Academic Editor: Rafael Franco
Traumatic brain injury (TBI), also known as head injury or brain injury, refers to the head injury caused by mechanical impact. It is necessary to develop effective new therapies for TBI injury. Gastrodin (GAS) is the main bioactive ingredient from the rhizome of Gastrodia elata and has significant therapeutic effect on nervous system diseases. However, the protective effects of GAS on brain tissue and related regulatory mechanism in traumatic brain injury remain elusive. Herein, we explored the role of GAS in traumatic brain injury and its related mechanism. We found Gastrodin reduced brain tissue injury and improved functional recovery of injury nerve in TBI rats, and alleviated inflammation. Gastrodin decreased the level of pyroptosis in brain tissue of TBI rats. Further, we found GAS suppressed NLRP3 inflammasome signaling pathway, and therefore suppressed pyroptosis and exerted neuroprotective effect. GAS could serve as a promising drug for TBI treatment.
Traumatic brain injury (TBI), also known as head injury or brain injury, refers to the head injury caused by mechanical impact, including primary brain injury and secondary brain injury [1]. TBI often causes severe paralysis or intellectual impairment in patients, with high rates of injury, disability and mortality [2]. The mechanism of injury includes multiple cascade-events, such as the release of excitatory toxic molecules, metabolic challenges, inflammation and apoptosis, accompanied by the destruction of the blood-brain barrier (BBB) and cell swelling, which eventually leads to cell death [3]. There has been no effective treatment strategies for TBI to date, though increasing attention has been paid to TBI. Therefore, it is necessary to develop effective new therapies for TBI.
Currently, there is a growing focus on natural products derived from herbal
medicines owing to their reduced adverse effect [4]. Gastrodin (GAS) is the main
bioactive ingredient from the rhizome of Gastrodia
elata, which is widely used for treating spasm, paralysis, dizziness, stroke and
dementia, and has significant therapeutic value for nervous system diseases [5].
Gastrodin can reduce the effects of amyloid
NOD-like receptor protein 3 (NLRP3) is a polymeric protein complex that can recruit and bind to Capase-1, in which the apoptosis-related macular protein of the adapter protein containing CARD (ASC) serves as a linker protein [10]. NLRP3 inflammasomes can sense some endogenous “danger signals” and trigger a variety of inflammatory diseases, including cardiovascular, renal, neurodegenerative diseases, and type 2 diabetes [11]. NLRP3 inflammasome is considered as a potential drug target for the treatment of inflammatory diseases, but there has been no available drugs targeting NLRP3 inflammasome in clinic [12]. Therefore, discovering a natural drug to regulate the NLRP3 inflammasome signaling pathway plays an important role in the treatment of TBI.
Herein, we explored the role of GAS in traumatic brain injury and the related mechanism. We found Gastrodin reduced brain tissue injury, improved functional recovery of injury nerve iand alleviated inflammation in TBI rats. Furthermore, Gastrodin decreased pyroptosis in brain tissue of TBI rats. Mechanically, we found GAS suppressed NLRP3 inflammasome signaling pathway, and could serve as a promising drug for TBI treatment.
In this study, 8-week-old male rats (Jackson Laboratories, ME, USA, 290–310 g) were used for construction of TBI models. Rats were kept on a 12-h light-dark cycle, with free access to food and water. All the animal experiments in this study were performed with the approval of the Ethics Committee of the First People’s Hospital of Hefei. Pentobarbital sodium (1%, 80 mg/kg) was used for anesthetic. Rats were fixed and the skull was exposed. TBI model was constructed with 200-g steel weight dropping from 5 cm height. Thus, the left cortex was moderately injured. The animals in the sham group were subjected to the same operation except for the dropped steel weight. 6 rats were used in each group. GAS (bought from Sigma-Aldrich, MO, USA) was injected into the peritoneal at the indicated doses. In TBI + GAS group, rats were given indicated doses of GAS (15, 30, and 60 mg/kg GAS) upon the finish of the operation once a day for 7 days. The doses were selected according to the previous study, which was used in in-vivo assays [13]. Thereafter, rats were sacrificed for further study.
Brain tissues were freshly excised and fixed with 10% paraformaldehyde
immediately. Then, tissues were embedded in paraffin and sliced into 5-
Neurological score was evaluated with a well-established, modified neurological severity scoring (mNSS) after TBI. The evaluation was carried out with single-blind method. Behavioral tests were repeatedly performed to further validate the outcomes. The mNSS test reflects of motor (muscle status, abnormal movement), sensory (visual, tactile, and proprioceptive), balance, and reflex functions of animals. Neurological score ranging from 0 to 18 (0 = normal function; 18 = maximal deficit) represents different degree of the neurological lesion.
For open-field test, rats were kept under moderate lighting for 15 min in open field, with recording software (ANY-Maze, Stoelting, USA). The traveling distances were recorded to reveal the general activity. The anxiety related behavior was measured in open-field test (center vs. periphery).
Motor coordination and motor learning were monitored with rotarod apparatus (RWD Life TBIence, China). Before the surgery, rats were trained for 3 consecutive days on the rotarod to get baseline latency. Then, rats were subjected to four 300-s accelerating rotarod trials with 30 min intervals. Passive rotation, as well as the rotating rod without walking, was also considered as a fall.
To evaluate the inflammation status in each group, the concentration of tumor
necrosis factor-
Proteins from brain tissues were extracted with RIPA lysis buffer (Cell
Signaling, Boston, USA). Then, the samples (20
Results were displayed as mean
To evaluate the effect of GAS on brain deficits in TBI rats, the histological analysis of rat brain in different groups was performed by H&E staining. As shown in Fig. 1, sham group showed no cortical lesion while clear cortex injure was observed in TBI rat brain. Moreover, decreased number of neurons, nuclear pyknosis and degeneration were observed in the brain stem region of rats with TBI treatment (Fig. 1). GAS treatment significantly relieved the brain lesion in a dose dependent manner. Besides, in the mNSS test, rats in the sham group showed low mNSS score. However, TBI caused significantly higher mNSS compared to the sham treatment. The scores were reduced in GAS treated TBI rats. The results indicated that GAS promoted nerve recovery after TBI.
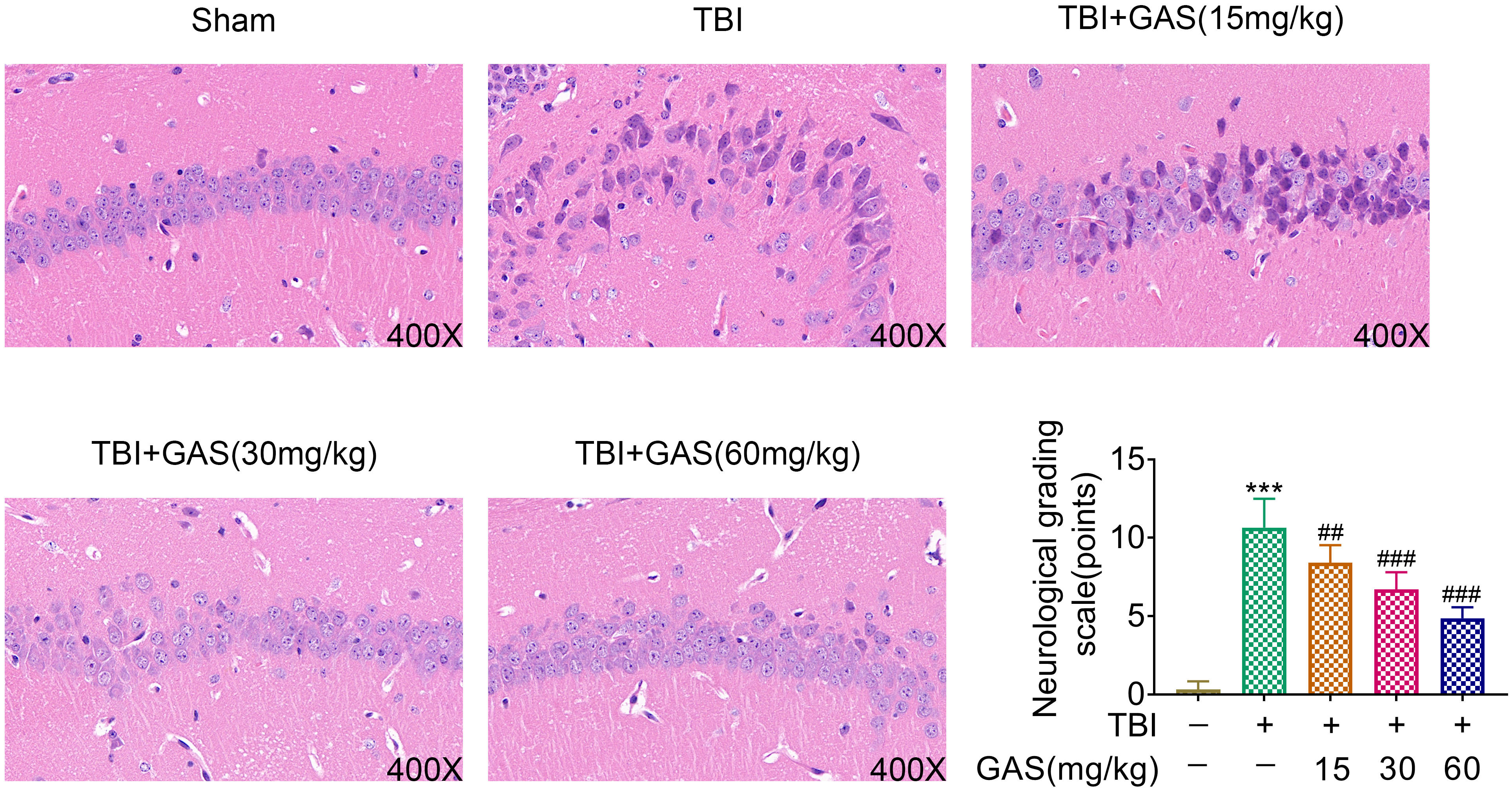
GAS alleviated brain injury of TBI rats. The histological
images and the neurological lesion score of brain in sham, TBI, TBI + GAS 15, 30
and 60 mg/kg groups were evaluated by HE staining. The decreased number of
neurons, nuclear pyknosis and degeneration were observed in the brain stem region
of TBI treatment group. GAS treatment significantly relieved the brain lesion in
a dose dependent manner. ***, p
To evaluate the inflammatory response in the cortex, the level of
TNF-
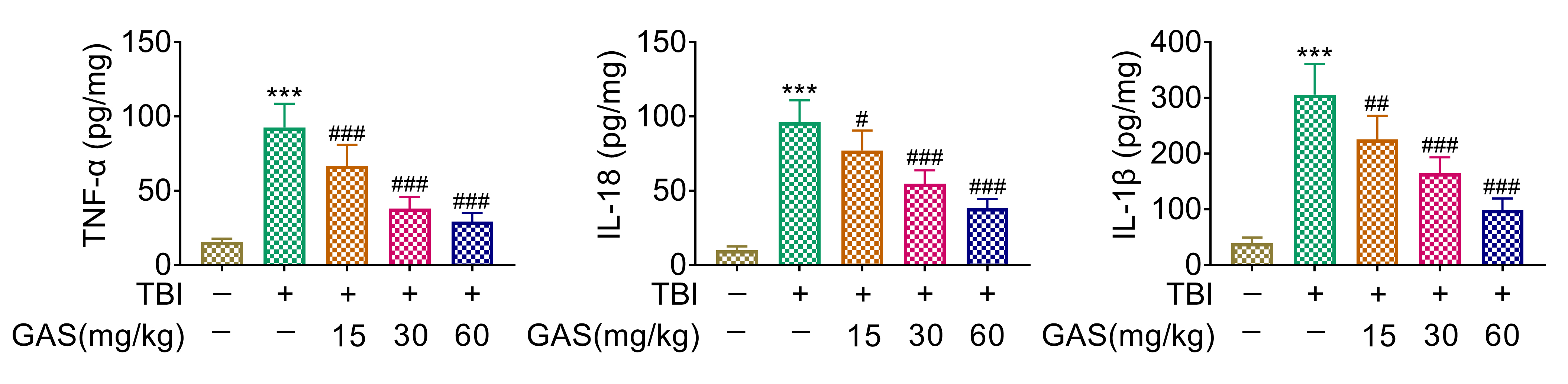
GAS reduced inflammatory responses in the brain of TBI rats.
The concentration of inflammatory cytokine in the brain of sham, TBI, TBI + GAS
(15 mg/kg), TBI + GAS (30 mg/kg), and TBI + GAS (60 mg/kg) group was detected by
ELISA. ***, p
To unveil the cellular mechanism in GAS-mediated TBI, we assessed the effect of GAS on cell pyroptosis by western blot. As shown in Fig. 3, the protein expression of GSDMD, caspase-1 and caspase-11 were increased in the brain in TBI rats compared with that in the sham rats. GAS treatment obviously lowered the expression of GSDMD, caspase-1 and caspase-11 in a dose dependent manner (Fig. 3), indicating the suppressive effect of GAS on cell pyroptosis in TBI model.
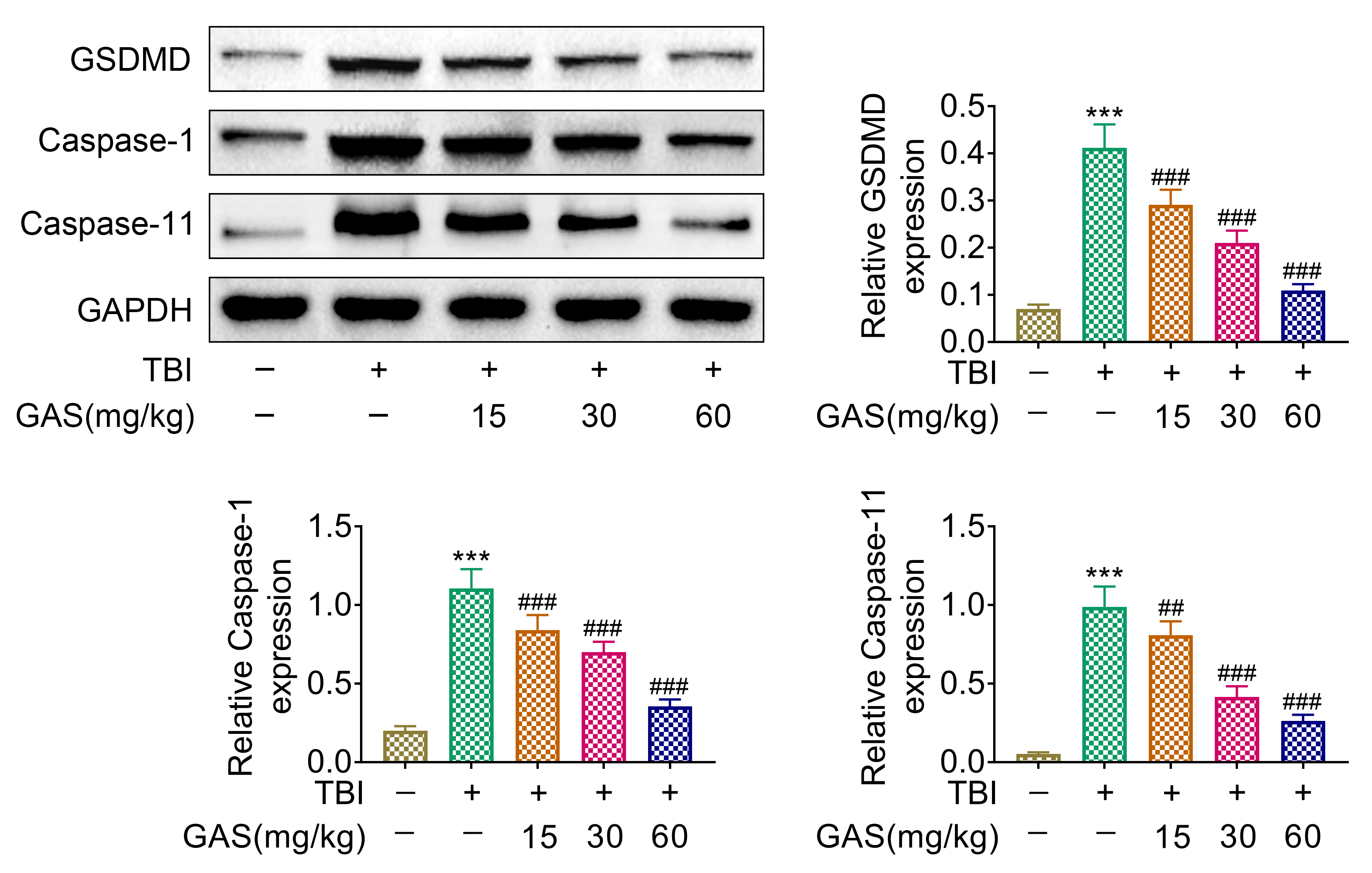
GAS treatment repressed cell pyroptosis in the brain of TBI
rats. The expression level of GSDMD, caspase-1 and caspase-11 in the brain of
sham, TBI, TBI + GAS (15 mg/kg), TBI + GAS (30 mg/kg), and TBI + GAS (60 mg/kg)
group was detected by immunoblot assays. ***, p
For uncovering the potential mechanism of GAS-mediated effect on TBI rats, the
role of GAS in NLRP3 signaling pathway was assessed in each group. The NLRP3
signaling pathway was activated following the construction of TBI model as
demonstrated by increased expression of NLRP3, ASC, IL-1
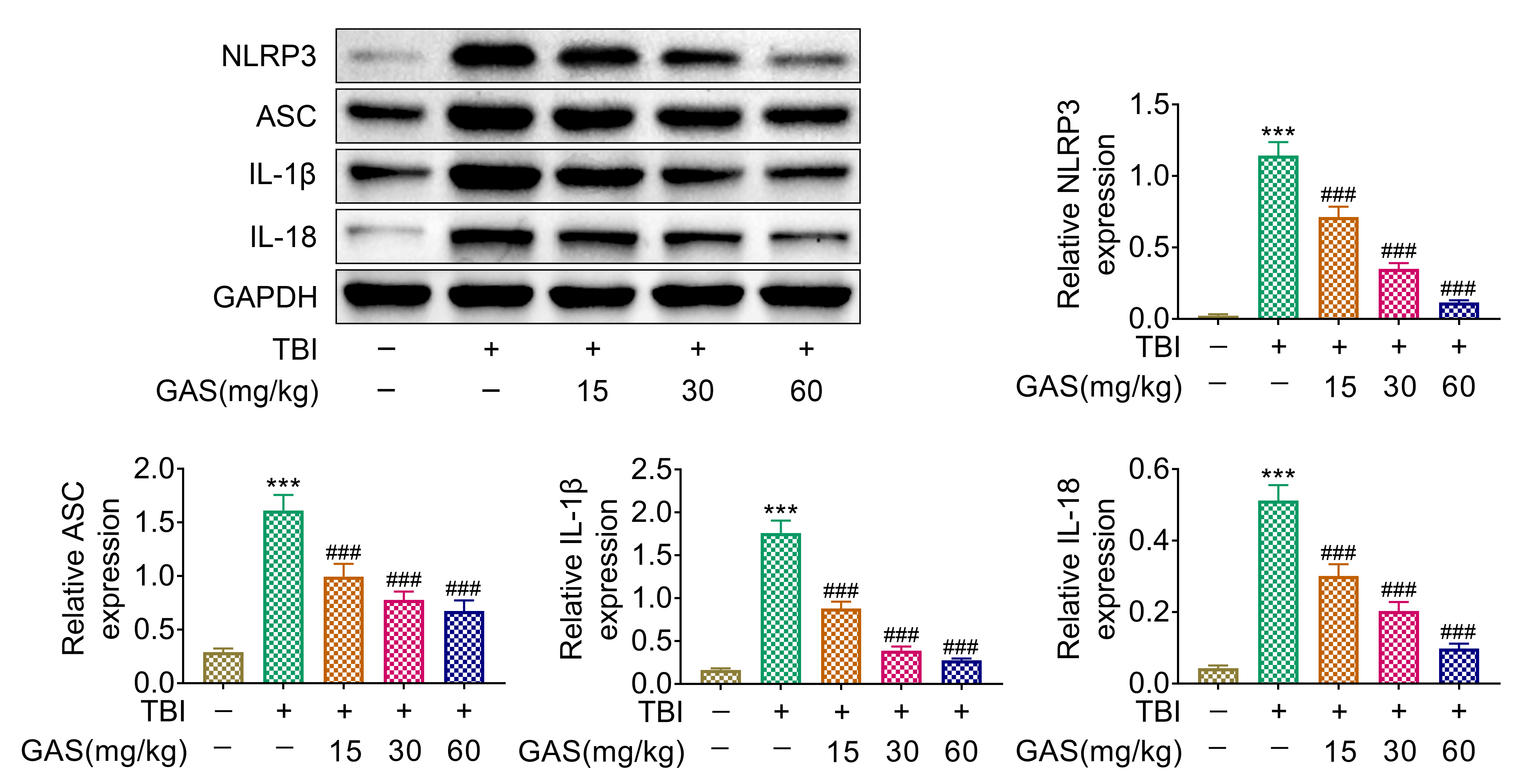
GAS suppressed NLRP3 inflammasome signaling pathway in the brain
of TBI rats. Immunoblot assays showed the expression level of NLRP3, ASC, IL-1b
and IL-18 in the brain of sham, TBI, TBI + GAS (15 mg/kg), TBI + GAS (30 mg/kg),
and TBI + GAS (60 mg/kg) group. The quantification was performed. ***, p
Traumatic brain injury (TBI) is a very common brain injury, which can be divided into two types: primary and secondary injury [14]. TBI has a high rate of disability and mortality, and it is very important to find effective treatment. The pathogenesis of TBI is a complex process, which is worthy of careful study and exploration [15]. Previous studies have shown that TBI causes a series of cellular responses, such as inflammation and apoptosis [16]. To date, there is no effective treatment for TBI. The development of effective treatments for TBI to reduce mortality remains a top priority. Importantly, we noticed a natural product, GAS, had the potential for the treatment of TBI. We further investigated its effects on TBI rat brain tissues and disclosed the underlying mechanisms.
HE and mNSS assays revealed that GAS improved nerve functional recovery and alleviated brain tissue injury in TBI rats. Furthermore, ELISA, and western blot assays suggested the counteractive role of GAS on the inflammation and pyroptosis of brain tissues in rats. Interestingly, the biological activities of GAS have been widely studied [9, 17]. GAS could attenuate inflammatory response and inhibit the migration of activated microglia [18]. Moreover, GAS alleviated perioperative neurocognitive dysfunction of aged mice via inhibiting neuroinflammation, and promoted CNS myelination [19]. GAS also exhibited influence on the activated microglia in cerebral hypoxic-ischemia neonatal rats [20]. Some study found that GAS could alleviate cerebral ischaemia/reperfusion injury via suppressing pyroptosis [21]. Similarly, GAS also exhibited suppressive effect on the inflammatory response and pyroptosis in TBI brain tissues. In addition, a previous study revealed the immunomodulatory and the possible therapeutic role of GAS in perinatal hypoxic-Ischemic brain damage. These studies collectively showed GAS could serve as a promising drug for the treatment of brain-related neuro diseases.
This study also demonstrated that GAS had an inhibitory effect on the pyrogenesis of brain tissues. In fact, the pyrogenesis played a critical role in the pathogenesis of TBI. Cell pyrogenesis is a type of programmed cell death and a natural immune response of the body to injury [10]. Several studies have confirmed the role of cell pyrotosis in TBI [22]. Inhibition of pyrotosis is important for the effective treatment of TBI.
In this study, we noticed GAS mediated the NLRP3 inflammasome signaling pathway. NLRP3 inflammasomes can sense some endogenous “danger signals” and trigger a variety of inflammatory diseases, including cardiovascular, renal, neurodegenerative diseases, and type 2 diabetes [22]. NLRP3 inflammasome is considered as a potential drug target for the treatment of inflammatory diseases, but drugs targeting NLRP3 inflammasome are not available in clinic. Previous studies have confirmed that gastrodin can improve cell pyroptosis caused by microvascular reperfusion injury by regulating the NLRP3 pathway [23]. Here, the study revealed that GAS could serve as a TBI therapy drug through targeting NLRP3 inflammasome pathway.
In fact, several drugs could relieve TBI through different manners. Dexmedetomidine alleviated early brain injury following traumatic brain injury by suppressing autophagy and neuroinflammation through inhibiting ROS/Nrf2 pathway [24]. In addition, Rhamnazin ameliorates TBI in mice via reducing apoptosis, oxidative stress, and inflammation [25]. These studies, together with our findings, showed promising therapeutic manners for TBI.
In conclusion, we found Gastrodin reduced brain tissue injury and improved functional recovery of injury nerve in TBI rats, and further alleviated inflammation. Gastrodin decreased the level of pyroptosis in brain tissue of TBI rats. In addition, we found GAS suppressed NLRP3 inflammasome-related signaling pathway, and therefore suppressed pyroptosis and exerted neuroprotective effects. GAS could therefore serve as a promising drug for TBI treatment.
TBI, Traumatic brain injury; GAS, Gastrodin; BBB, blood-brain barrier;
A
FY designed the study, supervised the data collection, GL analyzed the data, BL interpreted the data, KZ prepare the manuscript for publication and reviewed the draft of the manuscript. All authors have read and approved the manuscript.
All the animal experiments in this study were performed with the approval of the Ethics Committee of the First People’s Hospital of Hefei (2018-005-41).
We thank the reviewers for excellent criticism of the article.
This research received no external funding.
The authors declare no conflict of interest.