- Academic Editor
Pyroptosis is a type of regulated cell death that relies on caspases, vesicles,
and the cleavage of gasdermin proteins (which create pores in the cell membrane).
The nucleotide-binding oligomerization domain-like receptor protein 3
(NLRP3) inflammasome, which is involved in this process, is the most
widely studied inflammasome. Caspase-1 activates pro-inflammatory cytokines, such
as IL-1
Alzheimer’s disease (AD) is a disorder that causes people to gradually lose their memory and cognition. The pathophysiological process of AD is thought to begin years, or even decades, before symptoms arise [1]. Despite decades of research, our knowledge of AD pathogenesis remains unclear, and our ability to intervene via the prevention or treatment of dementia is still limited. There is still much we don’t know about the molecular mechanisms that lead to AD, and this is important because it would help us develop more sensitive diagnostic markers and find new ways to treat the disease.
Pyroptosis is an inflammatory form of regulated cell death and a critical and
necessary host-defense immune mechanism. Increasing evidence shows that
dysfunctional pyroptosis participates in neurological disorders such as
Parkinson’s disease [2], amyotrophic lateral sclerosis [3], and Huntington’s
disease [4]. There are emerging evidences that the inflammatory responses in the
central nervous system (CNS) may be a major cause and common feature of AD [5, 6]. Pyroptosis is also involved in
There are two main ways for cell death to occur: ordered (programmed-like, regulated) and nonordered (necrosis). To date, over 20 forms of regulated cell death have been identified and studied, including apoptosis, autophagy or autophagic death, pyroptosis, and ferroptosis, but they are not all equally well characterized. Studies have shown that substrates, rather than their upstream proteases, determine the nature of cell death. Because gasdermin family members are indispensable executors of pyroptosis, pyroptosis is also known as gasdermin-mediated regulated cell death [8, 9].
Pyroptotic cells display DNA fragmentation, which can be detected by terminal deoxynucleotidyl transferase dUTP nick-end labeling, but at a lower intensity than apoptotic cells. Chromatin condensation also occurs in pyroptosis, but the nucleus remains intact. Furthermore, pyroptotic cells become annexin-V-positive because, in early membrane rupture, the inner leaflet of membrane is exposed to the outside [10, 11]. During pyroptosis, the cell membrane breaks down to create small holes with a diameter of 11–24 nm; this causes increased cell permeability and the release of inflammatory cytokines, lactate dehydrogenase, and other intracellular substances. The small pores in the cell membrane cause the cell to lose its salt and water balance, and this causes it to swell. Finally, the cell membrane is destroyed, and the cellular contents are released into the extracellular environment. This causes the body’s immune system to become active, drawing in more inflammatory cells, and inducing a serious inflammatory reaction [12, 13]. Unlike necrosis, pyroptotic cell death and the consequent inflammatory responses are reversible and controllable. Thus, pyroptosis has attracted increasing attention in the study of infectious diseases, various neoplastic diseases and metabolic diseases, and represents a new research direction.
Members of the gasdermin family have been recently identified as having
pore-forming activity and are found in many different cells and tissues.
Currently, this family comprises six homologous genes in humans: gasdermin A
(GSDMA); GSDMB; GSDMC; GSDMD; GSDME;
and pejvakin. All gasdermins except for pejvakin contain a cytotoxic N-terminal
(GSDM
Pore formation by the gasdermin family is a characteristic of pyroptosis [16, 17], the binding of the GSDM
At present, the mechanism by which GSDMD induces pore formation in the
membrane (which constitutes cell pyroptosis) is relatively clear, but it is still
a matter of debate how caspases recognize and cleave GSDMD. In studies
on apoptosis, caspases have been shown to activate the substrate protein by
recognizing a tetrapeptide sequence in the substrate and cleaving after the
aspartate residue [22, 23]. However, this process is different in pyroptosis.
Recently, it was found that autocleavage at the Asp289/Asp285 location in
caspase-4/11 creates p10. To carry out a tetrapeptide sequence-independent
cleavage, the p10-form promotes the binding of caspase-4/11 to the
GSDMD
The canonical pyroptosis pathway is mediated by caspase-1. Proteins called
pattern recognition receptors (PRRs) assist the body in recognizing two groups of
substances: damage-associated molecular patterns (DAMPs), which are linked to
cell components, and pathogen-associated molecular patterns (PAMPs), which are
linked to microbial infections. Inflammatory signaling cascades are thereby
initiated. Several families of PRRs, including NOD-like receptors (NLRs), toll-like receptors (TLRs),
and RIG-I-like receptors, play vital roles in the immune system [25]. The
inflammasome is activated by various stimuli, which induces the activation of
caspase-1. On the one hand, GSDMD which is cleaved and activated by
activate caspase-1 causes cell membrane to form pores, and lead to pyroptosis; on
the other hand, caspase-1 can rapidly process pro-interleukin-1
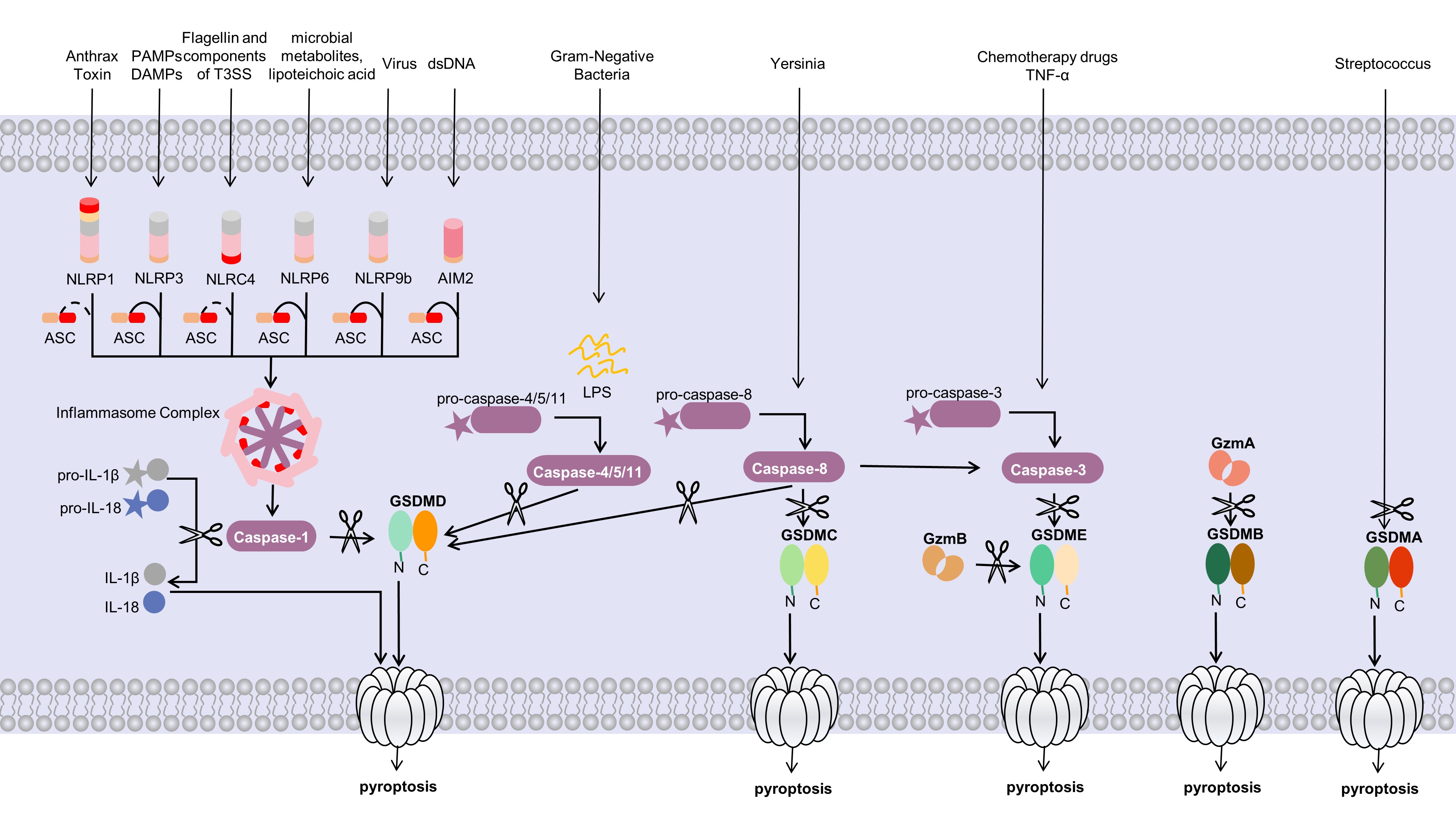
Molecular mechanisms of pyroptosis. The canonical pyroptosis
pathway depends on the inflammasome and GSDMD by caspase-1. Activated
inflammasome promotes the activation of caspase-1, which cleaves the pore-forming
factor GSDMD. Active caspase-1 also cleaves the proinflammatory
cytokines such as IL-1
A collection of multiprotein signaling complexes called inflammasomes are found
in the cytoplasm. Several inflammasomes, including NLRP1,
NLRP2, NLRP3, AIM2, and NLRC4, have been
discovered [27]. The immune receptor protein NLRP3, adaptor protein ASC
(apoptosis associated speck-like protein containing a caspase recruitment domain), and inflammatory
protease caspase-1 make up the most extensively studied inflammasome, which
reacts to microbial infections, endogenous danger signals, metabolic risk
factors, and environmental stimuli [28, 29]. An earlier investigation revealed
that the gene expression of NLRP3 was so low that it was insufficient to
activate the assembly of an inflammasome during resting conditions. Therefore,
the canonical NLRP3 inflammasome is activated when two steps are
completed [30, 31, 32]: the first step is the priming step, through the
myd88-NF-
The noncanonical pyroptosis pathway is mediated by caspase-4/5/11. Bacterial
lipopolysaccharide stimulates and activates caspase family proteases (caspase-4/5
in humans and caspase-11 in mice), and activate caspase-4/5/11 cleaves
GSDMD, which forms pores in the cell membrane and eventually leads to
pyroptosis [14, 37]. Unlike the canonical signaling pathway, IL-1
In addition to the two different pyroptosis pathways described above, recent research has revealed some new mechanisms. GSDME is specifically cleaved by caspase-3, and thereby induces the switching of caspase-3-mediated apoptosis to pyroptosis [39]. Caspases-3/7 which are thought to play a role in apoptosis, could induce GSDMD-associated microglial pyroptosis under neuroinflammatory conditions [40]. Caspase-8 is a caspase that is involved in apoptosis and necroptosis, however, recent evidence suggests that caspase-8 plays a critical role in pyroptosis [41]. The RIPK1- and caspase-8-dependent cleavage of GSDMD results in cell death; no other caspase is involved in the whole process [42]. Additionally, the lysosomal Ragulator-Rag complex initiates caspase-8-mediated pyroptosis by Yersinia [43].
Pyroptosis has been assessed in the CNS using a variety of assays; here, we review the evidence for pyroptosis in each CNS cell type (Fig. 2; Table 1, Ref. [5, 7, 44, 45, 46, 47]).
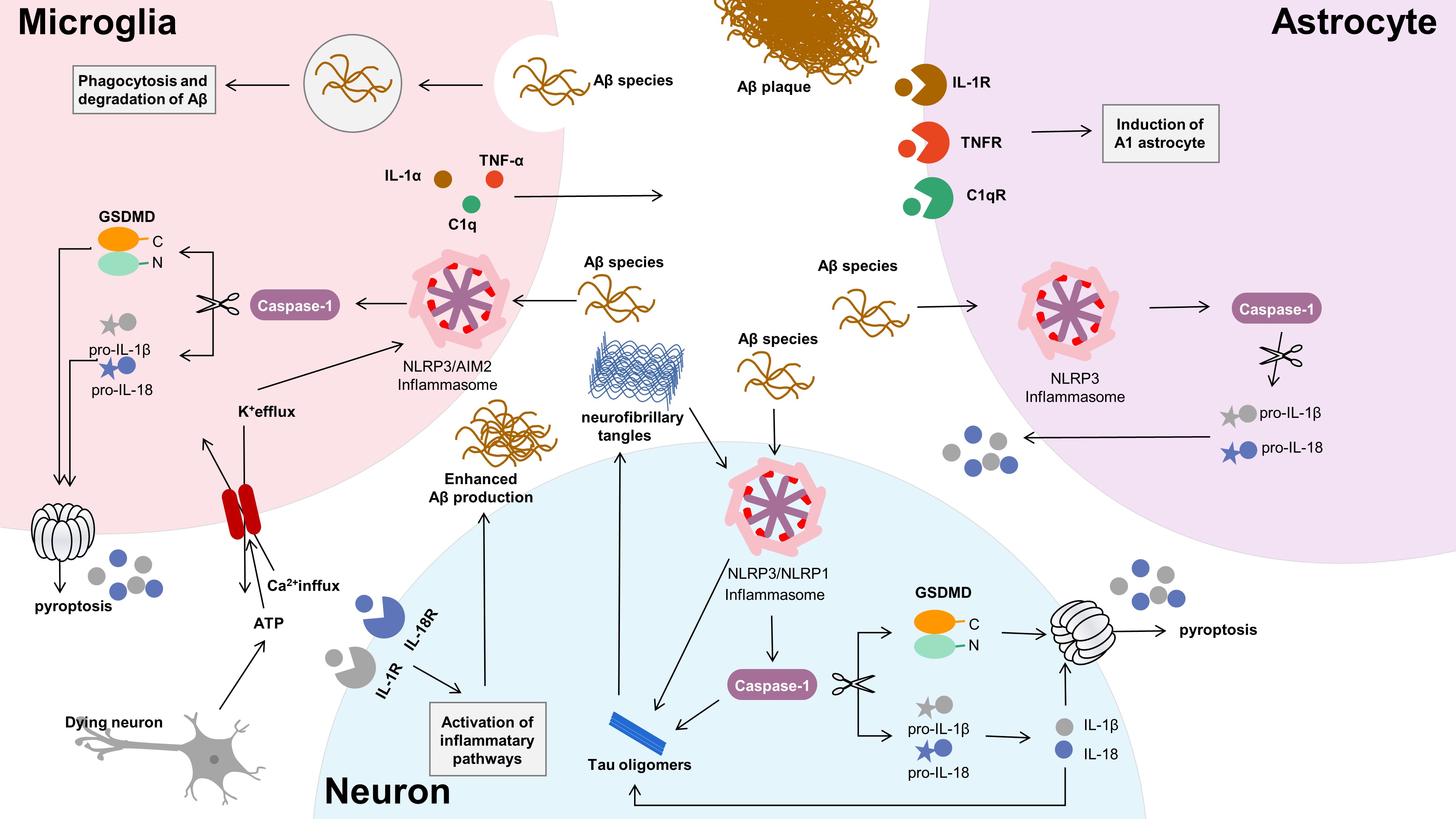
Neuron-glia crosstalk in Alzheimer’s disease. Microglia,
working as both phagocytic cells and innate immunocyte, can recognize and clear
A
Experimental model | Cellular location | Comments | Reference |
AD mice | microglia | Activation of NLRP3-caspase-1-GSDMD axis by ASC-A |
[44] |
AD mice | astrocytes | Activation of NLRP3 and caspase-1 by A |
[45] |
AD rats and PC12 cells | neurons | Activation of NLRP1-caspase-1-GSDMD axis by hyperphosphorylated tau | [7] |
AD mice | neurons | Activation of NLRP3-caspase-1-GSDMD axis by A |
[5] |
AD mice | neurons | Activation of NLRP1-caspase-1-GSDMD axis by A |
[46] |
AD mice | oligodendrocytes | Activation of NLRP3-caspase-1-GSDMD axis by A |
[47] |
Microglia is a type of resident macrophage present in the CNS. But the mechanism
by which it works in the resting state is still poorly understood. Activated
microglia can not only promote the repair of tissue damage, but also promote the
inflammatory response of the CNS. However, the effect of inflammatory microglia
on brain injury and damage is closely related to the pathogenesis of AD [6, 48].
The NLRP1, NLRP3, and AIM2 inflammasomes have been
reported to be activated in microglia, astrocytes, and neurons [49, 50, 51], and the
NLRP3 inflammasome is highly activated in microglia [52]. Activation of
the NLRP3 inflammasome triggers the release of several proinflammatory
cytokines, including IL-1
Astrocytes are the most widely distributed in the brain and play an important
role in normal central activities. Studies have shown that astrocytes play an
important role in the inflammatory response of the CNS. Together with other glial
cells (e.g., microglia, oligodendrocytes), astrocytes compose and maintain a
regulated microenvironment [59, 60]. Their activation states vary, ranging from
neuroprotective (decreases inflammatory response, promotes repair) to neurotoxic
(intensifies inflammatory response, causing neurodegeneration) [61]. A
Pyroptosis is of great significance for the pathogenesis of AD. However, most
studies have focused on glial cells, and there have been few experiments on the
interaction between neurons and pyroptosis. The latest research suggests that
pyroptosis is not restricted to glial cells; neurons are immunoreactive to
cleaved GSDMD [66]. Studies have showed that the inflammasomes of
NLRP3 and NLRP1 may induce neuroinflammatory processes by
pyroptosis in neurons. A
Oligodendrocytes (OLs) are important for the functioning of the CNS. Although there is increasing evidence that OL damage and white matter degeneration are important pathological changes in AD, their roles in the occurrence and development of AD are still unclear [68]. Zhang et al. [47] reported that mature OLs in both AD patients and AD mice undergo NLRP3-dependent GSDMD-associated inflammatory injury, accompanied by demyelination and neurodegeneration. In mature OLs, overactivation of Drp1 leads to impaired glucose metabolism, leading to NLRP3-related inflammation and pyroptosis.
AD is a neurodegenerative disorder clinically defined by gradually increasing
cognitive impairment and alterations in executive functions. The
neuropathological hallmarks of AD are the accumulation of A
Activation of the NLRP3 inflammasome by fibrillar A
Pyroptosis has been reported to act as a component in the progression of AD by
its interaction with A
With continuing research on pyroptosis, increasing evidence shows that pyroptosis can be used as a new therapeutic target for the treatment of AD (e.g., using NLRP3 inflammasome inhibitors such as MCC950 and JC-124 [78, 79] and proinflammatory caspase inhibitors such as VX765) [80, 81]. However, none of them have been applied in the clinic. At present, there is no relevant report on GSDMD inhibitors as an AD treatment strategy. However, because GSDMD is the main executor of cell pyroptosis, it is reasonable to speculate that inhibitors of GSDMD might have a place in the treatment of AD.
In recent years, Traditional Chinese Medicine (TCM) has been widely used to
treat AD, and research on the anti-pyroptotic effects of the active ingredients
of TCM preparations has gained increasing attention. Because it inhibits
NF-
Despite the substantial research effort focused on finding drugs for the treatment of AD, some therapeutics are single-target drugs, and most of these clinical trials have ended in failure. Therefore, a new approach to developing AD drugs is urgently needed. Considering the complex multifactorial etiology of AD, TCMs, which have the synergistic effects of binding multiple targets and activating multiple pathways, may be safe and ideal candidates as therapies for AD. Therefore, TCMs have broad application prospects in the treatment of AD.
All in all, Pyroptosis plays a pivotal role in the progression of AD. Our review highlights key developments in understanding pyroptosis in different CNS cells and AD pathology. Although the pyroptotic machinery has been studied in great detail, it is still a novel research topic, and there are many gaps and challenges in the regulatory mechanisms of pyroptosis during the AD process that should be investigated in future studies. In this review, we considered several critical points, including key pyroptosis-associated regulatory genes, noncoding RNAs, and even results from multiomics analyses. Perhaps pyroptosis-related molecules, such as GSDMD, can be used as biomarkers for diagnosis and prognosis. The exact pathogenetic mechanisms underlying AD remain uncertain, as there are still no drugs that can slow the progression of AD, let alone offer a cure. The exploration of pyroptosis may lead to new ways to treat AD. Overall, pyroptosis is a new perspective on the pathogenesis of AD.
AD, Alzheimer’s disease; CNS, central nervous system; A
YJ and QZ designed the review. YJ, LZ and SL collected the data. YJ and QZ wrote the manuscript. All authors contributed to editorial changes in the manuscript. All authors read and approved the final manuscript. All authors have participated sufficiently in the work and agreed to be accountable for all aspects of the work.
Not applicable.
Thanks to all the peer reviewers for their opinions and suggestions.
This research was funded by Fund of Science and Technology Development Project of Jilin Province, grant number 20200404076YY.
The authors declare no conflict of interest.
Publisher’s Note: IMR Press stays neutral with regard to jurisdictional claims in published maps and institutional affiliations.