- Academic Editors
Introduction: Some studies have
found that probiotics can improve cognitive impairment in Alzheimer’s disease,
although the specific molecular mechanism by which this occurs has not been
reported. Our previous research found that probiotics inhibited bacteria-related
Toll-like receptor 4- and retinoic-acid-inducible gene-I-mediated nuclear
factor-
The gut microbiota is comprised of more than 1000 different bacterial species [1] and plays an important role in gastrointestinal and brain functions [2]. It has been suggested the gut-brain axis is a bidirectional communication pathway because the brain can alter gut microbiota through the central nervous system, and the microbiota can regulate brain function through endocrine, immune, metabolic, and neuronal pathways [3, 4]. Accumulating evidence suggests a clear relationship between the gut microbiota and cognitive function [5, 6], thus raising the possibility that manipulation of gut microbiota may improve cognitive impairment.
Probiotics are mono- or mixed cultures of live microorganisms that are known to
confer health benefits to the host when administered in adequate amounts [7].
Research into the effects of probiotics on cognitive function is increasing
[8, 9]. Bifidobacterium breve strain A1 suppressed the
hippocampal expression of inflammation and
prevented cognitive dysfunction in a mouse model of Alzheimer’s disease (AD)
[10]. Consumption of the probiotics Lactobacillus acidophilus,
Lactobacillus casei, Bifidobacterium bifidum, and Lactobacillus
fermentum for 12 weeks positively affected cognitive function in AD patients
[9].
Furthermore,
our previous research found that the
mechanism by which probiotics improve
cognitive impairment is associated with inhibition of the bacterial-related
Toll-like receptor 4 (TLR4)- and retinoic-acid-inducible gene-I (RIG-I)-mediated
nuclear factor
Some bacterial products may cause cognitive deficits. For example,
lipopolysaccharide (LPS) levels are significantly increased in the plasma of
patients with dementia [12]. In addition to
TLRs, the main receptors associated with LPS are caspases [13].
Murine caspase-11 (the
ortholog of caspase-4 and caspase-5 in humans) is a caspase family member that
responds to LPS, thereby mediating inflammatory responses and contributing to
host defense mechanisms against Gram-negative bacteria [14, 15]. Activated
caspase-11 has been shown to interact with
caspase-1 to promote its activation and catalyze cleavage of the inactive
interlukin-1
We treated 9-month-old senescence-accelerated mouse prone-8 (SAMP8) mice, which develop neuronal loss and marked memory deficits [21, 22], for 12 weeks with ProBiotic-4 (a mixture of Lactobacillus acidophilus, Bifidobacterium bifidum, Lactobacillus casei, and Bifidobacterium lactis). ProBiotic-4 significantly reduced inflammation in the gut-brain axis and improved cognitive impairment in SAMP8 mice. The mechanism of action of ProBiotic-4 appears to be related to inhibition of the caspase-11/caspase-1 pathway and reduction of ALPK1 expression.
ProBiotic-4 was purchased from Swanson (198013, Fargo, ND, USA) and contains Lactobacillus acidophilus (12.5%), Bifidobacterium bifidum (12.5%), Lactobacillus casei (25%) and Bifidobacterium lactis (50%). The other reagents were of the highest quality available and were obtained from commercial sources.
Male 9-month-old senescence-accelerated
mouse resistant 1 (SAMR1) and SAMP8 mice were purchased from the First Teaching
Hospital of Tianjin University of Traditional Chinese Medicine (Tianjin, China).
They were housed at 22 °C
After 12 weeks of ProBiotic-4 treatment, the Morris water maze test was performed to assess spatial learning and memory ability in mice as previously described [23].
At the completion of the Morris water maze test, mouse plasma was collected for further analysis. The mice were perfused with cold normal saline via the ascending aorta, and cerebral and intestinal samples were collected for Western blotting and biochemical analyses.
The expression of ionized calcium binding adapter molecule 1 (Iba-1) and
synaptophysin (SYN) and neuron in per field of cerebral cortex or hippocampal CA1
was digitized as described previously [23, 24]. Selected brain sections were
incubated with the respective primary antibodies (Supporting Information
Supplementary Table 1) at 4 °C overnight, followed by detection
with a secondary antibody conjugated with fluorescein isothiocyanate (AR1177,
Boster, Wuhan, Hubei, China). The area and the level of immunoreactivity per
field of cerebral cortex or hippocampal CA1 was digitized
(400
To determine IL-1
Plasma IL-1
Protein samples were isolated from brain and intestinal tissue homogenates using
RIPA buffer (P0013B, Beyotime, Shanghai, China) according to the manufacturer’s
instructions. The detailed procedure for Western blot analysis was described
previously [25]. Primary antibodies against
SPSS 19.0 software (IBM Corp., Chicago, IL, USA) was used for the statistical
analysis. Data were expressed as the mean
The Morris water maze test was used to examine spatial learning and memory in
mice. In the hidden platform test (Fig. 1A), vehicle-treated
SAMP8 mice exhibited a significantly longer escape latency compared with
vehicle-treated SAMR1 controls (p
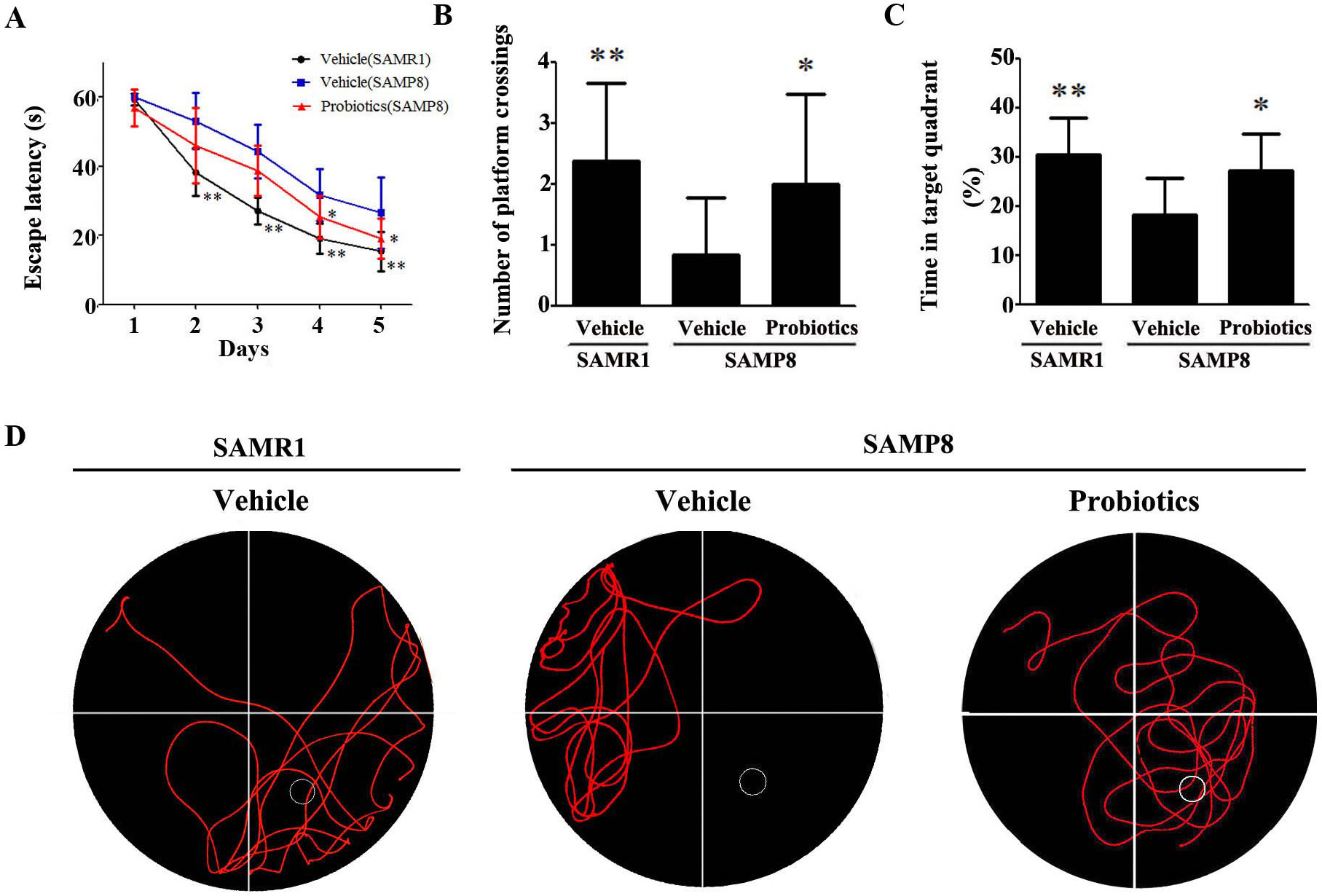
ProBiotic-4 improved the performance of SAMP8 mice in the Morris
water maze test. (A–C) Escape latency (A), number of platform crossings (B),
and percent time in the target quadrant (C). (D) Characteristic swimming trails
in the Morris water maze test. The data are expressed as mean
We examined the expression of SYN in the
hippocampus and cortex. As shown in Fig. 2A, immunostaining revealed
significantly lower SYN expression in the hippocampus and cortex of
vehicle-treated SAMP8 mice compared with vehicle-treated SAMR1 mice (p
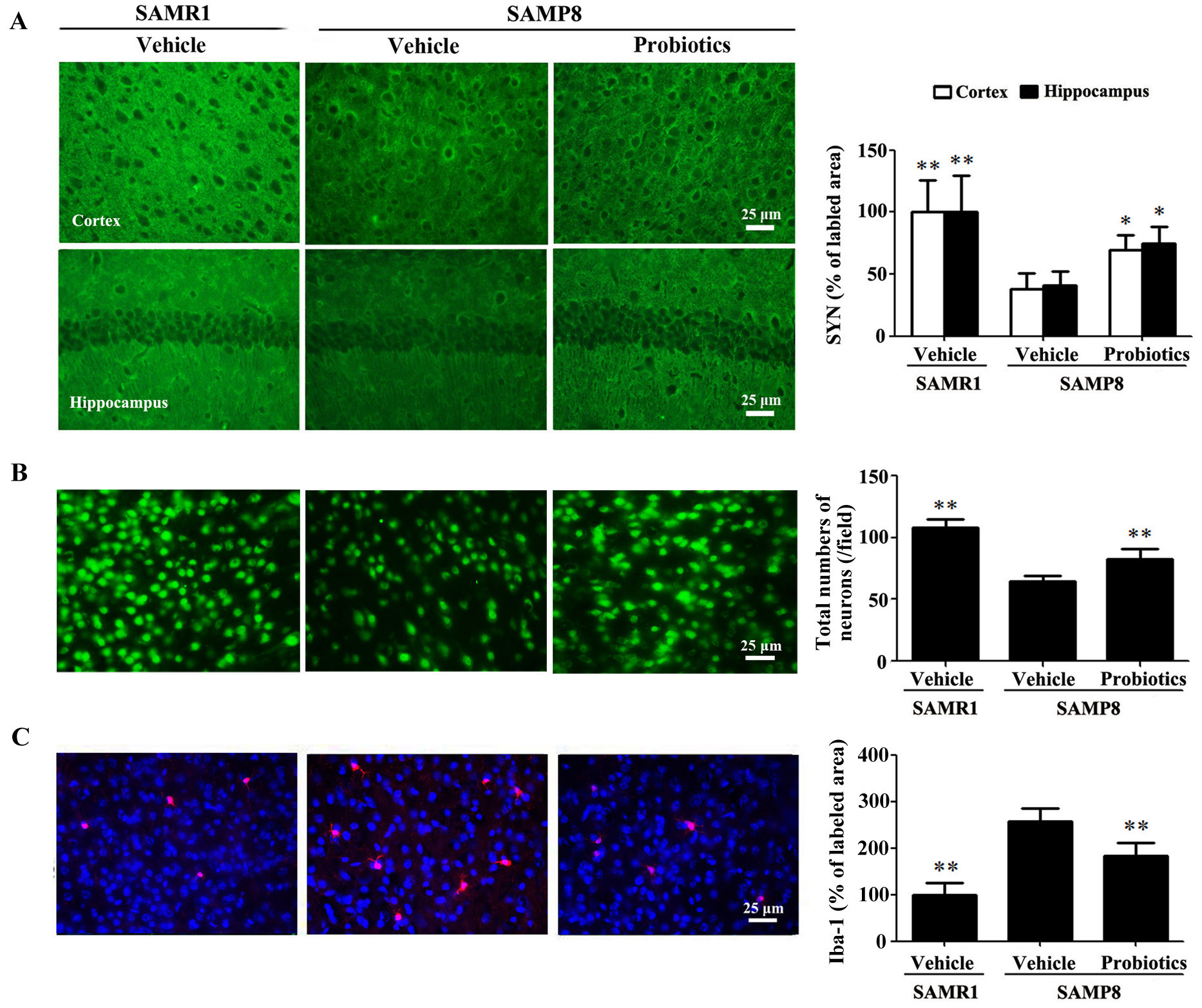
ProBiotic-4 increased synaptophysin expression, preserved
neuronal survival, and reduced Iba-1 immunoreactivity in SAMP8 mice. (A)
Representative images of SYN immunofluorescence and quantitative analysis of the
positive area of SYN expression in the cortex and hippocampal CA1 area of SAMR1
and SAMP8 mice. (B) Representative images of neuronal immunofluorescence and
quantitative analysis of the number of NeuN-positive neurons in the cortex. (C)
Representative images of Iba-1 immunofluorescence and quantitative analysis of
the positive area of Iba-1 expression in the cortex. Scale bar = 25
µm. The data are expressed as mean
Tight junction proteins play a crucial role in maintaining the integrity of the
intestinal barrier by effectively preventing harmful substances from entering the
bloodstream [26]. We evaluated the effect
of probiotics on the integrity of the intestinal barrier by examining the
expression of intestinal tight junction proteins. Western blot analysis showed
that expression of the claudin-1, occludin, and ZO-1 proteins was significantly
lower in vehicle-treated SAMP8 mice compared with vehicle-treated SAMR1 mice
(p
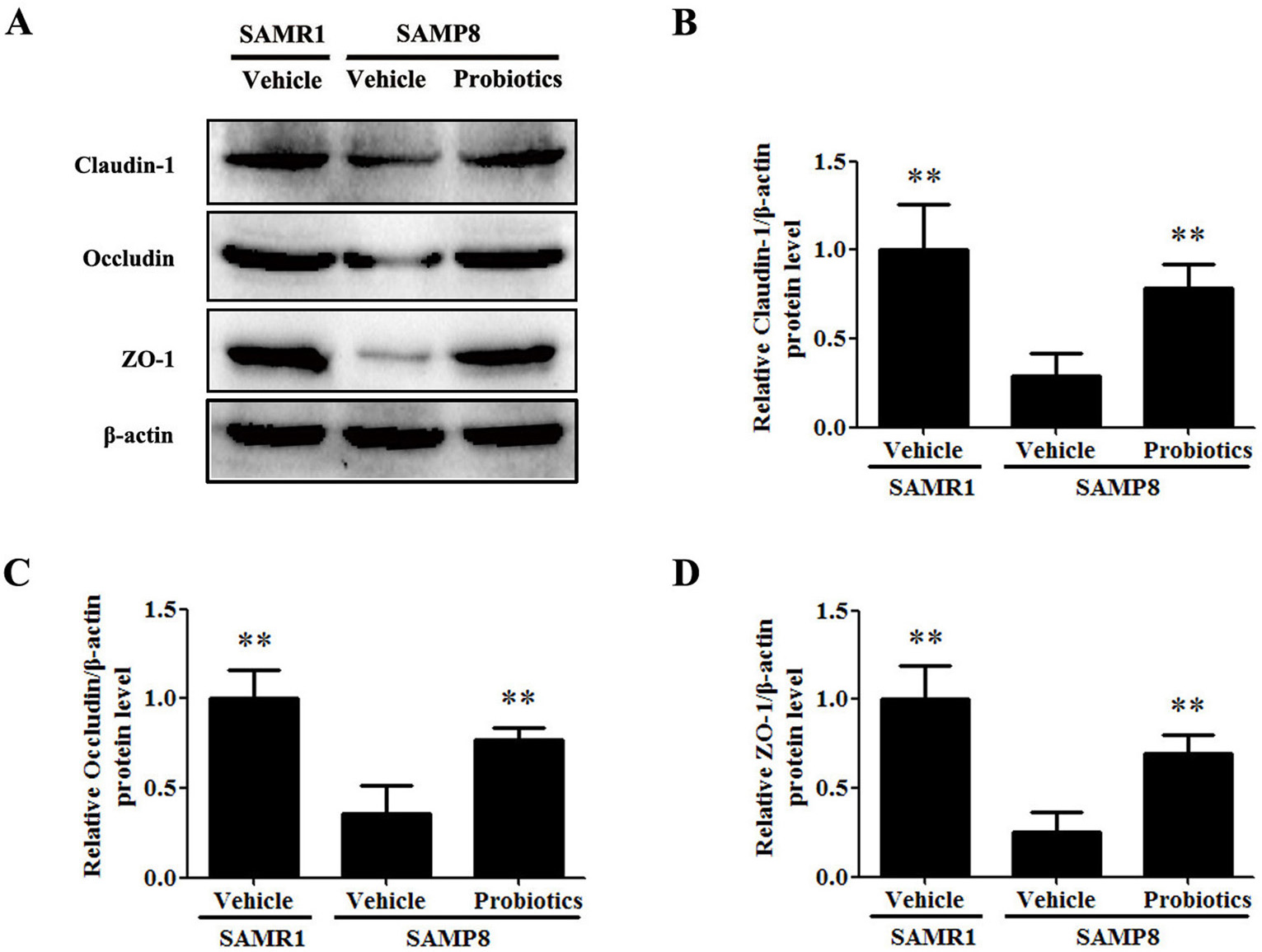
ProBiotic-4 increased the expression of intestinal tight
junction proteins in SAMP8 mice. (A) Protein expression of the intestinal tight
junction markers claudin-1, occludin, and ZO-1 as detected by Western blot
analysis in SAMR1 and SAMP8 mice. (B–D) Quantification of claudin-1, occludin,
and ZO-1 protein expression in the intestine. The data are expressed as mean
Activation of the caspase-11/caspase-1 signaling pathway was detected in
intestinal and brain tissues. As shown in Fig. 4A,B, the expression of caspase-11
and cleaved caspase-1 in the intestinal and brain tissues of vehicle-treated
SAMP8 mice were significantly higher compared with vehicle-treated SAMR1 mice
(p
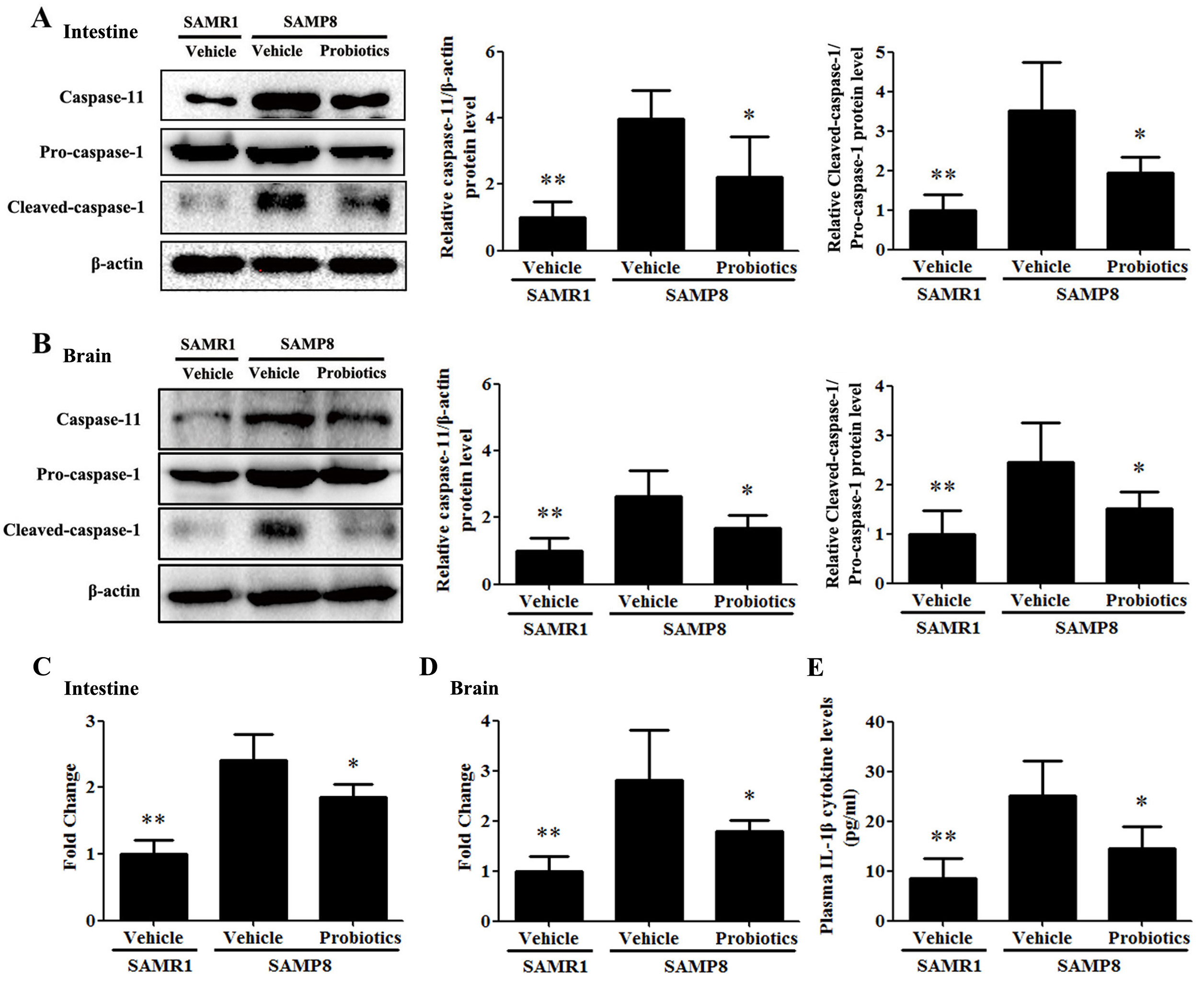
Probiotic-4 inhibited activation of the caspase-11/caspase-1
pathway in SAMP8 mice. (A,B) Representative immunoblots and quantitative
analysis of caspase-11, pro-caspase-1, and cleaved-caspase-1 expression in the
intestine and brain of SAMR1 and SAMP8 mice. (C,D) Polymerase chain reaction
analysis of IL-1
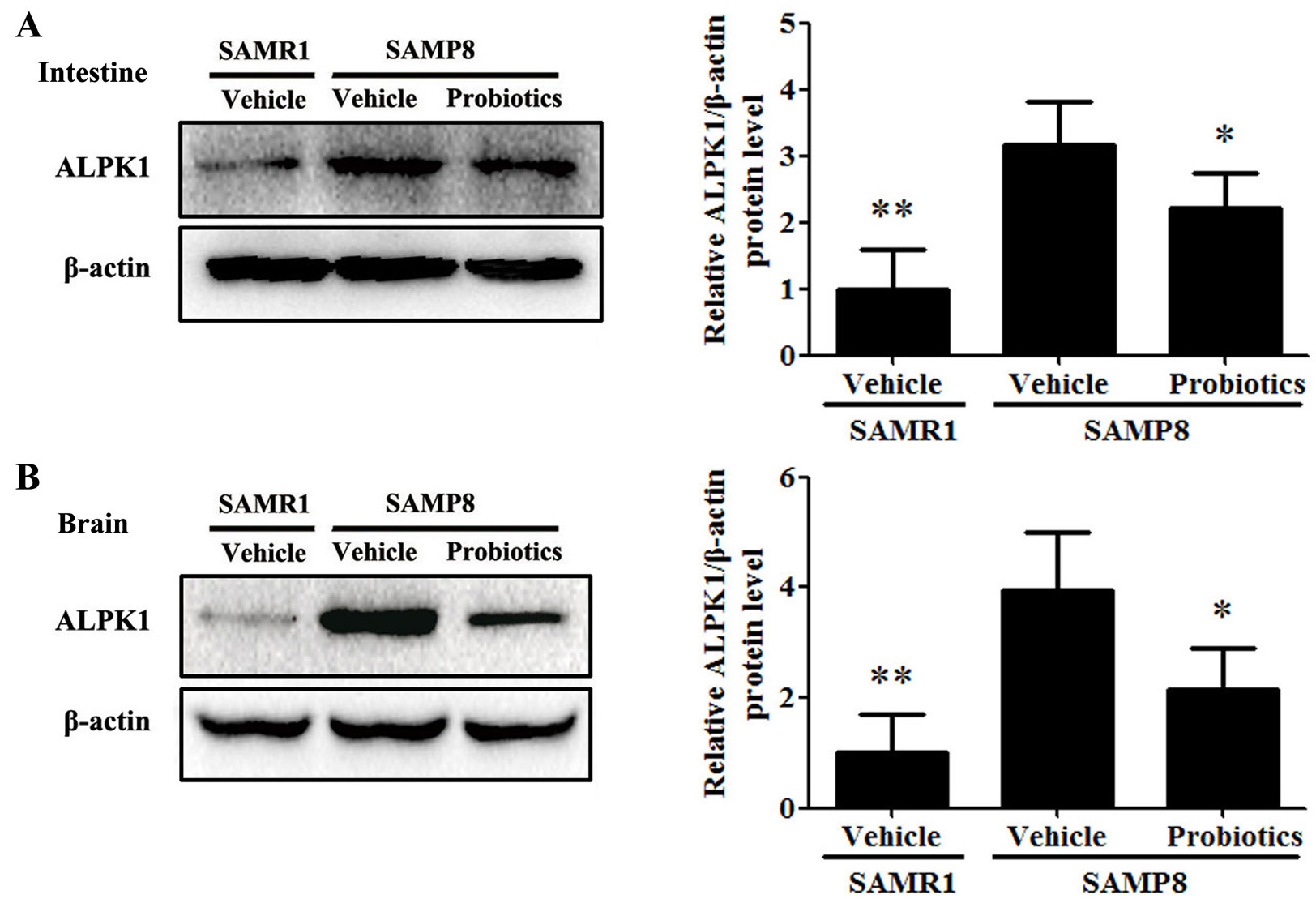
Probiotic-4 decreased the expression of ALPK1 in SAMP8 mice.
(A,B) Representative immunoblots and quantitative analysis of ALPK1 expression in
the intestine and brain of SAMR1 and SAMP8 mice. The data are expressed as mean
Although cognitive impairments are a common health problem in the elderly
population, effective therapies for these conditions have yet to be discovered.
Recent studies have drawn attention to the novel roles of probiotics in some
gut-brain axis related conditions [28]. Some workers have reported that
probiotics slowed the progression of cognitive decline in subjects with mild
cognitive impairment [29], although the molecular mechanisms remain to be
uncovered. Our previous research found that ProBiotic-4 could
attenuate cognitive deficits in aged SAMP8
mice. The mechanism of action appeared to be related to the inhibition of TLR4-
and RIG-I-mediated NF-
The present study used 9-month-old SAMP8 mice. These have previously been shown to exhibit cognitive deficits and neuronal injury compared with age-matched SAMR1 mice [21]. Similar to previous studies [30], vehicle-treated SAMP8 mice exhibited impairments in memory ability compared with age-matched SAMR1 mice. ProBiotic-4 treatment of SAMP8 mice significantly improved their spatial learning and memory ability, as determined by the Morris water maze test. ProBiotic-4 also improved neuropathological injuries in SAMP8 mice compared with vehicle-treated controls, including synaptic injury, neuronal loss and microglial activation, as well as reducing neuroinflammation in the brain. These findings suggest that ProBiotic-4 may improve cognitive impairment at least in part by inhibiting neuroinflammation.
Ever since the notion of a gut-brain axis was first proposed, the relationship
between cognitive function and gut microbiota has become an active field of
study. Dysbiosis of the gut microbiota can lead to an intestinal inflammatory
response, intestinal barrier damage, and the intestinal leakage of
proinflammatory cytokines, resulting in neuroinflammation [31]. Similar to the
above studies, we observed increased IL-1
When pathogenic bacteria invade the body, bacterial
pathogen-associated molecular patterns (PAMPs) are recognized
by special pattern recognition receptors and
these activate the body’s immune response
[34]. LPS is a component of the Gram-negative
bacterial cell wall and is also a PAMP that promotes activation
of caspase-11 [35, 36]. Together with caspase-1, caspase-11 is essential for the
production of IL-1
In summary, the present study confirmed that the mechanism by which probiotics improve cognitive impairment involves inhibition of the caspase-11/caspase-1 signaling pathway, reduction of ALPK1 expression, and reduction of inflammation in the gut-brain axis. Our findings shed more light on the mechanism by which probiotics improve cognitive impairment, thereby providing an experimental basis for further research on their beneficial effects. Since a multi-strain formulation of probiotic was used in this study, it is unclear whether the mechanism of action is driven by one or more specific strains. Therefore, further comparisons between individual strains and multiple combinations need to be made to help understand the mechanism of action of probiotics on cognitive impairment.
All data generated or analyzed during this study are included in this published article.
XQY—performed the research and drafted the manuscript; YZ and JZ—revised manuscript and made figures; LX—Validation and methodology; HSW—analysis of data; JRD—designed the work; ZX—revised manuscript, checked the statistical analysis and resulted analysis. All authors contributed to editorial changes in the manuscript. All authors read and approved the final manuscript. All authors have participated sufficiently in the work and agreed to be accountable for all aspects of the work.
The procedures were approved by the Animal Research Committee of West China School of Pharmacy (approval number: SYXK(Chuan)2018-113).
Not applicable.
This work was supported by the National Natural Science Foundation of China (81473219 and 81973307) and partly by 111Project of the National Ministry of Education (B18035, China).
The authors declare no conflict of interest.
Publisher’s Note: IMR Press stays neutral with regard to jurisdictional claims in published maps and institutional affiliations.