Academic Editor: Michael J. Spivey
Functional transcranial Doppler sonography (fTCD) is a time- and cost-effective, non-invasive approach to determining real time hemispheric lateralization and is well-suited for repetitive study designs comprising multiple days. To date, no study has examined the reproducibility of the direction and degree (strength) of lateralization during word fluency (WF) over multiple, consecutive sessions within a single person, although there are many studies of lateralization during language processing. Moreover, there is conflicting evidence as to whether there is a relationship between the degree of laterality and the word fluency performance. In this study, one right-handed male (aged 24 years) completed a total of seven examination sessions in the time span of 10 days. Each session comprised multiple phonological and semantic WF tasks. The maximum difference of relative cerebral blood flow velocity (CBFV) changes between the left and right middle cerebral artery (MCA) during WF was defined as the Lateralization Index (LI). The word-fluency performance and the LIs were used in a linear regression model to detect relative changes in the direction and degree of lateralization during repetitive WF tasks. The reproducibility of the direction of language-related lateralization is very stable over multiple sessions within this single person and the processed LIs were left-lateralized in every session for both WF tasks. In addition, performance during phonological WF could significantly predict the variability in the degree of lateralization. This result could not be confirmed for the semantic WF task. The results of this pilot study support the usage of fTCD as a reliable method for examining lateralization patterns, especially in longitudinal study designs. They also provide evidence for the notion that performance in WF tasks can be related to the degree of lateralization, at least intra-individually.
Most of the higher cognitive functions such as language processing and production are lateralized to one of the two hemispheres. To visualize these asymmetries, different neurophysiological methods and imaging techniques such as electroencephalography (EEG) or functional magnetic resonance imaging (fMRI) can be used [1, 2, 3, 4]. Since many of these examination methods require high effort and expenses, more easy-to-apply, non-invasive and cost-effective real time methods as the functional transcranial Doppler sonography (fTCD) have emerged. fTCD shows high correlations with lateralization patterns measured by fMRI [5, 6, 7, 8] or the Wada-Test [9] and is well suited for larger samples or study designs with multiple sessions [10]. It is currently applied in the examination of lateralization patterns in children [11, 12, 13] and adults [14, 15, 16, 17]. Additionally, it is applied in examining other cognitive functions, i.e., arithmetic [18] or spatial skills [19, 20]. Besides healthy subjects, it is used as a non-invasive diagnostic approach in clinical populations, i.e., children born deaf or with brain damage of different etiology [21, 22] as well as patients with epilepsy [23] or Parkinson’s Disease [24]. Furthermore, it is also an alternative for use in populations where fMRI examinations are impossible due to technical or medical reasons, such as metal implants, movement artifacts or, i.e., anxiety disorders. Since the mobile application of the fTCD allows for free head movement and speaking, it provides even more value in very young or uncooperative patients [25].
In fTCD, two ultrasound probes positioned on both temporal bones (Os temporale) simultaneously monitor the event-related changes in cerebral blood flow velocities (CBFV) in both middle cerebral arteries (MCA, Fig. 1). There is a high reproducibility of blood flow-velocity values in healthy individuals and patients with carotid artery stenosis for within-day as well as between-day measures [26], making it a reliable diagnostic tool. In general, fTCD can be used for detecting blood flow anomalies in cardiovascular diagnostics [27], but can also detect lateralization patterns during specific cognitive tasks [12]. According to Deppe et al. [5], velocities can be calculated via the formula:
where V(t) is the CBFV over time and V
where
is the difference between the relative velocity changes of the left and right
MCA. The time point t
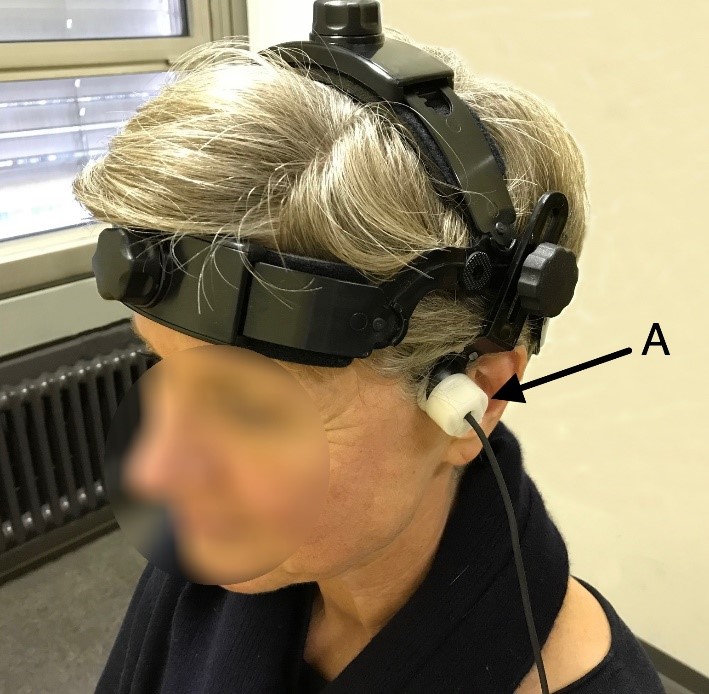
Application of the ultrasound probes during fTCD. Shown is the left ultrasound probe (A), which is connected to the TCD device.
When determining which language tasks are suitable for reliable fTCD results, research suggests that expressive language functions, i.e., word and sentence production, are generally stronger lateralized than their receptive counterparts, i.e., auditive discrimination/identification [29, 30, 31]. In language production, fTCD and fMRI studies reveal that lexical-semantic tasks show a stronger degree of lateralization than syntactic ones [2, 32, 33]. An often-used paradigm delivering reproducible patterns in Lateralization Indices comprises phonological and semantic word fluency tasks [14, 34]. In a phonological WF task, the participant is asked to find as many words beginning with a presented letter within a specific time span. In the semantic WF task, a semantic category is presented and the participants have to find as many words as possible matching the respective category. Both can be performed in a covert setting, which is currently the gold standard, in which a silent word-finding phase is followed by an overt reporting phase [35], and in an overt setting with immediate, open word production. The direction and degree of lateralization do not differ between these two conditions [36]. Unsworth et al. [37] attempted to model the cognitive processes underlying both WF tasks and described working memory capacity and vocabulary size as predictors of subsequent WF performance. Concerning the cerebral blood flow pattern as a neurophysiological correlate for these different underlying processes, recent findings by Gourovitch et al. [38] which used WF tasks during PET indicated that phonological WF led to increased levels of CBFV in the inferior frontal and temporoparietal cortices. Semantic WF was associated with increased CBFV in the left temporal cortex when the two WF tasks were compared directly. The anterior cingulate cortex, the left prefrontal regions, the thalamus, and the cerebellum showed comparatively increased CBFV values in both tasks.
Still, study designs with both WF tasks and repetitive follow-up measurements are rare. Knecht et al. [10] found a high test-retest reliability in healthy individuals for hour up to 14 months after the initial examination (r = 0.95; p = 0.0001) for the direction and degree of the LI during a phonological WF task. Additionally, Stroobant et al. [39] described a significant test-retest reliability (r = 0.61 to 0.83) for lateralization patterns of multiple tasks. This allows for reliably detecting shifts in task-related hemispheric perfusion exceeding 1% of the mean CBFV making them a valid source for longitudinal study designs. However, both studies only provided one follow-up measurement at different intervals relative to the first examination for each participant, lacking comparability and leaving open how changes in WF performance would alter the degree of the LI in a more continuous study design. Similarly, Woodhead et al. [17] also found a high stability of mean LI values over different language tasks, but again only used two measuring points. In addition, there was a lack of information on whether performance during these tasks affected the degree of language lateralization. In an approach to studying this effect, other authors found no correlation between the performance in covert WF tasks and the degree of lateralization measured by the LI in healthy adults [32, 40, 41], but found a positive correlation between the number of words produced during phonological WF in an overt condition [36]. However, they did not use follow-up measurements to evaluate the stability of their findings. Other authors tried to examine the correlation between task difficulty and the degree of language lateralization for several different language tasks, but found contradicting results ranging from the correlation of the LI values across several language functions [22, 29, 42] to significant differences across different language tasks [30, 43]. Lohmann et al. [44] performed a study with ten sessions of parallel fMRI and fTCD and found no trend in the lateralization pattern with a consistent LI during fTCD, but a decreasing degree of language lateralization over the sessions during fMRI.
Complementing the aforementioned findings, the current pilot study investigates (1) the reproducibility of the direction and degree of LI measurements over seven consecutive examinations in a single person using fTCD. (2) WF performance is assessed in both a phonological and a semantic WF task with multiple items per session to assess the impact of changes in WF performance on the direction and degree of language lateralization. Therefore, we need to test the following hypotheses: (1) at each of the seven sessions (over a short period of time) a consistent direction of lateralization of the measured LI should be found. (2) WF performance is positively correlated with the degree of the LI.
A male student of Bielefeld University, a native German speaker, 24 years of age and right-handed with a lateralization quotient of 80 according to the Edinburgh Handedness Inventory [45] took part in the experiment. The participant had corrected-to-normal vision and did not chronically take any medication in the last seven days before the experiment. He was paid for his participation. This study was approved by the ethics committee of Bielefeld University (ethics approval No. 2021-028). Written consent regarding data collection and publication was obtained from the participant.
Five items for phonological and five items for the semantic word fluency task were selected as experimental stimuli. For the phonological WF task, the five items were the letters A, K, H, B and S. These are the five most frequent German initial letters (defined by total amount of words beginning with the aforementioned letters) according to the Wahrig-Brockhaus Lexikon of German Language [46]. For the semantic word fluency task, we used the five categories furniture, drinks, animals, electronic gadgets and diseases. They were chosen from a collection of semantic categories frequently used in German aphasia therapy or in children with semantic impairment (Memogym: Prolog). All semantic categories had to fit the criterion that a possible member of one category could not be assigned to any of the other categories. The stimuli were arranged and presented in Cogent2000, a MATLAB-based toolbox, which was installed on a PC (Windows XP). Before the presentation of each item, electric trigger signals were sent from the PC (Windows XP) to the fTCD-Computer (MultiDop T2, DWL, Singen, Germany) via a customized cable connecting the PC’s DB-25 parallel port to the fTCD-Computer’s Av-in-port to mark the beginning of each trial. The latter then recorded lateralization patterns extracted from the participants blood flow patterns in the left and right MCA.
The study consisted of seven examination sessions each lasting 30 minutes (15
minutes of probe positioning and 15 minutes of WF) over a ten-day period with
never more than one day of rest between sessions. A schematic representation of
the procedure is shown in Fig. 2, upper part. The participant was seated in front
of a computer monitor at a distance of 50 cm between the participant’s eyes and
the screen with no other applications on the table in front of him or on the
plain wall behind the screen. The letters and semantic categories for both WF
tasks were visually presented in a light grey serif-free font (Helvetica size 40)
on a black background on a 15” LCD Monitor. The visual angle between the
participants’ eyes and the center of the monitor, on which the WF items were
presented was 1.15
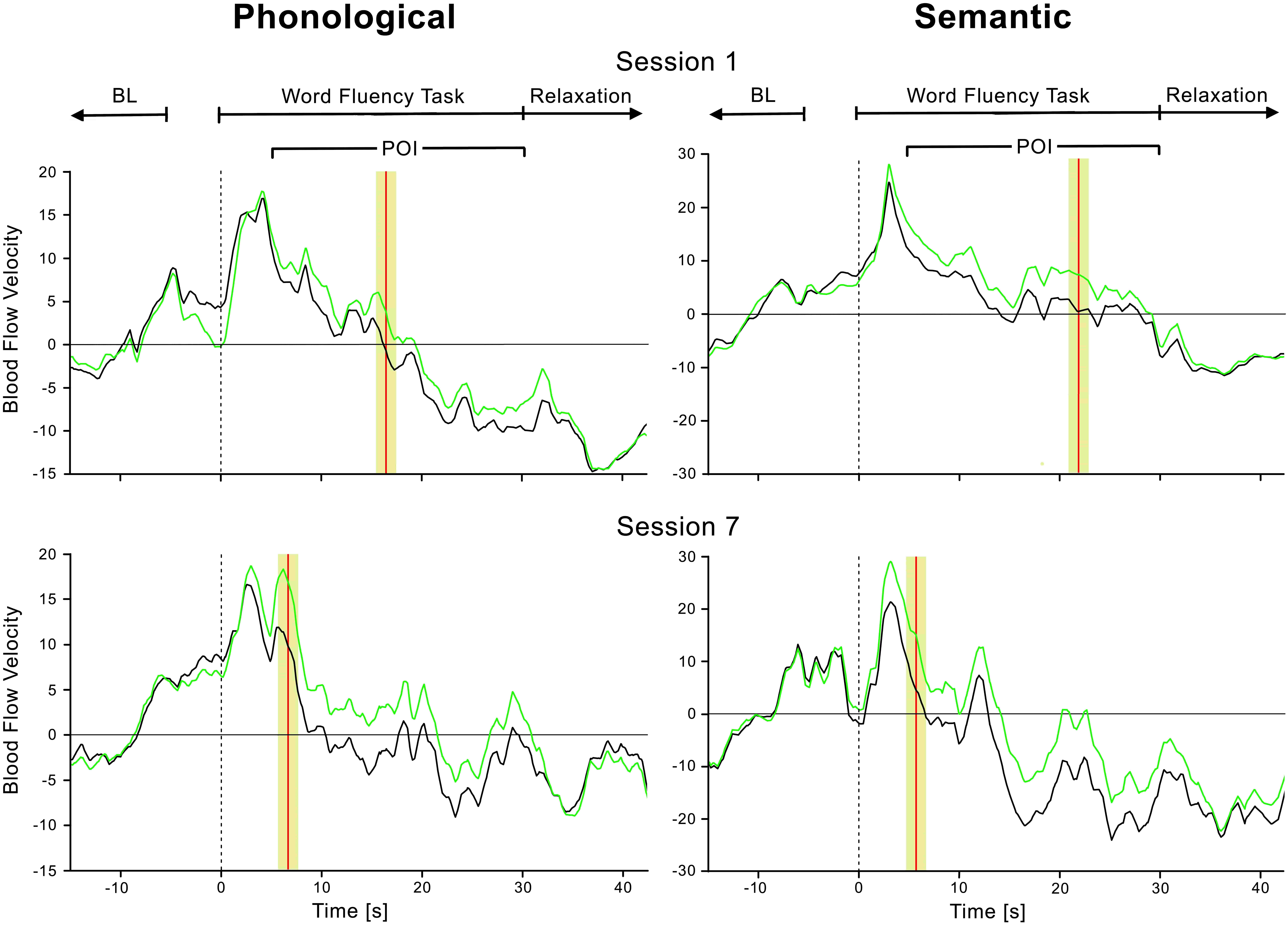
Mean blood flow velocities of the left (green) and right (black) middle cerebral arteries (MCA) during the phonological and semantic WF task of the first and seventh (last) session. The velocity values were normalized and averaged over all five consecutive WF trials. The item presentation and overt word production start at 0 s and last up to 30 s (x-axes). To account for the process of neuro-metabolic coupling, the period of interest (POI) begins 5 s after the item presentation. After a subsequent relaxation phase of 30 s, the next item is presented. The vertical red line indicates the largest difference between the CBFV values of both MCAs and the highlighted 2 s interval around this time point is the activation window, where the LI is calculated. A 10 s (from –15 to –5 s) interval before each item presentation is used as the baseline (BL) for rCBFV values.
For the fTCD measurement, the 2 MHz ultrasound probes on the mobile device were adjusted at an insonation depth of 52 mm in order to measure the CBFV in the M1 segments of both MCAs. Moreover, the detection sensitivity was set to 38%, standard Volume = 12, Output = 420 and Filter = 150 in the DWL Multidop QL Software Routine Version 2.5 (Singen, Germany).
Recorded fTCD data was analyzed using dopStep Master, which evolved from dopOSCCI, a Matlab (Mathworks, Natick, MA, USA) based software package [47]. Its programming is based on the software package AVERAGE [48] and can be used with various TCD devices and allows for subtle quantitative off-line analysis of Doppler flow signals. First, the channels for the left and right MCA as well as the trigger channel were set. The latter contains markers required to be stored in the data file in order to time-lock the task-related activity and are commonly sent via the parallel port. To prevent interference from involuntary cardiac events with the examination of the task-related signals, the activity within a single heart cycle was averaged, which resulted in a step-like summary of the activity as opposed to the natural variations in blood flow velocity during a heartbeat. Additionally, the time span between two event markers was set to 60 s and the range of blood flow was limited to 150 cm/s to exclude any measurement or movement artifacts. As probe angles might differ between the two sides [22], data from the left and right MCA are normalized to a mean of 100 using the following equation:
where data refers to a collection of blood flow velocity values. The epochs themselves were specified from –5 to 30 s relative to the event marker at 0 s (t0), with the time span from –15 to –5 s before t0 serving as the baseline CBFV. The period of interest was specified to begin 5 seconds after t0 and to last until 30 seconds relative to the event marker to consider the process of neuro-metabolic coupling. The activation window itself, which describes the time interval with the largest event-related changes in CBFV in both MCAs, indicates the time across which the LI will be calculated and was set to 2 seconds. The presented rCBFV values were averaged over all five phonological/semantic WF trials for each of the seven respective sessions. Since the participant completed five phonological and semantic WF trials in each of the seven sessions, we were able to compute the LI values on the basis of a total of 70 valid WF trials with the aforementioned time frame.
In a first step, we performed univariate one-way ANOVAs with session as factor and mean WF performance as the dependent variable to compare changes in WF performance across all sessions. In a second step, we tested our hypothesis that a consistent direction of lateralization would be found, and our second hypothesis that WF performance correlates positively with the degree of the LI. For this, we performed a linear regression with WF performance as a predictor and the LI values as the dependent variable.
The mean number of produced words by the participant during both WF tasks were
normally distributed for the phonological (Kolmogorov-Smirnov, d = 0.12,
p
Session | Phonological WF task | Semantic WF task | ||
M | SD | M | SD | |
1 | 11.6 | 0.55 | 12.6 | 2.30 |
2 | 11.4 | 1.14 | 13.8 | 2.17 |
3 | 10.4 | 0.89 | 14.4 | 1.95 |
4 | 12.6 | 1.14 | 15.6 | 4.22 |
5 | 12.6 | 2.19 | 17.0 | 4.00 |
6 | 13.2 | 1.30 | 20.0 | 2.35 |
7 | 14.2 | 1.92 | 17.2 | 4.82 |
We performed univariate one-way ANOVAs comparing the WF performance across all
sessions for each WF task. There was a main effect of the factor session (F(6,28)
= 3.99, p
Session | Session | Mean difference | t | p | p |
1 | 2 | 0.200 | 0.224 | 0.824 | 1.000 |
3 | 1.200 | 1.346 | 0.189 | 0.824 | |
4 | –1.000 | –1.122 | 0.271 | 0.916 | |
5 | –1.000 | –1.122 | 0.271 | 0.916 | |
6 | –1.600 | –1.795 | 0.083 | 0.562 | |
7 | –2.600 | –2.917 | 0.007 | 0.087 | |
2 | 3 | 1.000 | 1.122 | 0.271 | 0.916 |
4 | –1.200 | –1.346 | 0.189 | 0.824 | |
5 | –1.200 | –1.346 | 0.189 | 0.824 | |
6 | –1.800 | –2.020 | 0.053 | 0.425 | |
7 | –2.800 | –3.142 | 0.004 | 0.054 | |
3 | 4 | –2.200 | –2.469 | 0.020 | 0.209 |
5 | –2.200 | –2.469 | 0.020 | 0.209 | |
6 | –2.800 | –3.142 | 0.004 | 0.054 | |
7 | –3.800 | –4.264 | 0.003 | ||
4 | 5 | 0.000 | 0.000 | 1.000 | 1.000 |
6 | –0.600 | –0.673 | 0.506 | 0.993 | |
7 | –1.600 | –1.795 | 0.083 | 0.562 | |
5 | 6 | –0.600 | –0.673 | 0.506 | 0.993 |
7 | –1.600 | –1.795 | 0.083 | 0.562 | |
6 | 7 | –100.000 | –1.122 | 0.271 | 0.916 |
Session | Session | Mean difference | t | p | p |
1 | 2 | –1.200 | –0.5747 | 0.570 | 0.997 |
3 | –1.800 | –0.8620 | 0.396 | 0.975 | |
4 | –3.000 | –1.4367 | 0.162 | 0.778 | |
5 | –4.400 | –2.1072 | 0.044 | 0.376 | |
6 | –7.400 | –3.5440 | 0.001 | 0.021 | |
7 | –4.600 | –2.2030 | 0.036 | 0.326 | |
2 | 3 | –0.600 | –0.2873 | 0.776 | 1.000 |
4 | –1.800 | –0.8620 | 0.396 | 0.975 | |
5 | –3.200 | –1.5325 | 0.137 | 0.724 | |
6 | –6.200 | –2.9693 | 0.006 | 0.078 | |
7 | –3.400 | –1.6283 | 0.115 | 0.666 | |
3 | 4 | –1.200 | –0.5747 | 0.570 | 0.997 |
5 | –2.600 | –1.2452 | 0.223 | 0.870 | |
6 | –5.600 | –2.6819 | 0.012 | 0.140 | |
7 | –2.800 | –1.3410 | 0.191 | 0.827 | |
4 | 5 | –1.400 | –0.6705 | 0.508 | 0.993 |
6 | –4.400 | –2.1072 | 0.044 | 0.376 | |
7 | –1.600 | –0.7663 | 0.450 | 0.986 | |
5 | 6 | –3.000 | –1.4367 | 0.162 | 0.778 |
7 | –0.200 | –0.0958 | 0.924 | 1.000 | |
6 | 7 | 2.800 | 1.3410 | 0.191 | 0.827 |
The participants’ WF performance during the phonological WF task tended to increase from session to session and indicated a general learning effect during this task. In particular, the sixth and seventh sessions had a tendency to differ from the first three sessions. Exceptions were the first sessions, where the learning effect did not yet occur, and the fourth and fifth sessions, during which the same number of words were produced. In the semantic WF task, the performance in the sixth session was slightly better than the first four. The participant also showed a general learning effect on this task with an improvement in mean word production, although he produced fewer words in the last session than in the sixth session. Presumably, since only five values per session were included in the analysis, most of the individual comparisons did not reach the level of significance after the Tukey correction.
In addition, the participant demonstrated a robust, left-lateralized pattern for both WF tasks in all seven sessions, although the degree of language lateralization varies. The left-lateralized pattern is indicated by the positive LI values in Table 4 and results from higher mean blood flow velocity in the left compared to the right MCA. A somewhat unclear finding is that sessions 3 and 5 showed strongly lateralized LI values which, according to LI calculations with dopStep Master [47], were not significantly lateralized. Since no artifacts were found in the analysis this result is likely due to normal variation in the Doppler signal within one individual.
Session | LI phon | SD | LI sem | SD |
1 | 4.05 | 2.36 | 6.17* | 0.52 |
2 | 3.74* | 1.34 | 6.45* | 0.46 |
3 | 4.01 | 0.24 | 5.50* | 0.94 |
4 | 4.65* | 1.45 | 2.14 | 1.35 |
5 | 2.03 | 0.89 | 2.76 | 1.45 |
6 | 7.23* | 0.68 | 4.52* | 0.79 |
7 | 6.54* | 0.78 | 8.60* | 1.62 |
Fig. 2 shows the difference in the mean blood flow velocities of the left and right MCA during the phonological and semantic WF task for the first and the last session. In both presented sessions, the participant shows a higher mean blood flow velocity in the left hemisphere 2 to 3 seconds after the start of the WF-Task (item presentation), which exceeded that of the right hemisphere until about 5 s after the WF task ended. In the last session, we observed an earlier differentiation of the CBFV values compared to the first, which already took place in the first few seconds and thus within the period of the task-related neuro-metabolic coupling. This may be due to an anticipation effect which could reduce the reliability of LI values in the last session compared to the first session somewhat.
To determine whether these changes in WF performance might affect language
lateralization in this individual, we conducted a linear regression with the mean
WF performance as predictor and the LI values as the dependent variable. Data
were normally distributed for the phonological WF task (Kolmogorov-Smirnov, d =
0.148, p
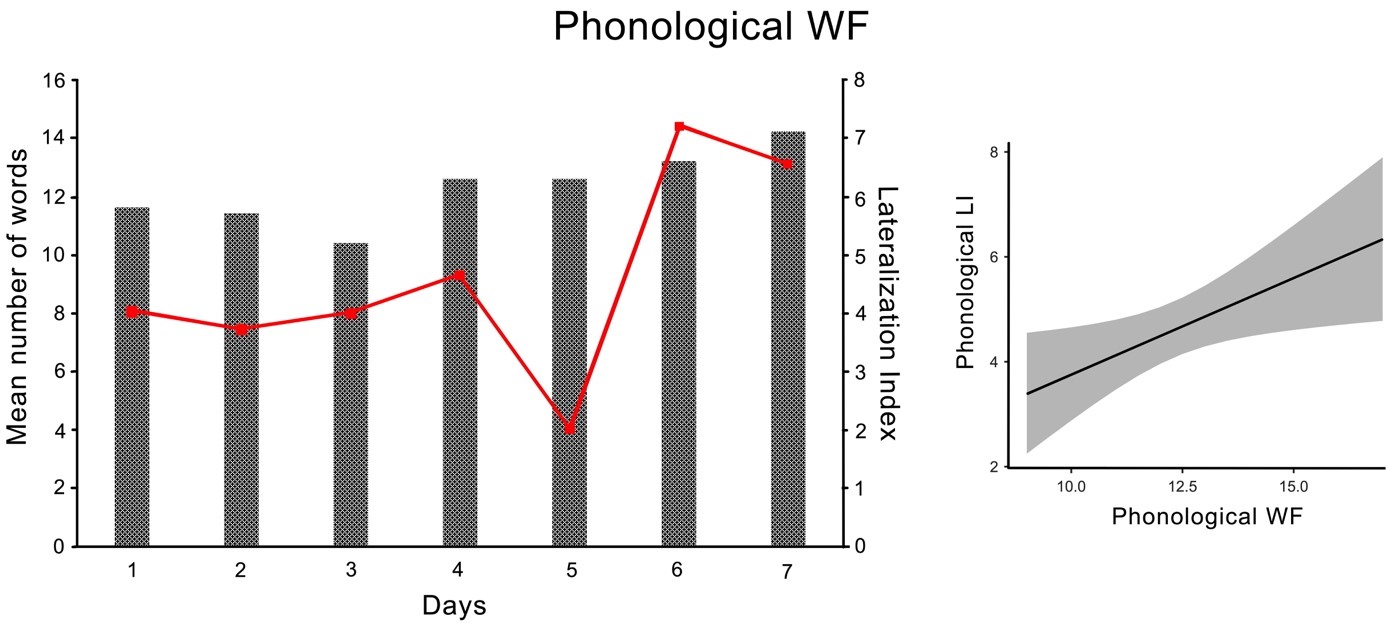
Phonological Word Fluency Task. Left panel: average number of produced words (columns) and Lateralization Index (line graph) in the phonological WF task for each session. Right panel: Estimated marginal means of the regression between WF performance (x-axis) and the degree of language lateralization as indicated by the LI (y-axis) for the phonological task.
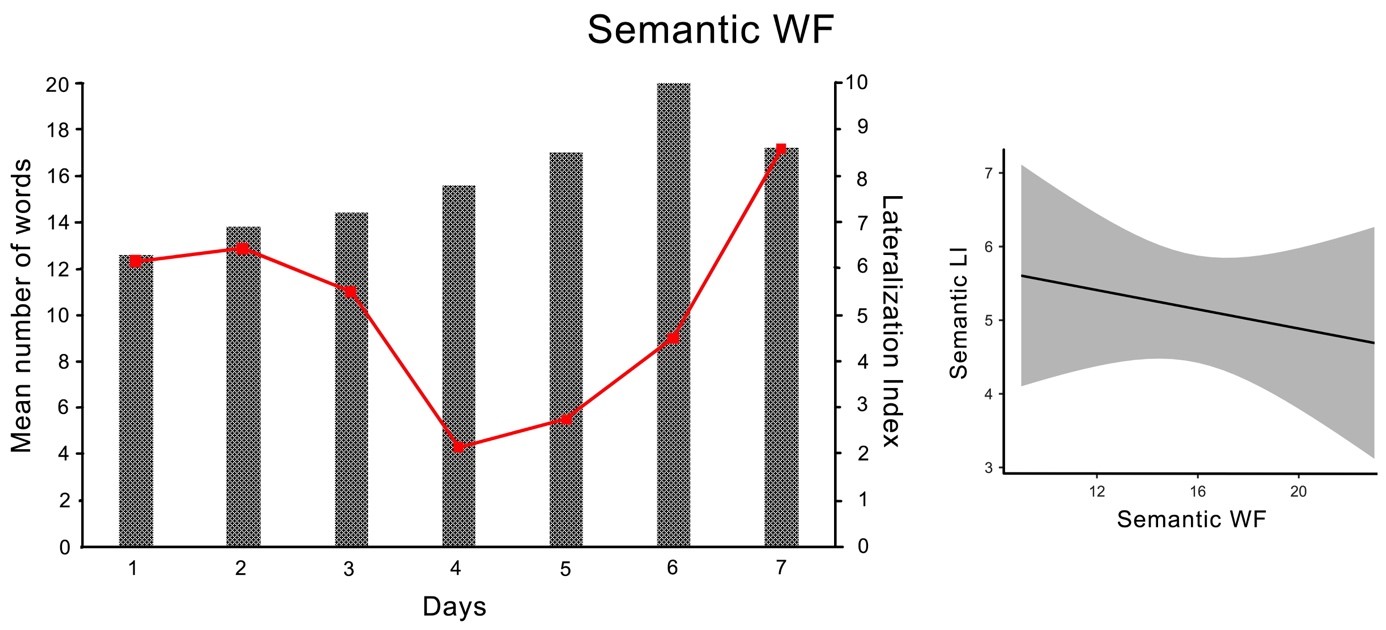
Semantic Word Fluency Task. Left panel: average number of produced words (columns) and Lateralization Index (line graph) in the semantic WF task for each session. Right panel: Estimated marginal means of the regression between WF performance (x-axis) and the degree of language lateralization as indicated by the LI (y-axis) for the semantic task. Although semantic WF could not predict the corresponding LI, there may be a negative trend in the correlation of the data.
Our main research question was whether fTCD can provide reproducible results in an individual regarding the direction and degree of language-related lateralization over multiple follow-up examinations within a ten-day period. This reproducibility was investigated using phonological and semantic WF tasks. It was hypothesized that a left lateralized LI should be found at each of the seven sessions and that WF performance correlates with the degree of the LIs.
In concordance with Knecht et al. [10], we found a highly reproducible direction of hemispheric lateralization towards the left hemisphere in each of the seven examination sessions for both WF tasks in this individual. However, the degree of the lateralization varied considerably in both tasks. The calculation of a linear regression showed that variations in the individual’s WF performance predict the variable degrees of LI values, at least in the phonological task. This could indicate that although the direction of language lateralization shows high stability, positive as well as negative shifts in phonological WF performance can lead to a corresponding variability in LI values. This would lead to the assumption that the degree of lateralization is not a static construct for a person, but instead fluctuates depending on the task performance. In contrast, the LI scores could not be predicted by the semantic WF performance in our study because the linear regression showed no statistical significance. Possibly, the semantic fluency task is more complex than the phonological fluency task [34], allowing for a greater cognitive load which could explain the greater variability in the data of this single subject.
A further possible explanation for these findings could be the difference in underlying cerebral blood flow patterns during word generation between the two tasks. Although there is an increase in CBFV patterns in certain cortical, subcortical and cerebellar areas, phonological WF is associated with increased CBFV values in the inferior frontal cortex and temporoparietal cortex. In contrast, during semantic WF, the patterns of increased CBFV shift more towards the left temporal cortex [38]. This could be a result of other, partly non-linguistic cognitive processes (i.e., using mental images, semantic features). Although Unsworth et al. [37] found no significant differences in the influence of the underlying cognitive processes on WF performance, the distinct regional cerebral blood flow patterns may explain the measured differences in the strength of the correlation between WF performance and the degree of lateralization. Further research is needed to better understand the cognitive mechanisms underlying these WF tasks and their interconnection between regional cerebral blood flow patterns. Nevertheless, our results may indicate that changes in word production, at least when mainly phonological tasks are solved, are associated with the degree of language lateralization.
Concerning the quantitative performance in word production, during the phonological WF tasks, the participant produced more words in the last session compared to the first three, and there is an outlier in WF performance during the third session which is significantly lower compared to the remaining four sessions. A similar improvement was also measured in the semantic WF task, in which the participant’s WF performance was significantly better in the sixth session compared to the first four and decreased again in the last session. This indicates that repetitive training of both WF tasks in healthy adults results in a practice effect.
In our study, the courses of mean word production and LI values are clearly related in this individual during the phonological WF tasks. While these results may not hold true for the entire population, especially left-handed and ambidextrous persons, they point out that the lack of correlation between these two parameters described by Lust et al. [41] could be a result of their just one-day study design. The results may be different in elderly populations or in patients with underlying neurological diseases and disorders. Comparing these results from a young, male participant with persons showing a different handedness and with different age groups will be a subject further research will have to address. In addition, future studies will need to be conducted in a larger sample with comparable multi-session measurements to further substantiate our results.
To sum up, the results of our study support the suitability of fTCD as a tool to reliably identify patterns of lateralization and to illustrate correlations between cerebral lateralization patterns over multiple, consecutive sessions and performance in WF tasks in a single person. In terms of the reproducibility of the language-related lateralization patterns of this young male participant, this study confirms that fTCD is a viable alternative to fMRI examinations when temporal resolution of perfusion patterns is more of concern than spatial resolution, or when a cost-effective alternative is required.
In this pilot study, we measured the reliability of the reproducibility of lateralization patterns in a young, healthy adult during phonological and semantic WF tasks. We found a stable, left-lateralized pattern of LI values and a significant effect showing that LI values fluctuate depending on task performance during phonological WF in this individual. Further studies with larger samples are needed to compute a reliable model that provides better insight into the relationship between word production and lateralization patterns and clarifies differences in the phonological and semantic WF. The high stability of the direction of the measured language lateralization across multiple different sessions supports the assumption that fTCD is well suited for study designs over longer periods of time or for studies with less cooperative participants such as children or patients with dementia.
All authors, FH, SW, HM contributed to the study conception and design. FH conducted the fTCD measurements and drafted the manuscript. All authors, FH, SW, HM analyzed the data and revised the manuscript. All authors, FH, SW, HM read and approved the final manuscript.
The underlying study was approved by the ethics committee at Bielefeld University (ethics approval No. 2021-028). Written consent regarding data collection and publication was obtained from the participant. A copy of the consent form for review is available from the author FH upon request.
We would like to acknowledge the consistent support by Nicholas A. Badcock (Macquarie University, Sydney, Australia) in the adaptation and usage of the dopStep Master toolbox. We thank the anonymous reviewers for the very helpful comments on the first draft of the manuscript.
This study was supported by the “German Research Foundation” (Cluster of Excellence “Cognitive Interaction Technology”, EC 277).
The authors declare no conflict of interest.