†These authors contributed equally.
Academic Editor: Rafael Franco
Background: The difference in spontaneous brain activity between acute subjective tinnitus patients (with or without hearing loss) and control participants was explored using the amplitude of low-frequency fluctuations and degree centrality methods through resting-state functional magnetic resonance imaging. The study aimed to provide an objective basis for clinical diagnosis and pathogenesis of patients with acute subjective tinnitus. Methods: Fourteen acute subjective tinnitus (AST) patients with hearing loss (AST-HL), 6 AST patients with no hearing loss (AST-NHL), and 14 healthy controls (HCs) with age, sex, and education status matched were recruited for this study. Resting-state functional magnetic resonance imaging (fMRI) examinations were performed in a resting state and the amplitude of low-frequency fluctuations (ALFF) and degree centrality (DC) values of each group were acquired. Statistical analysis was performed to assess the ALFF and DC values of different brain areas of the participants (AST-HL and AST-NHL were compared with HCs, but AST-HL and AST-NHL were not). Results: Patients with acute subjective tinnitus and hearing loss showed a significantly increased amplitude of low-frequency fluctuation values in the left middle temporal gyrus and bilateral frontal gyrus/marginal lobe/cingulate gyrus but a decreased amplitude of low-frequency fluctuations values in the bilateral superior temporal gyrus/anterior cerebellar lobe in comparison with healthy controls. The amplitude of low-frequency fluctuation values of patients with acute subjective tinnitus and hearing loss in the right posterior lobe of the cerebellum, bilateral temporal gyrus, bilateral lenticular nucleus, bilateral frontal gyrus, right inferior occipital gyrus, were higher, but were significantly lower in the bilateral anterior lobe of cerebellum/superior temporal gyrus and left posterior cerebellar lobe as compared with those of healthy controls. Degree centrality values in the healthy controls group were increased in the right superior marginal gyrus and decreased in the right thalamus in patients with acute subjective tinnitus and hearing loss, while patients with acute subjective tinnitus with no hearing loss presented significantly higher degree centrality values in the left frontal lobe and lower degree centrality values in the left center rear return. Conclusions: Aberrant amplitude of low-frequency fluctuations and values exist in various brain regions, indicating abnormal spontaneous brain activity in both acute subjective tinnitus and hearing loss and acute subjective tinnitus no hearing loss patients. The pathogenesis of acute subjective tinnitus may be related to abnormalities in both the auditory cortex and nonauditory cortex. These findings provide more evidence to help clarify the neuronal symptoms of acute subjective tinnitus patients.
Tinnitus, a common hearing disease, can be subdivided into subjective tinnitus and objective tinnitus. Subjective tinnitus is often observed in clinical settings, while objective tinnitus is rare. Without any corresponding external auditory source, tinnitus can be expressed by affected patients in a variety of ways including ringing, buzzing, hissing, or clicking. Additionally, tinnitus can be accompanied by different degrees of hearing loss. The prevalence of tinnitus is about 10% to 15% in humans [1] and the incidence rate of tinnitus increases with age, so it is more common among adults older than 65 years [2]. Tinnitus has become the third most harmful otological disease after deafness and vertigo. According to the 2014 United States tinnitus Clinical Application Guidelines, acute subjective tinnitus (AST) refers to tinnitus with an onset time of no more than six months. Long-term tinnitus can have a serious impact on patients, who may also suffer from sleep disorder, inattention and emotional disorder. As compared with chronic tinnitus, patients with AST are more motivated to seek clinical help. Currently, the pathogenesis of tinnitus remains unclear, and effective objective examinations and tailored medicines for tinnitus are rare, causing difficulties for clinical treatment and nursing.
The mechanism of tinnitus has been studied for many years but has yet not been fully elucidated. Previously, it was generally believed that tinnitus occurs when the peripheral auditory system was acutely or chronically damaged or underwent pathological changes. Recently it has been found that tinnitus may continue to exist even if the auditory nerve is severed to block the input of sound to the ear, so tinnitus is not only linked to a damaged cochlea [1]. More recently, with the progression of basic research using tinnitus animal models, an updated theory of tinnitus centralization has been put forward: abnormal central neuronal activity and reorganization of the central cortex or subcortical structure may be the cause of tinnitus. Furthermore, nonauditory centers including the limbic system and autonomic nervous system may also participate in tinnitus.
The rapid development of neuroimaging and neuroelectrophysiology has made it possible to gather detailed evidence verifying recently updated theory. Resting-state functional magnetic resonance imaging (fMRI) has the advantages of being an objective and repeatable data acquisition technique. It displays the brain function activities of specific areas and is a powerful tool for observing auditory activities. The amplitude of low-frequency fluctuations (ALFF) is one type of fMRI data that measures the internal average response of the brain to indicate the intensity of local neuron activity. Specifically, it reflects the level of spontaneous activity of each single voxel from the perspective of energy and has been demonstrated to be an effective method for the evaluation of spontaneous changes in brain activity associated with various neurological or mental diseases [3]. The degree centrality (DC) value is the most direct index available to describe node centrality in a network analysis. The higher the DC value of a node, the more important that node is in the network. The DC method reflects the functional connection of the human brain at the voxel level. It has been successfully used to assess the pathological mechanisms of many diseases, such as primary open-angle glaucoma [4], schizophrenia with auditory hallucinations [5] and Parkinson’s disease [6].
Previous studies have shown that tinnitus might be correlated with abnormal spontaneous neuronal activity in the auditory and nonauditory centers (frontal lobe, marginal lobe and cerebellum) [7, 8, 9]. However, previously published results have varied widely, due to differences in the fMRI techniques and calculation methods applied. In the current study, ALFF and DC were used to analyze the brain function activities of patients with tinnitus. These two techniques can directly measure the intensity and connection state of neural activity between brain regions, and have high test-retest reliability. Therefore, the combination of these two methods may be more realistic than using either method alone. the ALFF and DC methods were employed to detect spontaneous brain activity in patients with AST to clarify the neuropathological mechanism of tinnitus-related symptoms.
The present study was approved by the Medical Ethics Committee of Nanchang University. Fourteen AST patients with hearing loss (AST-HL) and six AST patients with no hearing loss (AST-NHL) were included in the study alongside 14 healthy controls (HCs) who were matched to the AST patients according to age, sex and education state.
The inclusion criteria for patients with AST-HL and those with AST-NHL were as follows: (1) No neurologic or psychiatric history or standard MRI contraindications; (2) No organic tinnitus, myogenic tinnitus, vascular tinnitus, or certain systemic diseases such as diabetes, acoustic neuroma, Meniere’s disease, or unilateral cochlear injury caused by head trauma, infection, or hemorrhage; (3) An onset time of tinnitus of no more than six months prior; (4) Right-handed; (5) The ability to undergo pure tone audiometry at frequencies of 125, 250, 500, 1 K, 2 K, 4 K and 8 kHz, with an average hearing threshold of 0.125 to 8 kHz being more than 30 dB or not more than 30 dB in all patients; (6) A tinnitus diagnosis confirmed by the main complaint, history inquiry, general clinical examination, audiology test and tinnitus examination according to the 2015 Tinnitus Clinical Application Guidance; and (7) An fMRI examination performed after admission before any treatment. All AST patients were asked to complete the Tinnitus Handicap Inventory (THI) to assess the severity of their tinnitus; the higher the total score (0–100 points), the greater the impact of tinnitus on daily life.
During the same period, 14 HCs were recruited who met the following criteria: (1) No tinnitus, vertigo, hearing loss, or other vestibular diseases; (2) Normal otology and audiology examination findings; (3) No system or psychiatric diseases; (4) Right-handed; and (5) No contraindications for MRI scanning.
All participants underwent MRI scanning using a 3.0-Tesla Verio scanner
(Siemens, Erlangen, Germany) at the First Affiliated Hospital of Nanchang
University. During the scanning process, patients were asked to remain quiet with
their eyes closed. During imaging, gradient-echo echo-planar imaging sequence was
performed with the following parameters: repetition time 2000 ms, echo time 40
ms, flip angle 90
Bold data were processed using SPM8 software
(http://www.fil.ion.ucl.ac.uk/spm;
Wellcome Centre for Human Neuroimaging, UCL Queen Square Institute of Neurology,
London, UK) on the MATLAB platform (MathWorks Inc, Natick, MA, USA). Specific
procedures were as follows: (1) The first 10 volumes were removed for each
subject and the remaining 230 useful volumes were corrected for slice timing and
motion correction, (2) The data underwent spatial standardization according to
the Montreal Neurological Institute (MNI) template (resampling voxel size of 3
Data Processing & Analysis for Brain Imaging software was used to calculate ALFF and DC values and resting-state data analysis was adopted for the purposes of statistical analysis.
The ALFF value was obtained with the following steps. First, a 6 mm
full-width-at-half-maximum Gaussian kernel was used to smooth the
z-score map, each voxel ALFF value of each frequency point was divided
by the average value of the whole-brain ALFF value to obtain a standardized ALFF
value. Spatial smoothing was then used to correct and reduce the errors with the
Gaussian random field (GRF). Finally, a comparison between groups was completed
with the SPM8 software. Clusters with more than 26 adjacent suprathreshold voxels
were selected as the regions with statistically significant differences between
the two groups (p
The voxel-wise functional network was generated for each subject, for which we
took each voxel as a node and inter-voxel correlations as the edge. Within the
default brain mask provided by DPARSFA (in the MNI-152 standard space with 3
Zi =
where i is the voxel index, DCi is the DC value for the i-th voxel, std is the standard deviation, and Zi is the z-score for the i-th voxel. The z-score map was spatial smoothed with a Gaussian kernel of FWHM (full width at half maximum) of 6 mm.
The Statistical Package for the Social Sciences version 22.0 software (IBM Corporation, Armonk, NY, USA) was used to compare differences between groups (age, years of education, listening). The chi-squared test was used to compare the classification data (handedness and sex). A two-sample t-test was conducted to test the difference in z-scores between AST-HL or AST-NHL and HC groups. A p-value of less than 0.05 was taken to indicate significant difference.
No significant difference was found in terms of sex, age, education state or
handedness between AST-HL or AST-NHL patients and HCs (see Table 1). In the
AST-HL group, six cases occurred in the right ear where the average hearing
threshold was 53.5
AST-HL | AST-NHL | HC | p-value | p-value | |
Sex (male/female) | 7/7 | 3/3 | 7/7 | – | – |
Age (years) | 33.3 |
26.85 |
27.6 |
0.214 | 0.285 |
Handedness | 14R | 6R | 14R | – | – |
Education state (years) | 13.9 |
12.6 |
14.5 |
0.384 | 0.391 |
Tinnitus laterality (right/left) | 6/8 | 0/6 | – | – | – |
PTA of right ear (dB) | 53.5 |
– | 15.2 |
/ | |
PTA of left ear (dB) | 60 |
15.2 |
15.6 |
0.601 | |
Duration (days) | 3.35 |
37.5 |
– | – | 0.007 |
THI score | 69.7 |
42 |
– | – | 0.003 |
AST, acute subjective tinnitus; HC, healthy control; AST-HL, AST patients with hearing loss; AST-NHL, AST patients with no hearing loss; R, right; THI, Tinnitus Handicap Inventory. A chi-squared test for sex and handedness data and an independent t-test for continuous data were used. |
As compared with the HC group, the AST-HL group showed significantly increased ALFF values in the left middle temporal gyrus cluster voxel = 232 (t = 4.7469) and bilateral middle frontal gyrus/superior frontal gyrus/marginal lobe/anterior uncinate gyrus/cingulate gyrus cluster voxel = 712 (t = 5.9543) but decreased ALFF values in the bilateral superior temporal gyrus/anterior cerebellar lobe cluster voxel = 2442 (t = –6.050) left posterior cerebellar lobe cluster voxel = 216 (t = –6.137) bilateral posterior central gyrus cluster voxel = 549 (t = –5.649) see Table 2 and Fig. 1. As seen in Table 3 and Fig. 2 post hoc comparison of ALFF scores between AST-NHL and the combined HCs group, using a two-sample t-test, resulted in eleven clusters of increased ALFF scores in the AST-NHL group (the right posterior lobe of the cerebellum cluster voxel = 210 (t = 5.275), left middle temporal gyrus cluster voxel = 1140 (t = 6.451), right inferior temporal gyrus cluster voxel = 106 (t = 5.218), left lenticular nucleus cluster voxel = 194 (t = 5.633), right middle frontal gyrus cluster voxel = 375 (t = 6.410), right lenticular nucleus cluster voxel = 312 (t = 5.653), right inferior occipital gyrus cluster voxel = 549 (t = 5.452), left inferior frontal gyrus cluster voxel = 72 (t = 4.837), left superior frontal gyrus cluster voxel = 538 (t = 6.202), right medial frontal gyrus cluster voxel = 184 (t = 5.357), and right middle frontal gyrus cluster voxel = 328 (t = 5.984). Conversely, ALFF values in the bilateral anterior lobe of the cerebellum/midbrain/superior temporal gyrus cluster voxel = 2731 (t = –6.493) and left posterior cerebellar lobe cluster voxel = 188 (t = –5.909) in AST-NHL patients were significantly lower than those of HCs. However, as compared with among HCs, DC values were increased in the right superior marginal gyrus/inferior parietal lobule/angular gyrus cluster voxel = 384 (t = 3.784) and decreased in the right thalamus cluster voxel = 316 (t = –4.105) in AST-HL patients (shown in Table 4 and Fig. 3). Additionally, AST-NHL patients displayed significantly higher DC values in the left frontal lobe cluster voxel = 43 (t = 4.380) and lower DC values in the left center rear return cluster voxel = 64 (t = –5.403) (see Table 5 and Fig. 4).
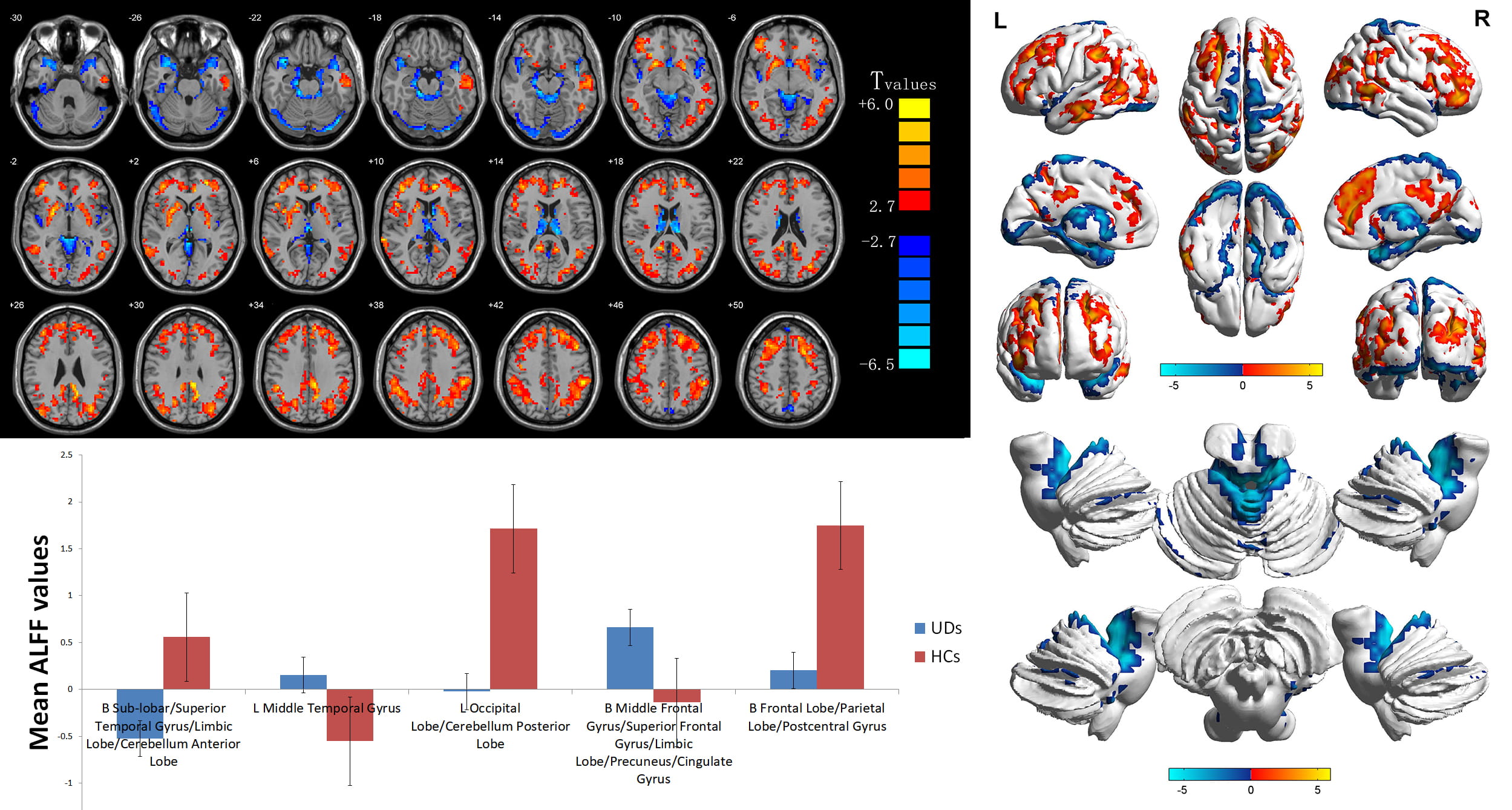
Voxelwise comparison of ALFF values between AST-HL and HC groups
(two-dimensional sections). Brain clusters show a significant difference in ALFF
values between AST-HL and HC groups (cluster-level p
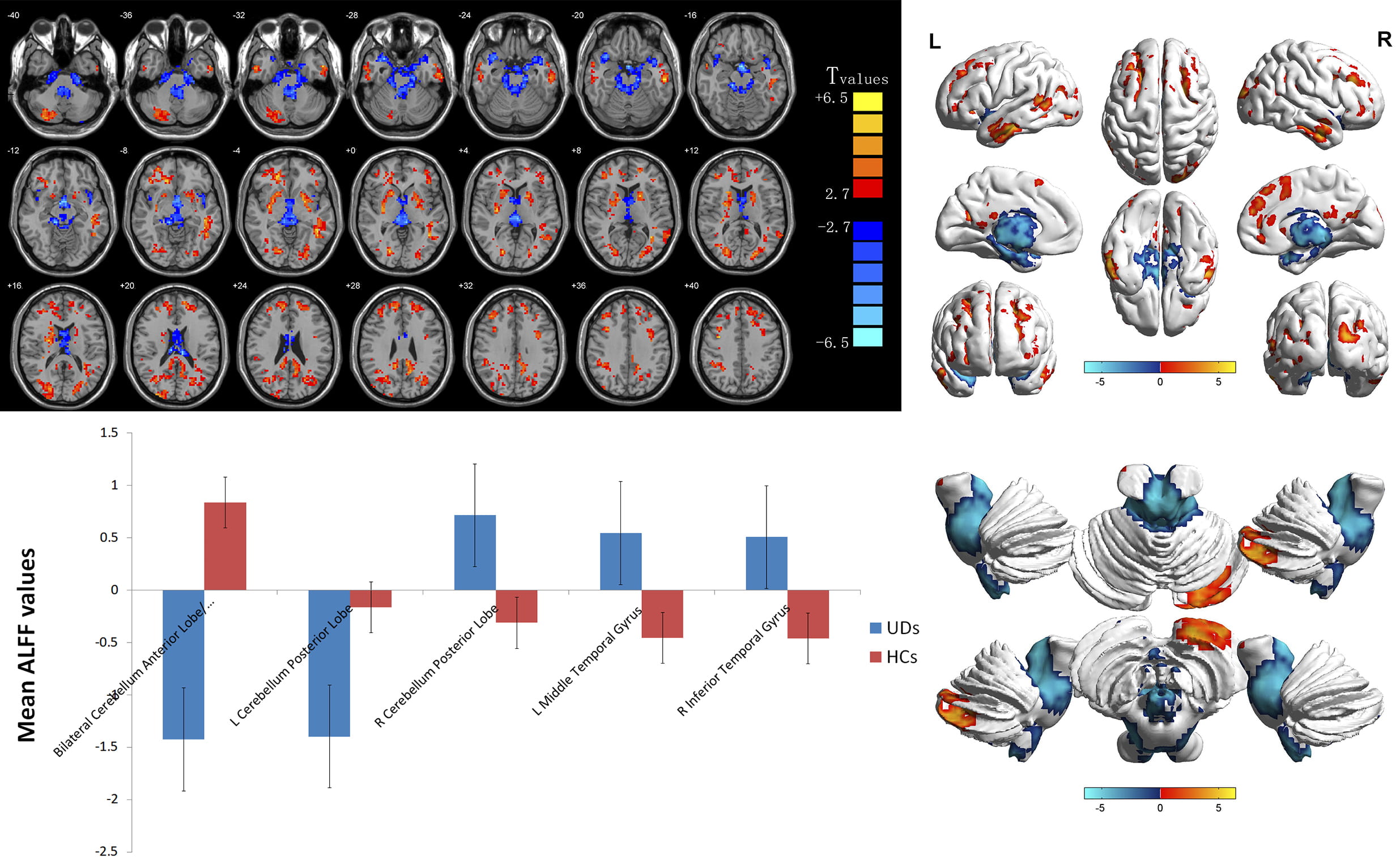
Voxelwise comparison of ALFF values between AST-NHL and
HC groups (two-dimensional sections). Brain clusters show a significant
difference in ALFF values between the two groups (cluster-level p
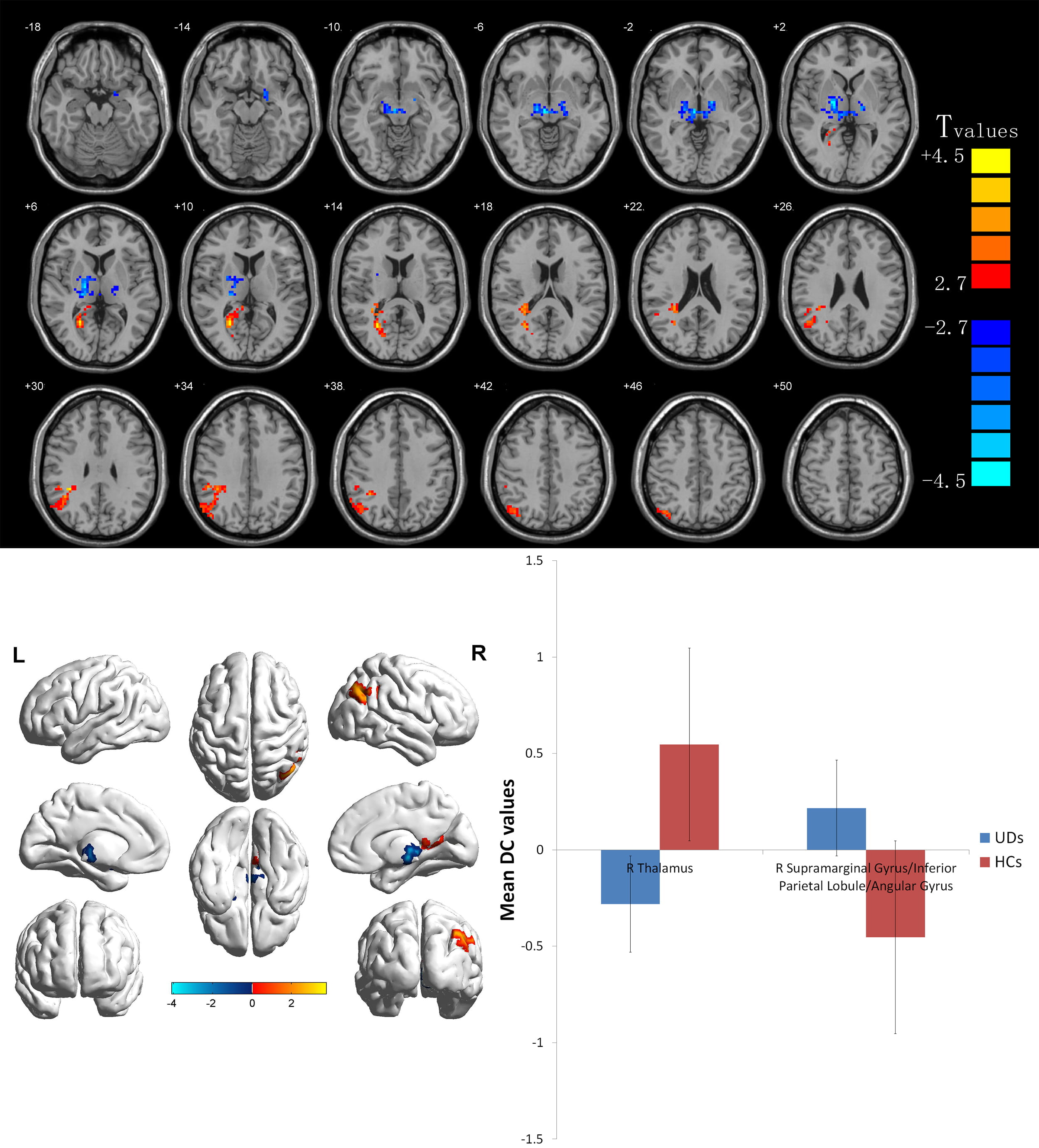
Voxelwise comparison of DC values between AST-HL and HC
groups (two-dimensional sections). Brain clusters show a significant difference
in DC values between these two groups (cluster-level p
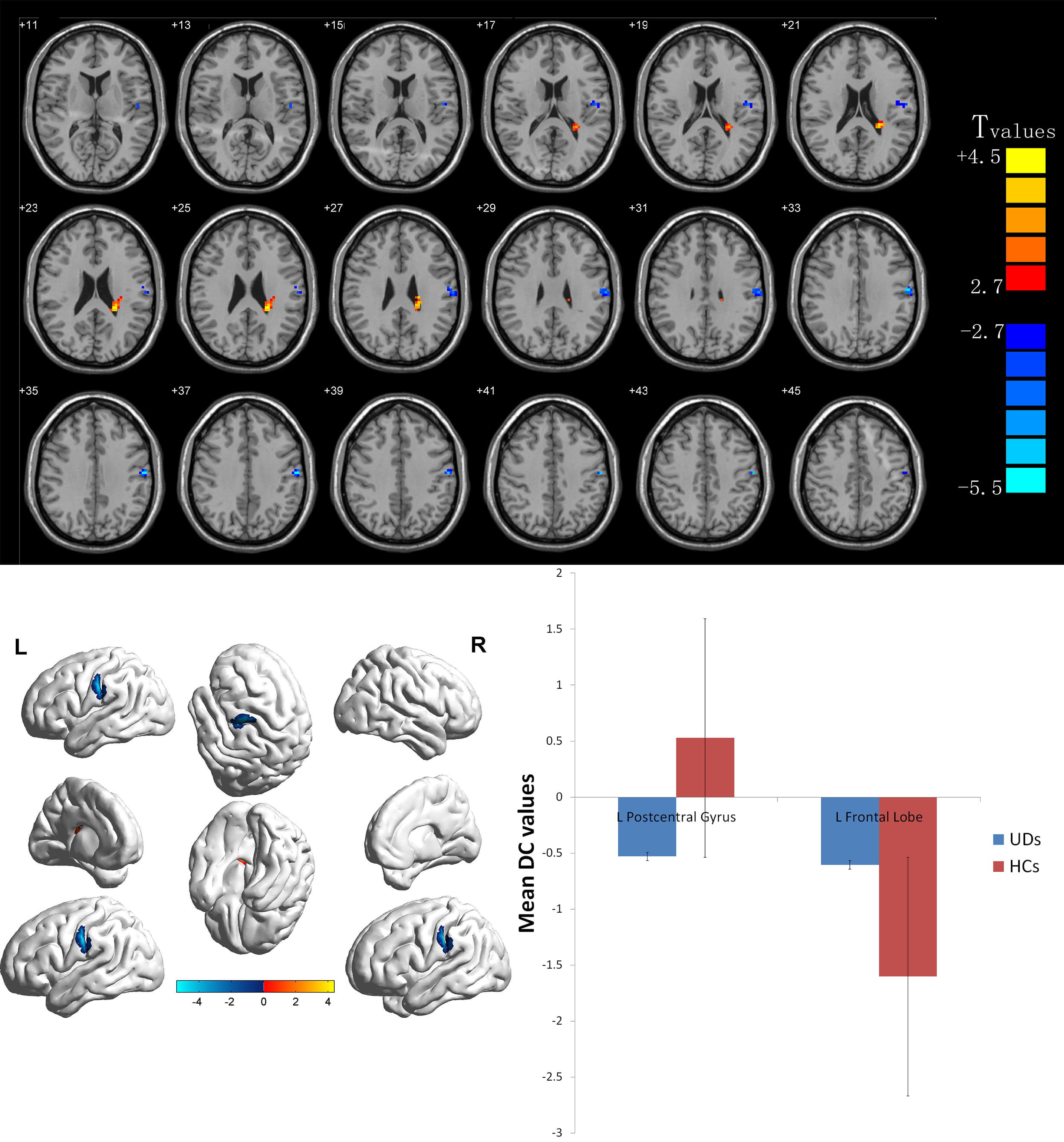
Voxelwise comparison of DC values between AST-NHL and
HC groups (two-dimensional sections). Brain clusters show a significant
difference in DC values between the two groups (cluster-level p
Brain area | Peak MNI coordinates | Cluster voxel | BA | L/R/B | t-value | ||
x | y | z | |||||
AST-HL |
|||||||
Middle temporal gyrus | –60 | –18 | –18 | 232 | 21 | L | 4.7469 |
Middle frontal gyrus/superior frontal gyrus/marginal lobe/anterior uncinate gyrus/cingulate gyrus | 21 | 12 | –9 | 7120 | 40 | B | 5.9543 |
AST-HL |
|||||||
Superior temporal gyrus/anterior cerebellar lobe | 15 | –30 | –18 | 2442 | 38 | B | –6.0499 |
Posterior cerebellar lobe | –45 | –75 | –21 | 216 | 18 | L | –6.1371 |
Posterior central gyrus | 3 | –24 | 78 | 549 | 6 | B | –5.6494 |
MNI, Montreal Neurological Institute; BA, Brodmann area. (x, y, z) Coordinates of the peak location in the MNI space, t statistical value of the peak voxel. |
Brain area | Peak MNI coordinates | Cluster voxel | BA | L/R/B | t-value | ||
x | y | z | |||||
AST-NHL |
|||||||
Posterior lobe of cerebellum | 30 | –84 | –39 | 210 | – | R | 5.2752 |
Middle temporal gyrus | –45 | –48 | –3 | 1140 | 7 | L | 6.4505 |
Inferior temporal gyrus | 54 | –6 | –30 | 106 | 21 | R | 5.2177 |
Lenticular nucleus | –24 | 0 | –3 | 194 | 47 | L | 5.6326 |
Middle frontal gyrus | 15 | 33 | –6 | 375 | 10 | R | 6.4096 |
Lenticular nucleus | 30 | –12 | 3 | 312 | – | R | 5.6533 |
Inferior occipital gyrus | 21 | –81 | 0 | 549 | 19 | R | 5.4516 |
Inferior frontal gyrus | –42 | 36 | 3 | 72 | 46 | L | 4.8373 |
Superior frontal gyrus | –18 | 24 | 45 | 538 | 8 | L | 6.2018 |
Medial frontal gyrus | 9 | 48 | 30 | 184 | 9 | R | 5.3571 |
Middle frontal gyrus | 9 | 18 | 51 | 328 | 6 | R | 5.9842 |
AST-NHL |
|||||||
Anterior lobe of cerebellum/midbrain/superior temporal gyrus | 0 | 0 | –18 | 2731 | – | B | –6.493 |
Posterior cerebellar lobe | –3 | –75 | –48 | 188 | – | L | –5.9085 |
MNI, Montreal Neurological Institute; BA, Brodmann area. (x, y, z), coordinates of the peak location in the MNI space, t statistical value of the peak voxel. |
Brain area | Peak MNI coordinates | Cluster voxel | BA | L/R/B | t-value | ||
x | y | z | |||||
AST-HL |
|||||||
Superior marginal gyrus/inferior parietal lobule/angular gyrus | 27 | –60 | 12 | 384 | 39 | R | 3.7843 |
AST-HL |
|||||||
Thalamus | 18 | –9 | 3 | 316 | 34 | R | –4.1048 |
MNI, Montreal Neurological Institute; BA, Brodmann area. (x, y, z) coordinates of the peak location in the MNI space, t statistical value of the peak voxel. |
Brain area | Peak MNI coordinates | Cluster voxel | BA | L/R/B | t-value | ||
x | y | z | |||||
AST-NHL |
|||||||
Frontal lobe | –24 | –36 | 21 | 43 | – | L | 4.3801 |
AST-NHL |
|||||||
Center rear return | –60 | –15 | 33 | 64 | 3 | L | –5.4032 |
MNI, Montreal Neurological Institute; BA, Brodmann area. (x, y, z) coordinates of the peak location in the MNI space, t statistical value of the peak voxel. |
The current study revealed findings of altered brain activity related to AST patients for the first time by combining ALFF and DC analyses. Specifically, extensive brain regions were identified that belonged to the auditory network, default mode network and visual network that presented remarkably different ALFF and DC values in the study population. More generally, the reported findings support that neuroimaging may be useful for detecting the characteristics of neural network modifications in patients with AST by indicating the neuropathological mechanism of tinnitus.
The auditory cortex is divided into the primary and secondary cortices. This study reported increased ALFF values in the middle temporal gyrus (secondary auditory cortex) and decreased ALFF values in the superior temporal gyrus (primary auditory cortex) in both the AST-HL and AST-NHL groups. In a positron-emission tomography study, a correlation between higher brain metabolism in the middle temporal gyrus and tinnitus severity was confirmed [11]. Further, Chen et al. [9] recorded increased ALFF values in the right middle temporal gyrus of patients with chronic tinnitus. Additionally, a greater level of tinnitus disturbance was correlated with increased ALFF values in the middle temporal gyrus, suggesting that a change in the ALFF value may reflect an influence of tinnitus perceptions. Previous studies have demonstrated that the volume of the bilateral superior temporal gyrus in patients with unilateral pulsatile tinnitus was significantly larger than that in the normal control group, while FC in both the right superior temporal gyrus and left inferior temporal gyrus was abnormally increased [12, 13]. Contrary to the above results, the ALFF value of the superior temporal gyrus was significantly reduced in this study, suggesting dysfunction of the superior temporal gyrus. Therefore, it is speculated that the mechanism of tinnitus might be linked to activation of the secondary auditory center and inhibition of the primary auditory center.
The superior frontal gyrus and middle frontal gyrus may be key nodes in the tinnitus network responsible for receiving and integrating tinnitus sensory information and transmitting the impulses to maintain the overall coordination of the central nervous system. Geven [14] found an enhancement metabolism of the left middle frontal gyrus in tinnitus patients using 2-deoxy-2- (fluorine-18) fluoro-D-glucose positron-emission tomography. Additionally, a correlation between high neural activity in the middle frontal gyrus and the severity of tinnitus was observed [15]. The results of task-state fMRI also revealed an abnormal degree of activation of the superior frontal gyrus in tinnitus patients [16]. In chronic tinnitus patients, the FC of the left middle frontal gyrus was also significantly increased [17]. Consistent with previous studies, the findings reported here included significantly higher ALFF values from the superior and middle frontal gyrus in both AST-HL and AST-NHL patients. However, a previous study has shown that regional homogeneity (ReHo) values in several brain regions of the tinnitus group, including the inferior frontal gyrus, were increased [18]. Increased ALFF values were also observed in this study in the inferior frontal gyrus in the AST-NHL group Acting as the core brain area of the central inhibitory response, the inferior frontal gyrus might reflect the presence of long-term control of central attention allocation to tinnitus perception from the bottom to top. It has been hypothesized that a default mode network (DMN) exists in the human brain, with the prefrontal gyrus holding an important role [19]. The DMN is involved in scene memory, self-evaluation and internal expression. Schmidt et al. [20] have also reported specific changes in patients with tinnitus, including connectivity alterations in the DMN and dorsal attention network. They speculated that DMN might play a key role in the neurophysiological process of tinnitus. Taken together, tinnitus patients show extensive abnormally spontaneous brain activity in the frontal lobe, indicating that the frontal cortex may be a participant in the pathogenesis of tinnitus.
The limbic system and frontal lobe are the main brain areas that participate in emotion processing. However, studies are increasingly pointing to the fact that tinnitus is not only limited to the auditory system but also closely related to the psychological activities of emotion and memory. Boyen [21] contended that gray matter in the marginal lobe of tinnitus patients with hearing loss was increased. Significantly greater ReHo values were also found in tinnitus patients, related to the increased tinnitus duration and hearing threshold of tinnitus [22]. In this study, abnormal ALFF values were obtained in the marginal lobe, anterior uncinate gyrus and cingulate gyrus, which might be related to psychological problems linked to stress, anxiety, inattention and insomnia present in AST patients. From an anatomical perspective, there was an indirect fiber connection observed between the auditory cortex and the limbic system, which allowed for a new model for the pathophysiology of tinnitus. In this context, the abnormal activity of the auditory system is transmitted to the brain and experienced as tinnitus. Simultaneously, negative experiences such as fidgeting, insomnia, depression and fear are generated due to the involvement of the limbic system and induce the perception of tinnitus, resulting in an abnormal cycle between tinnitus and negative characteristics or feelings.
The cerebellum has always been considered as the center of coordination for the random movement and contraction of muscles in the whole body. However, a recent study showed that the cerebellum also plays an important role in auditory afferent processing [23]. Bauer [24] observed that the cerebellum might be an important and nonmandatory site of tinnitus in a rat model of noise-induced chronic tinnitus. Once cerebellum lesions occur, tinnitus signals might appear. Tinnitus patients additionally showed significantly decreased ReHo values in the anterior lobe of the cerebellum [25]. Han [26] recorded altered ALFF values in the posterior lobe of the right cerebellum in pulsatile tinnitus patients in a manner linked to the diversity and duration of tinnitus. The results reported here indicate that ALFF values in the left posterior cerebellar lobe and bilateral anterior lobe of cerebellum were decreased in patients with tinnitus, which confirmed that the integration of cerebellar functional processing of cochlear afferent signals might be involved in the occurrence and persistence of tinnitus.
In the AST-NHL group, it was observed that the visual and extrapyramidal systems were activated. As an important part of the visual cortex, the inferior occipital gyrus is involved in logic analysis in the brain and the processing of visual memory. Based on the anatomic connection between auditory and visual areas, Ibrahim [27] suggested that the auditory cortex directly regulates the visual cortex. Therefore, the current authors speculated that higher ALFF values in the right suboccipital gyrus in AST-NHL patients might be the result of the activation of the visual center triggered by tinnitus. The above result was consistent with that of Zhou et al. [2]. However, it was found instead that the central visual system was not activated in the AST-HL group, which might be due to the time of onset or degree of hearing loss in the current study population. This study also reported increased ALFF values in the bilateral lenticular nucleus of the AST-NHL group. The lenticular nucleus belongs to the striatum, which receives almost all projections from the cerebral cortex and has direct fiber connections with the auditory cortex and the medial geniculate body. As such, changes in the cortical striatal pathway might constitute an important factor in tinnitus. However, the specific role of the lenticular nucleus in tinnitus requires further investigation.
The DC values in the right superior marginal gyrus/inferior parietal lobule/angular gyrus were increased in AST-HL patients, suggesting that the DMN plays an important role in tinnitus. The DMN influences negative emotions and attention disorders among patients. Therefore, it was speculated that the clinical manifestations of anxiety and insomnia in these patients might be correlated with increased activity of the DMN and limbic system network, causing a damaging cycle between tinnitus and negative events or feelings, as described above. The thalamus has also been found to be essential for the processing of tinnitus [28]. As an important component of many pathways of the brain, the thalamus transmits sensory information to the cerebral cortex and is the most important hub of sensory transmission. Except for olfaction, all other sensory pathway transmission neurons in the body are managed in the thalamus. Llinás proposed a model of thalamic cortical rhythm disorder that suggested that tinnitus was caused by the interruption of coherent oscillation between the thalamus and cortex [29]. Impaired white matter integrity involving thalamic connectivity was previously identified in tinnitus patients [30]. Other studies have also observed decreased short-range FC density and ALFF values in the thalamus [9, 31]. Consistent with the foregoing results, this study recorded significantly decreased DC values of the right thalamus in the AST-HL group, speculated to be caused by hearing loss—that is, in AST-HL patients, decreased auditory information stimulation caused weakened thalamo-cortical connections, thus leading to changes in the normal rhythm of the thalamo–cortical ring and ultimately, the generation of tinnitus.
As described above, the frontal gyrus was widely activated in tinnitus. Significantly higher DC values were obtained from the frontal gyrus of AST-NHL patients, which reinforced the fact that the frontal lobe plays an indispensable role in tinnitus. The posterior central gyrus, functioning as the primary somatosensory cortex, has been referred to as the joint area together with the frontal lobe and temporal lobe [32]. In this study, remarkably lower ALFF values were observed in the posterior central gyrus in AST-HL patients and decreased DC values were observed in the posterior central gyrus in AST-NHL patients. The dysfunction of the posterior central gyrus might be associated with sensory abnormalities in tinnitus patients.
First, this study was based on temporal sequences and the sample size was relatively small, so larger subject groups should be studied in the future. Second, patients with tinnitus on either the left or right side were included in this study, which does not explain the lateral problems of brain function. Third, the noise during fMRI scanning was quite loud. Although measures were taken, such as wearing earmuffs, this may not have totally eliminated this influence on the experimental results.
The results reported here confirmed that the auditory and nonauditory centers of tinnitus patients showed abnormal ALFF and DC values, indicating that both the auditory center and nonauditory centers (limbic system, frontal lobe, cerebellum, posterior central gyrus) are crucial for tinnitus. The neural activity of tinnitus brain area is related to the disease stage, suggesting that we should pay attention to the different stages of tinnitus disease; The abnormality of tinnitus brain network is mainly manifested in the enhancement of frontal lobe centrality and the decrease of posterior central gyrus centrality. fMRI data was employed to compare the abnormal activity brain area of tinnitus patients in a resting state, which is proposed to reflect the neural mechanism of tinnitus relatively objectively. The relationship between tinnitus and spontaneous brain activity of the auditory cortex, DMN, limbic system and attention network was revealed first by combining the ALFF and DC methods. In short, the current study provides details on a comprehensive neurological pattern in patients with AST.
JC, LF, carried out the experiments and wrote the manuscripts. GC wrote and edited manuscript and performed f-MRI experiments. YX, ZZ conceived the study, designed experiments and wrote the manuscript. BH, JC performed the acquisition, analysis and interpretation of data.
Medical research ethics committee of the First Affiliated Hospital of Nanchang University (2019) Yi Yan Lun Shen No. (100).
The authors would also like to thank Yu Guo for helpful comments and lively discussion.
This research received no external funding.
The authors declare no conflict of interest.
Publisher’s Note: IMR Press stays neutral with regard to jurisdictional claims in published maps and institutional affiliations.