapacity worldwide. Its pathogenesis and
treatment have been the focus of considerable research. In traditional Chinese
medicine, the root of Mongolian astragalus has been important in the treatment of
stroke since ancient times. Astragalus polysaccharide (APS) is a key active
ingredient of astragalus and offers therapeutic potential for conditions
affecting the neurological system, the heart, cancer, and other disorders.
However, it is not yet known how APS works to protect against ischemic stroke.
Methods: Rats were subjected to middle cerebral artery occlusion (MCAO)
to imitate localized cerebral ischemia. Each of four experimental groups (normal,
sham, MCAO, and MCAO+APS) contained 12 adult male Sprague-Dawley (SD) rats
selected randomly from a total of 48 rats. Following successful establishment of
the model, rats in the MCAO+APS group received intraperitoneal injection of APS
(50 mg/kg) once daily for 14 days, whereas all other groups received no APS. The
Bederson nerve function score and the forelimb placement test were used to detect
motor and sensory function defects, while Nissl staining was used to investigate
pathological defects in the ventroposterior thalamic nucleus (VPN).
Immunohistochemical staining and Western blot were used to evaluate the
expression of Neurogenic locus notch homolog protein 1 (Notch1), hairy and enhancer of split 1 (Hes1), phospho-nuclear
factor-
Ischemic stroke refers to cerebrovascular diseases that are caused by obstruction of cerebral artery blood flow leading to local brain tissue hypoxic-ischemic necrosis and to neurological defects. It is the second-leading cause of death and disability worldwide, with an increasing incidence [1, 2]. Neuropathological studies have shown that the ipsilateral thalamus suffers secondary injury following middle cerebral artery occlusion (MCAO), which may result in thalamic shrinkage and reduced sensorimotor function after ischemia [3]. In a prior study, we also discovered that after cerebral ischemia, ventroposterior thalamic nucleus (VPN) neurons were harmed and diminished, which may be a significant contributing cause to the inadequate recovery of neurological function in clinical stroke patients.
Astragalus is the root of the leguminous plant astragalus mongholicus, and has
long been used in Traditional Chinese medicine (TCM). It is the principal
ingredient in the Buyang Huanwu decoction, a traditional TCM remedy for treating
strokes. Previous pharmacological studies have shown that astragalus
polysaccharide (APS), a key active ingredient of astragalus, can reduce the
inflammatory response and immune dysfunction caused by cerebral ischemic injury,
while also enhancing the index of immune organs and inhibiting the release of
pro-inflammatory cytokines [4, 5]. The Notch signaling axis is one of the most
crucial pathways contributing to the long-term survival of neurons. Hairy and
enhancer of split 1 (Hes1) and nuclear factor-
Specific pathogen free (SPF) healthy male Sprague-Dawley (SD) rats (n = 48) with a body weight of 200
The MCAO model was established using the suture-occluded method [10].
Intraperitoneal injection with 1.5% pentobarbital sodium (2 mL/kg, AM00469,
Beijing Chemical Reagent Company, Beijing, China) was first used to anesthetize
rats. They were then fixed in a supine position on the operating table under a
shadowless lamp and the neck skin was disinfected with iodophor. The layers of
tissue were separated to expose the right common carotid artery (CCA) and a glass
minute hand was used to carefully separate the vagus nerve and CCA. The
separation was continued to the bifurcation of the internal carotid artery (ICA)
and external carotid artery (ECA), the CCA and distal ECA were ligated, and a
spare wire was set up at the proximal end. Ophthalmic scissors were used to make
a tiny incision in the ECA so that a threaded plug could be inserted (A2-2432,
Beijing Cinonech Co. Ltd, Beijing, China). The ECA was then cut off and the
thread was looped into the ICA through the stump of the ECA. The insertion depth
was 18.5
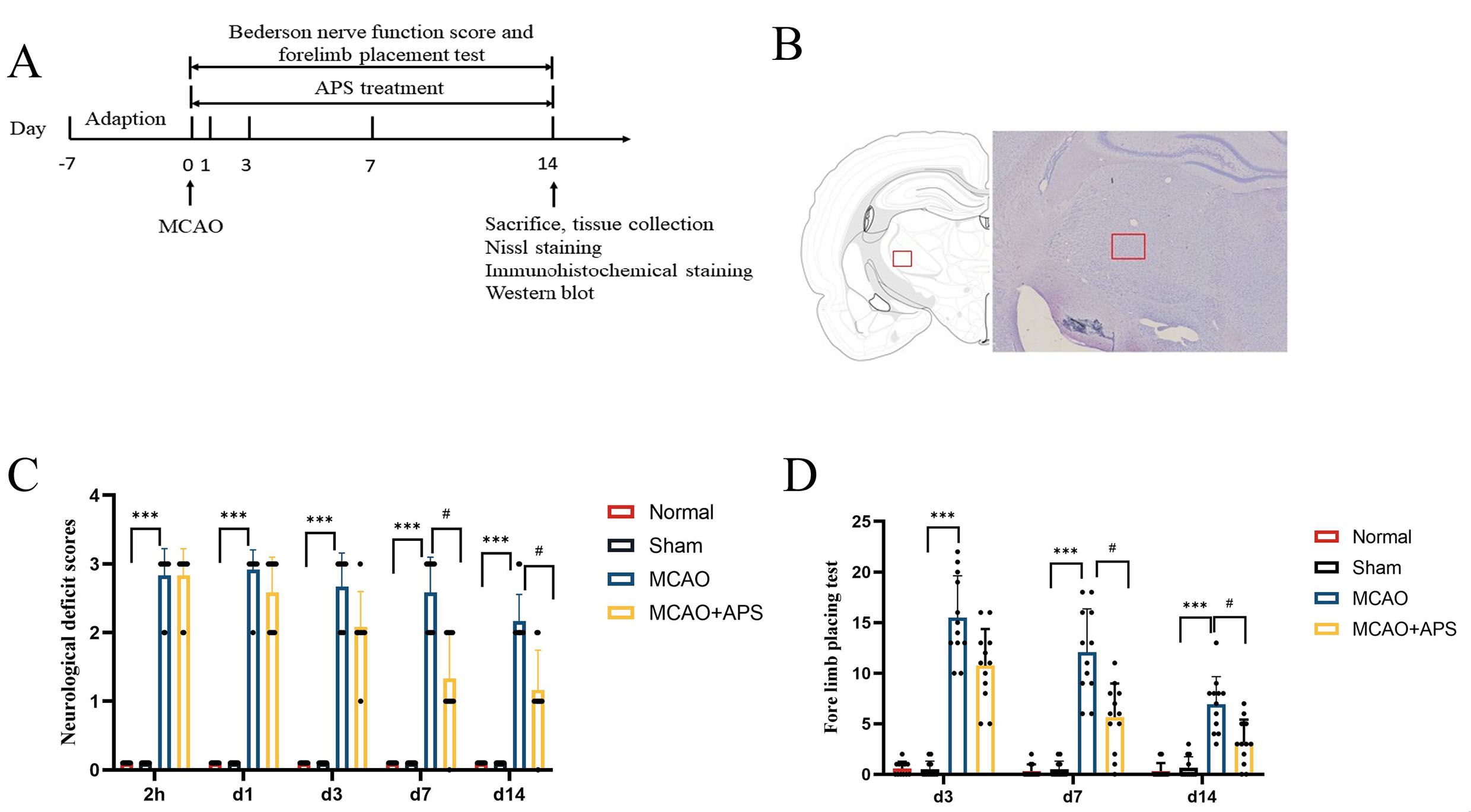
Experimental design and behavioral tests. (A) Schematic of the
study design. (B) Schematic diagram of statistical area for counting positive
cells by Nissl and immunohistochemical staining. (C) Bederson nerve function
score; n = 12 per group; ***p
Neurological impairment in rats was assessed 2 hours after surgery and on days 1, 3, 7, and 14. Neurological function was classified into four levels: 0, no neurological impairment; 1, the forepaw on the opposite side of the tail suspension test could not be fully extended; 2, the resistance of the opposite front paw to thrust decreased; 3, turned to the opposite side after placing.
The forelimb placement test was used to assess the comprehensive ability of
visual and proprioceptive perception of rats on days 3, 7, and 14. A square grid
(3 cm
Following treatment, 6 rats in each group were injected intraperitoneally with
1.5% sodium pentobarbital (3 mL/kg) and 4% paraformaldehyde was then infused
before brain extraction. Brain tissue was fixed in 4% paraformaldehyde. After
dehydration with a sucrose gradient, the tissue was embedded using optimal
cutting temperature compound (OTC) in a –20 °C frozen microtome
(HM525NX, Thermo Fisher Science, Waltham, MA, USA). It was then cut into 20
µm slices and washed three times with phosphate buffered saline (PBS) for 5 min each before use.
Filtered Nissl staining solution was then added dropwise to the tissue slices
containing the VPN, and these were placed in a 37 °C incubator for 30
minutes. Excess staining agent was washed off with double distilled water and the
sections were dehydrated, made transparent and sealed. Images were observed and
recorded at 400
Frozen sections of brain tissue containing VPN were baked in an incubator in a
60 °C incubator for 2 hours. H
The remaining 6 rats from each group were anaesthetized by intraperitoneal
injection with 1.5% pentobarbital sodium. After decapitation, the brain was
removed and placed on an ice box. According to a Stereotaxic map of rat brain
described by Paxinos et al. [13], a surgical blade was used on an ice
box to cut 2.16 and 3.84 mm behind the anterior fontanel of the brain tissue to
collect contains VPN area of the brain tissue, and then the VPN was extracted
with microscopic tweezers as described in Fig. 1B. Proteins from the VPN were
extracted using radio immunoprecipitation assay (RIPA) lysis buffer,
phenylmethanesulfonyl fluoride (PMSF) and phosphatase inhibitors. The sample
protein (40 ug) was run for two hours on an sodium dodecyl sulfate polyacrylamide
(SDS-PAGE) gel electrophoresis according to the molecular weight of the target
protein. Proteins were then transferred to a polyvinylidene fluoride (PVDF)
membrane using a continuous flow of 300 mA for 1.5 hours. The membranes were
blocked for two hours before incubation overnight at 4 °C with primary
antibodies against Notch1, Hes1, p-NF
SPSS 26.0 software (IBM Corp., Chicago, IL, USA) was used for statistical
analysis of the data, and GraphPadPrism 9.0 (Dotmatics, Boston, MA, USA) for the
creation of images. The measurement data were evaluated by a normality test and
by a homogeneity test of variance. Data with a normal distribution were reported
as the mean
The Bederson nerve function score revealed that rats in the normal group and in
the sham operation group had no neurological function defects (Fig. 1C). In
contrast, the MCAO group had severe neurological damage and considerably greater
neurological impairment scores than those of the sham group at 2 hours after
cerebral ischemia and at days 1, 3, 7, and 14 (p
The forelimb placement test showed that rats in the normal and sham operation
groups would grasp the grid line tightly when crawling, and almost never fall
into the grid (Fig. 1D). Rats in the MCAO group showed a significantly higher
number of hemiplegic forelimb falls into the grid on days 3, 7 and 14 compared to
the sham group (p
Nissl staining revealed that neurons in the VPN of rats in the normal and sham
groups showed a complete morphology, structure, and quantity, with clear outline,
centered nuclei, and complete Nissl bodies (Fig. 2A,B). However, in the MCAO
group, the volume of neurons in the affected side of the thalamus was smaller,
the edge of the cell membrane was blurred, and both necrolysis and nuclear
pyknosis were observed. Furthermore, the number of Nissl-positive neurons was
significantly lower in the MCAO group than in the sham group (p
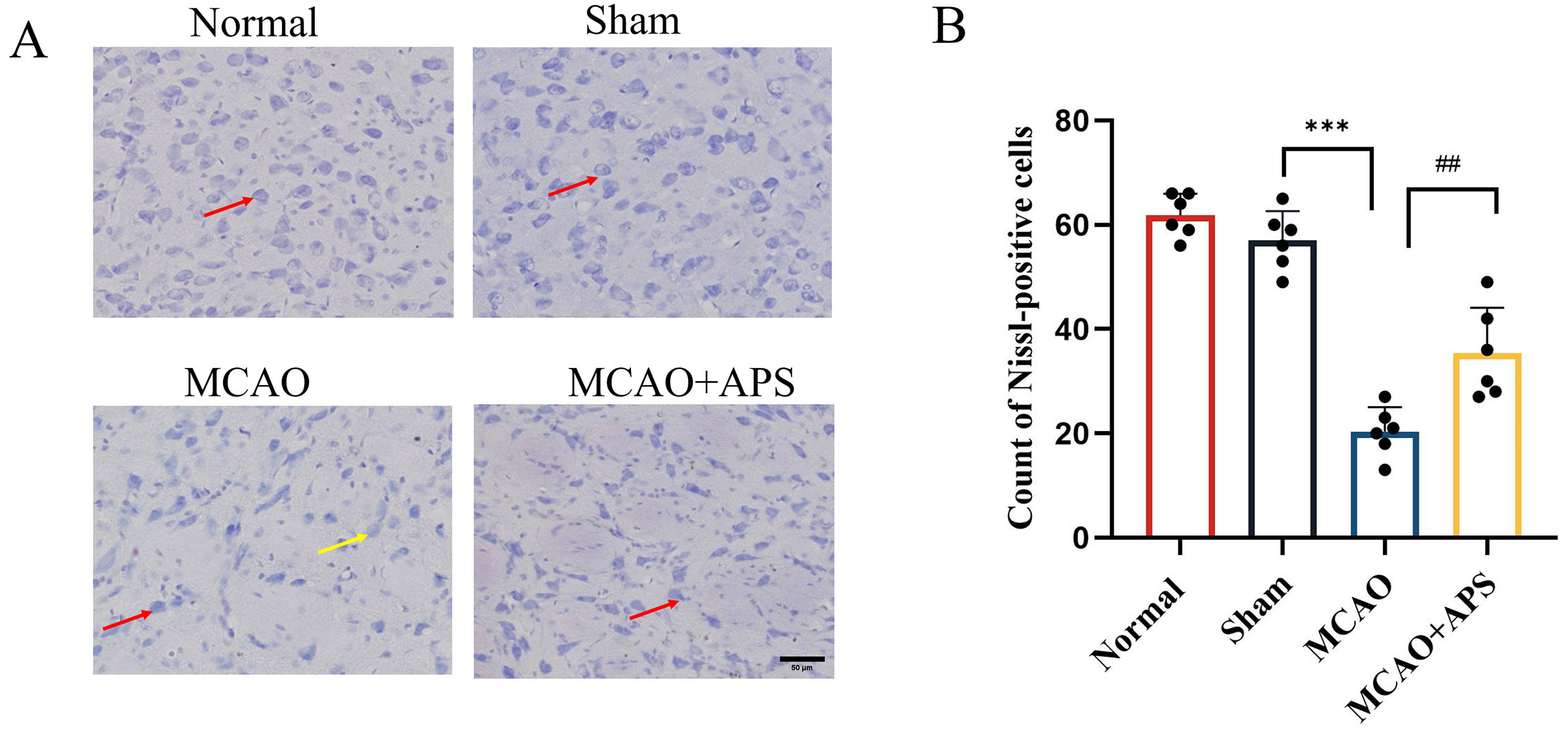
Effect of APS on histopathological changes to ventroposterior
thalamic nucleus (VPN). (A) Representative Nissl staining images of each group.
(400
Immunohistochemistry was used to investigate the expression of Notch1, Hes1, and
p-NF
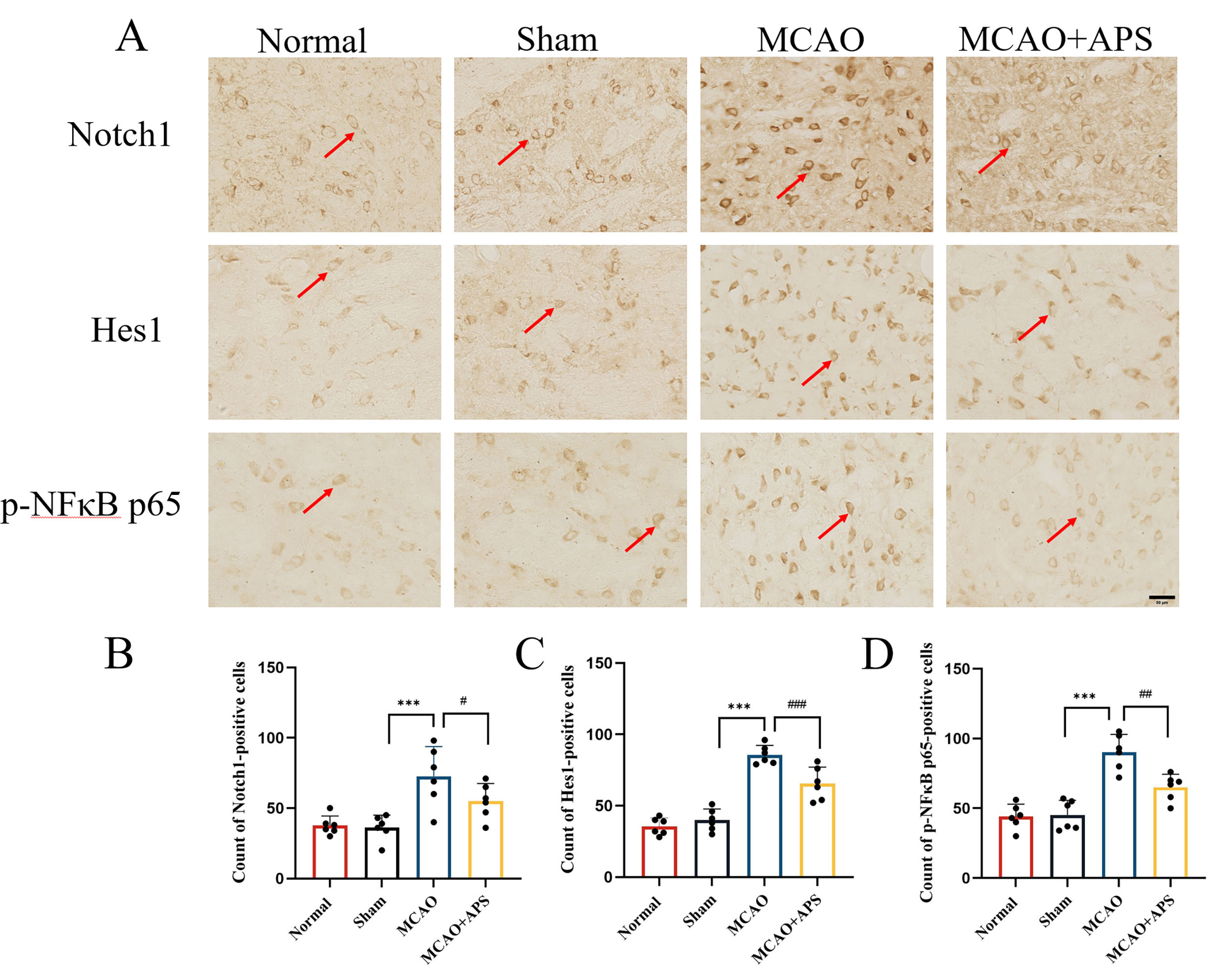
Immunohistochemistry was used to investigate the effect of APS
on the expression of Notch1, Hes1, and p-NF
The results obtained with Western blot analysis mirrored those from
immunohistochemistry (Fig. 4). The relative expression levels of Notch1 and Hes1
in the VPN were significantly higher in the MCAO group than in the sham group
(p
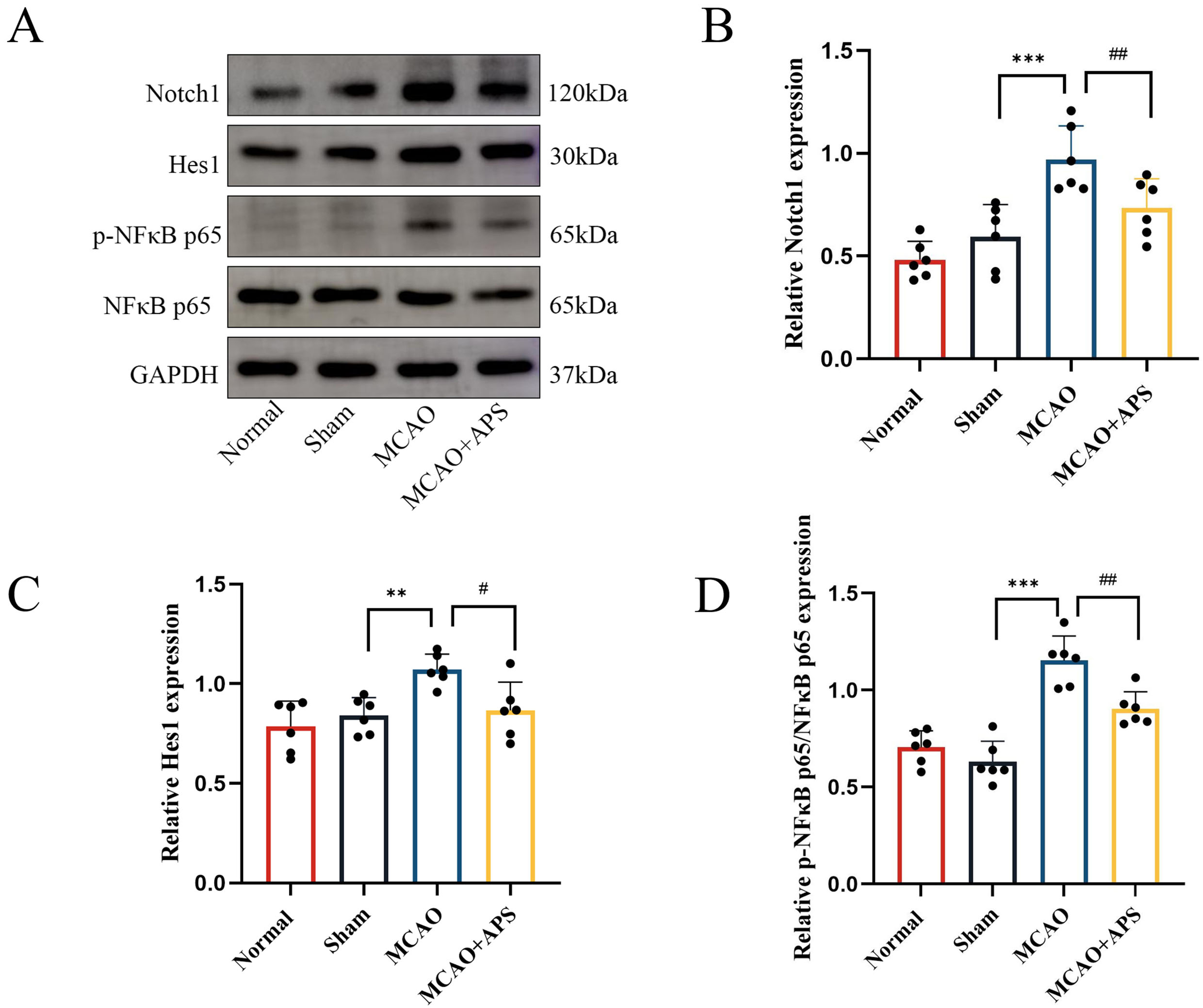
Western blotting was used to determine the effect of APS on the
expression of Notch1, Hes1, and p-NF
The incidence of stroke continues to increase [14]. However, therapeutic options for cerebral ischemia are very limited, and the recovery of neurological function in clinical stroke patients is often not ideal [15]. The search for effective treatment methods and drugs for ischemic stroke has therefore been a popular area of research. The MCAO model used in this study was demonstrated to be successful by the increased nerve defect score observed in these rats. Following APS treatment, the neurological deficit function score in the MCAO+APS group of rats decreased. Moreover, they experienced less falls of hemiplegic forelimbs into the grid, suggesting that APS can promote early recovery of sensorimotor function in rats. A previous study using a rat model of MCAO showed that APS could inhibit neuroimmune disorders and inflammation induced by cerebral ischemic injury, reduce the extent of edema in brain cells, and play a protective role in brain tissue [12]. This is consistent with the findings of the present study.
According to the literature and to previous laboratory studies, it is important to treat secondary damage in the thalamus following cerebral infarction. The thalamus is the sensory center under the cortex. In rat models of MCAO, secondary damage to the thalamus leads to cognitive dysfunction and sensory defects after focal cerebral infarction, with the damage occurring in the VPN [3, 16, 17, 18]. Nissl staining in the present study showed damage to the morphological structure of neurons in the VPN region of the MCAO group, as well as a reduced number of Nissl-positive neurons. However, the morphological structure of cells in the ischemic region of the MCAO+APS group showed significant improvement compared to the MCAO group, together with an increased number of Nissl-positive neurons. These results suggest that APS could help to attenuate the damage to neurons caused by cerebral ischemia.
Inflammation occurs during the entire process of ischemic stroke, and the
pathogenic mechanism of ischemic stroke injury is extremely complex [19]. The
generation of free radicals and the resulting oxidative stress that occurs during
ischemic stroke can cause brain damage and activation of an inflammatory
response. Both the Notch signaling pathway and NF
APS can promote the recovery of motor and sensory functions and attenuate
secondary thalamic injury in rats with cerebral ischemia. This effect may be
linked to the inhibition of Notch1/NF
The datasets used and analyzed during the current study are available from the corresponding author on reasonable request.
HL, HM, and WL conceived and designed the experiment. WL and ZN conducted the experiments and analyzed the data. WL drew up the first draft. FW and HM analyzed the data and revised the manuscript. HL gave final approval to the upcoming release. All authors contributed to editorial changes in the manuscript. All authors read and approved the final manuscript. All authors have participated sufficiently in the work and agreed to be accountable for all aspects of the work.
The animal studies were reviewed and approved by the Laboratory Animal Welfare and Ethics Committee of Wannan Medical College (No.WNMC-AME-2023134).
Not applicable.
This work was supported by the Research Fund Project of Wannan Medical College (No.WK2022XS45), the Collegiate Major Natural Science Research Projects of Anhui Province (No.KJ2020A0603) and the Teaching and Research Project of Anhui Provincial Department of Education (2019jxtd072).
The authors declare no conflict of interest.
Publisher’s Note: IMR Press stays neutral with regard to jurisdictional claims in published maps and institutional affiliations.