†These authors contributed equally.
Stroke is a leading cause of death and disability world-widely. The incidence rate of stroke has been increasing due to the aging population and lifestyle changes. At present, the only drug approved by the US Food and Drug Administration (FDA) for the treatment of ischemic stroke is tissue plasminogen activator (t-PA), but its clinical application is greatly limited because of its narrow time window and bleeding risk. Natural products have a long history of being used in traditional medicine with good safety, making them an important resource for the development of new drugs. Indeed, some natural products can target a variety of pathophysiological processes related to stroke, including oxidative stress, inflammation and neuronal apoptosis. Therefore, the development of high-efficiency, low-toxicity, safe and cheap active substances from natural products is of great significance for improving the treatment alternatives of patients with stroke. This article reviews the neuroprotective effects of 33 natural compounds by searching recent related literature. Among them, puerarin, pinocembrin, quercetin, epigallocatechin-3-gallate (EGCG), and resveratrol have great potential in the clinical treatment of ischemic stroke. This review will provide a powerful reference for screening natural compounds with potential clinical application value in ischemic stroke or synthesizing new neuroprotective agents with natural compounds as lead compounds.
Stroke is a major cause of death and disability in the world, often occurring in elderly patients [1]. There are two main types of stroke: ischemic (IS) and hemorrhagic stroke (HS). Among these, IS accounts for 80% of all cases of stroke and is caused by a blood clot in a cerebral artery. HS can be divided into intracerebral hemorrhage and subarachnoid hemorrhage and accounting for approximately 20% of the total number of stroke cases [2, 3]. With unhealthy dietary patterns, increased prevalence of high blood pressure and an aging population, the incidence rate of stroke has been increasing over recent years, bringing a heavy burden to society, families and individuals, therefore becoming a global public health issue [4, 5].
At present, the two therapeutic strategies for stroke are reperfusion and neuroprotection [6]. Reperfusion includes mechanical thrombectomy and thrombolytic therapy. However, due to this short time window of reperfusion therapy, most patients do not receive effective treatment in time. In addition, thrombolytic therapy also has the risk of reperfusion injury and bleeding, with poor long-term efficacy [7, 8]. The development of neuroprotective drugs mainly includes calcium channel antagonists, free radical scavengers, glutamate antagonists and cell membrane stabilizers, all of which are currently in the experimental stage [9]. Therefore, the characterization of novel drugs that protect against ischemic and neuronal damage is an important task at present.
Natural products have received extensive attention in recent years as a valuable resource bank for drug discovery due to their rich resources and long history of clinical application [10]. Indeed, many natural compounds against stroke have been found. They have a significant role in the early intervention of stroke, reducing adverse reactions, thus becoming potentially effective for the prevention and treatment of stroke [11, 12]. Some natural compounds can simultaneously target multiple cell signaling pathways to regulate cell function. Considering the complexity of stroke pathophysiology, multi-target strategy should be more effective for the treatment of ischemic stroke [13]. In this paper, the mechanism of different natural products in the treatment of stroke was reviewed to provide a reference for further development of drugs for the prevention and treatment of stroke.
A systematic literature search through 2000–2021 was performed in Google Scholar, PubMed, and a Scientific Direct Database to identify relevant studies. We used natural compounds, phytochemicals, polyphenols, flavonoids, quercetin, kaempferol, curcumin and other search terms in the full text options, and combined stroke, cerebrovascular accident, cerebral ischemia, and ischemic stroke. The titles and abstracts were scanned to exclude any studies that were clearly irrelevant. We read the full texts of the remaining articles and classified the compounds according to their chemical structures.
Stroke is a series of complicated pathophysiological reactions caused by ischemia and hypoxia. It is currently believed that energy metabolism disorders, cytotoxic effects of excitatory amino acids, oxidative stress injury, inflammation and apoptosis are the main mechanisms that cause neuronal death in focal ischemic areas of the brain and lead to IS (Fig. 1) [14].
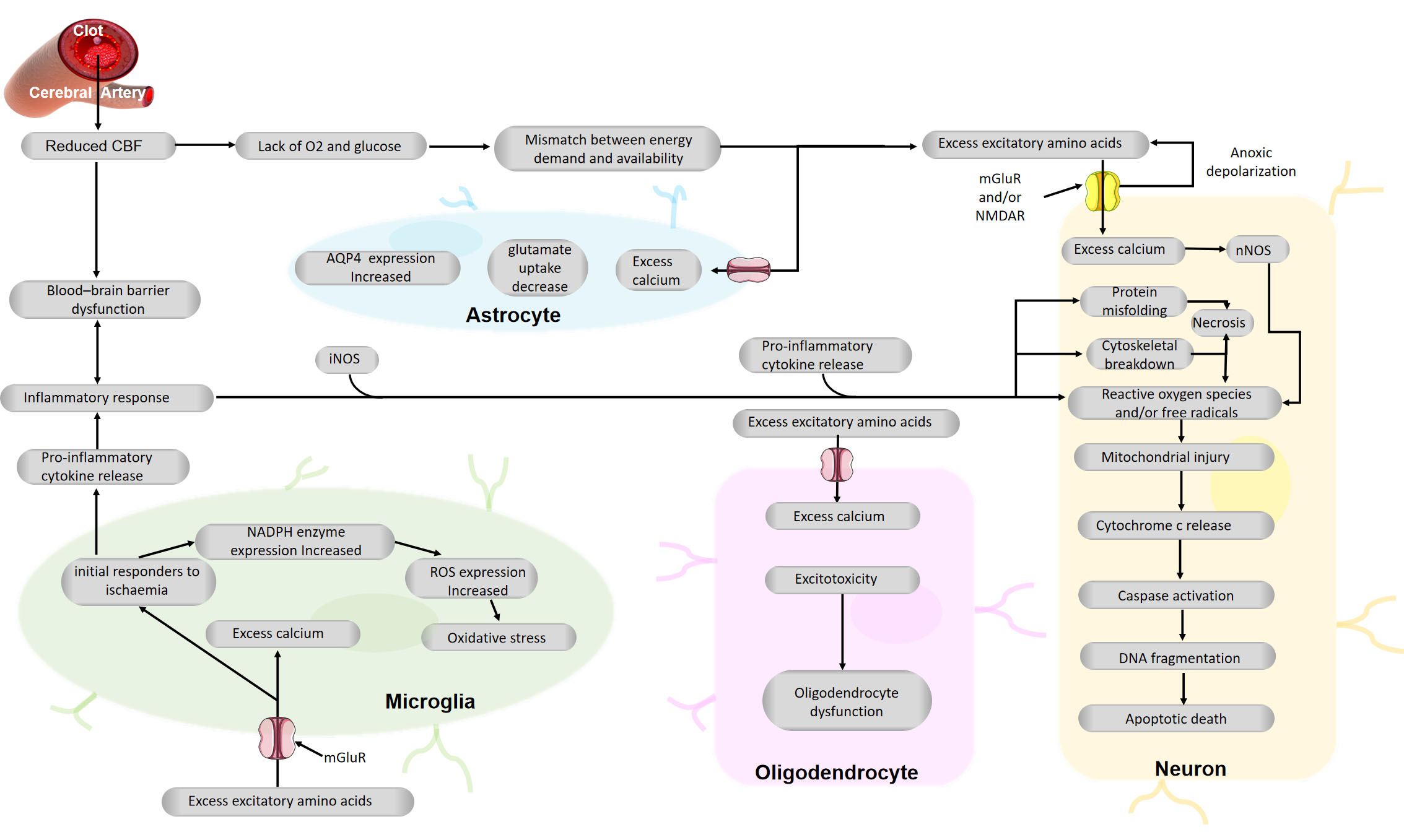
Pathophysiology of stroke.
Cerebral artery occlusion leads to the decrease of cerebral blood flow, the insufficiency of oxygen and glucose supply, the disorder of energy metabolism in the brain, and the mismatch between energy requirements and availability of neurons, glial cells and endothelial cells. Anoxic depolarization and decreased glutamate reuptake activity lead to the increase of extracellular glutamate level, activation of glutamate receptor, promotion of the influx of calcium ions into cells, mitochondrial dysfunction and a series of signal events such as the activation of free radicals and proteases, and neuronal apoptosis. This process has been termed excitotoxicity. Excessive extracellular excitatory amino acids can inhibit the absorption of cysteine by oligodendrocytes, resulting in dysfunction of oligodendrocytes. Blood-brain barrier dysfunction and release of signaling molecules (for example, cytokines) from microglia lead to an inflammatory response. Inflammatory response promotes the release of inducible nitric oxide synthase (iNOS) from neutrophils, leading to the increase of nitric oxide level. Activated microglia can increase the expression of NADPH oxidase, induce ROS production, cause oxidative stress and further damage.
The disturbance of energy metabolism in the brain is a direct factor that
contributes to brain injury in the early stages of IS. The brain tissue is the
most oxygen-consuming in the body, therefore ischemia can easily disturb energy
metabolism, reduce blood flow, limit the supply of oxygen and glucose, and reduce
the production of ATP in mitochondria [15, 16]. ATP deficiency leads to the
inhibition of ATP-dependent Na
Glutamic acid (Glu) and aspartic acid (Asp) are the two main excitatory amino acid transmitters in the central nervous system, of which Glu is the most abundant in the brain and is mainly distributed in the cortex and hippocampus [18, 19]. When the blood supply to the brain is insufficient, Glu is released in large quantities from presynaptic nerve endings, decreasing the ability of neurons and astrocytes to absorb Glu, which will disrupt the dynamic balance of Glu synthesis and decomposition, uptake and release. Glu accumulates in the synaptic space and over activates its receptor, which can cause a series of reactions such as calcium overload, increased production of nitric oxide, and generation of free radicals, which are highly toxic to the central nervous system, exerting an improvisational neurotoxic effect, namely excitotoxic effects [20, 21]. In addition, excessive extracellular EAA can inhibit the absorption of cysteines by oligodendrocytes, thereby reducing the synthesis of glutathione and damaging to oligodendrocytes [22].
Upon cerebral ischemia, the mitochondrial respiratory chain is damaged and ATP
synthesis is reduced, which is accompanied by the excessive generation of
reactive oxygen species (ROS) and reactive nitrogen species
(RNS) [23]. In addition, activated microglia can increase the expression of
nicotinamide adenine dinucleotide phosphate (NADPH) oxidases, inducing the
generation of ROS [24, 25]. ROS has been reported to accumulate in ischemic
tissue; its strong oxidation ability leads to the peroxidation of lipids,
proteins and unsaturated fatty acids on the basement membrane and destroys the
blood-brain barrier (BBB), ultimately causing irreversible damages on nucleic
acid and carbohydrate metabolism [26, 27]. Oxidative stress also indirectly
affects the permeability of the BBB by promoting the cytoskeleton reorganization,
regulating the expression and distribution of tight junction proteins, affecting
the expression and activity of matrix metalloproteinases, and promoting the
synthesis of inflammatory factors. In the ischemic core, severe oxidative stress
can lead to cell necrosis by degrading key cell components, while in the ischemic
penumbra, oxidative stress activates NF-
The inflammatory response after cerebral ischemia plays an important role in the
occurrence and development of IS, and a variety of immune cells and inflammatory
cytokines participate in this process. During cerebral ischemia, activated
astrocytes and microglia produce tumor necrosis factor-
Within a few hours after cerebral ischemia, dysfunctional energy metabolism,
excitotoxicity of EAA, free radical damage and inflammatory immune effects will
eventually induce neuronal cell death or apoptosis around the infarcted area or
ischemic penumbra [33]. According to different forms of activation, apoptosis can
be divided into the exogenous death receptor signaling pathway and endogenous
signaling pathway, involving the activation of a variety of genes and proteins.
In the endogenous pathway, the protein Bax forms oligomeric channels on the
mitochondrial membrane, leading to the outflow of cytochrome c, which activates
caspase 3, and ultimately removes cytoskeletal proteins and induces DNA cleavage
[34, 35, 36]. As an important tumor suppressor protein and transcription factor, p53
can directly induce the expression of apoptotic proteins and genes under ischemia
and hypoxia [37]. Neuronal death caused by ischemia commonly results in cell
necrosis and apoptosis. Lack of oxygen induces the opening of calcium channels,
and this large influx of Ca
The blood-brain barrier (BBB) plays a vital role in regulating the trafficking of fluid, solutes and cells at the blood-brain interface and maintaining the homeostatic microenvironment of the CNS [40]. Pathological mechanisms such as inflammation and oxidative stress caused by cerebral ischemia and hypoxia can change the permeability of the BBB, resulting in the permeability towards water molecules and ions, which can form brain edema [41]. In addition, the expression of aquaporin 4 (AQP4) on the astrocyte foot process is up-regulated after cerebral ischemia-reperfusion, which can also allow excess water to accumulate in the brain [42, 43]. As a serious complication of stroke, cerebral edema can lead to higher intracranial pressure, compression of brain tissue, obstruction of cerebrospinal fluid circulation and blood flow, aggravating ischemia and edema, thus forming a vicious cycle [44, 45].
Many natural compounds that can treat stroke have been well studied (Figs. 2 (Ref. [46, 47, 48, 49, 50, 51, 52, 53, 54, 55, 56, 57, 58, 59, 60, 61, 62, 63, 64, 65, 66, 67, 68, 69, 70, 71, 72, 73, 74, 75, 76, 77, 78, 79, 80, 81, 82, 83, 84, 85, 86, 87, 88, 89, 90, 91]), 3 (Ref. [92, 93, 94, 95, 96]), 4 (Ref. [97, 98, 99, 100, 101]), 5 (Ref. [102, 103, 104]), 6 (Ref. [105, 106]), 7 (Ref. [107, 108, 109, 110, 111])). We reviewed and summarized them for future drug discovery and application (Table 1, Ref. [46, 49, 52, 53, 54, 56, 58, 59, 60, 61, 62, 63, 66, 68, 69, 70, 72, 73, 74, 76, 77, 79, 80, 81, 83, 85, 87, 90, 91, 94, 95, 96, 97, 98, 100, 102, 103, 104, 105, 106, 107, 108, 109, 110, 111]).
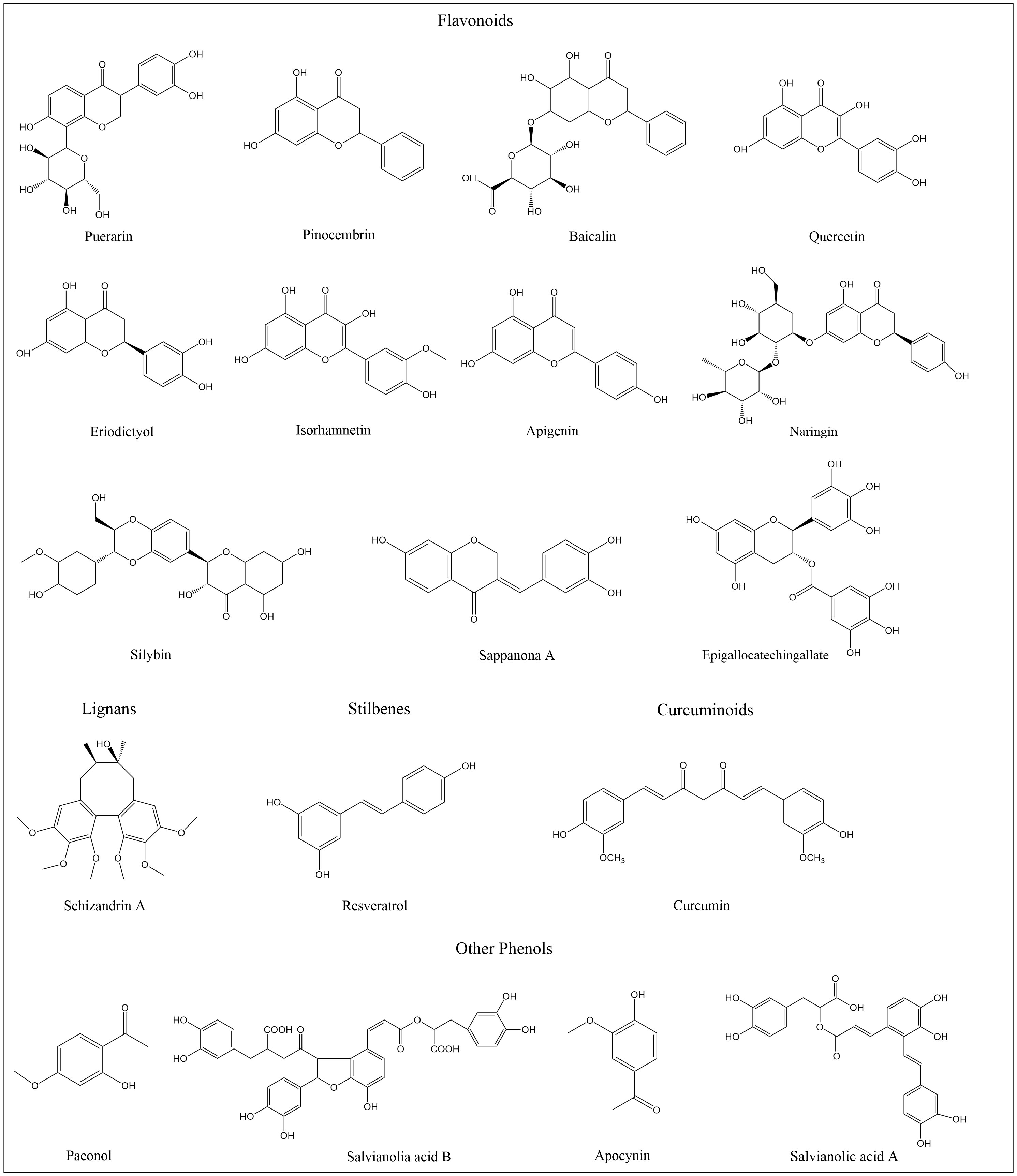
Chemical structures of some representative neuroprotective phenols (Ref. [46, 47, 48, 49, 50, 51, 52, 53, 54, 55, 56, 57, 58, 59, 60, 61, 62, 63, 64, 65, 66, 67, 68, 69, 70, 71, 72, 73, 74, 75, 76, 77, 78, 79, 80, 81, 82, 83, 84, 85, 86, 87, 88, 89, 90, 91]).
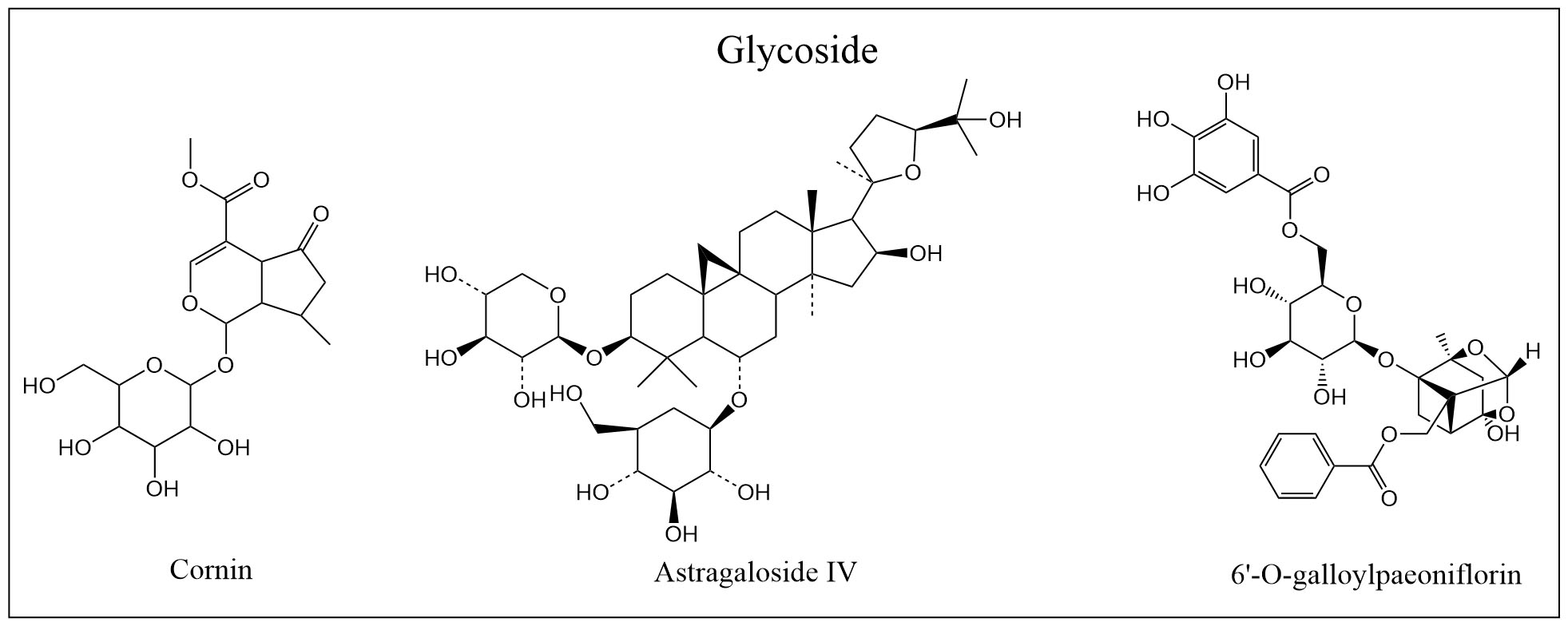
Chemical structures of some representative neuroprotective glycosides (Ref. [92, 93, 94, 95, 96]).
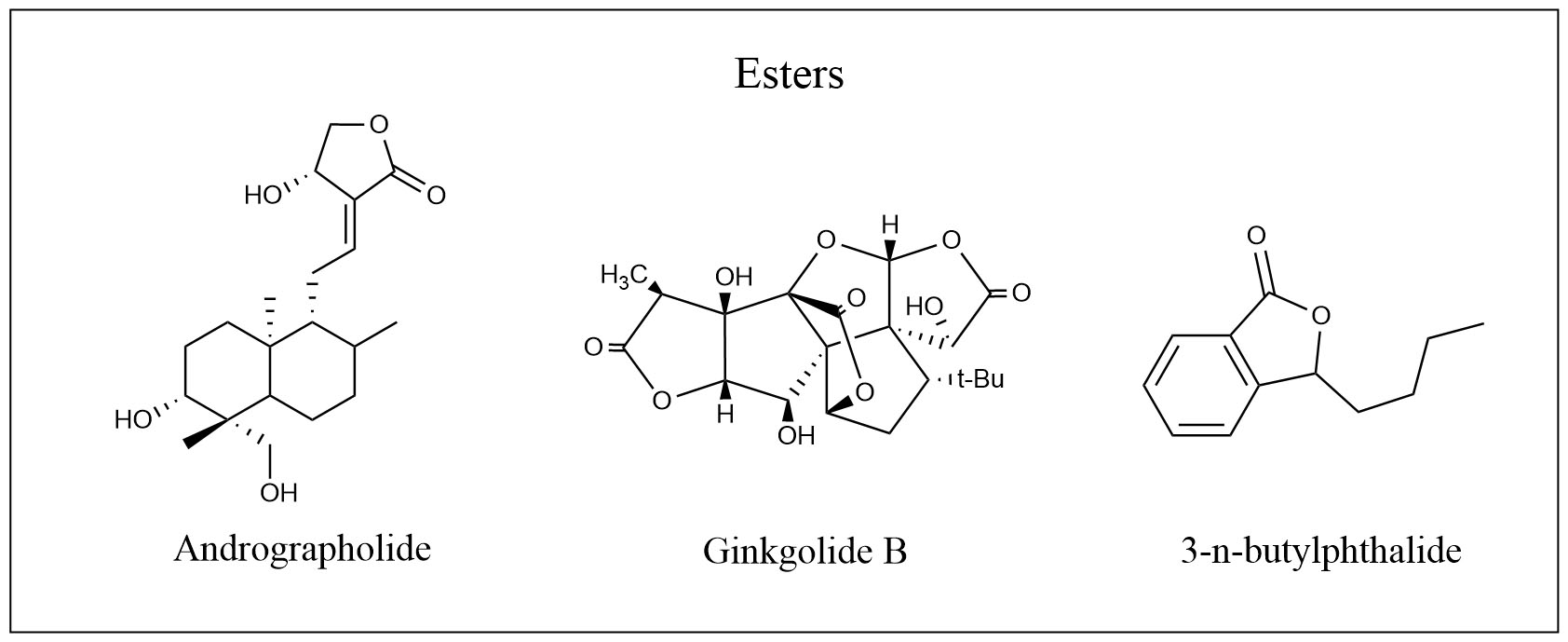
Chemical structures of some representative neuroprotective esters (Ref. [97, 98, 99, 100, 101]).
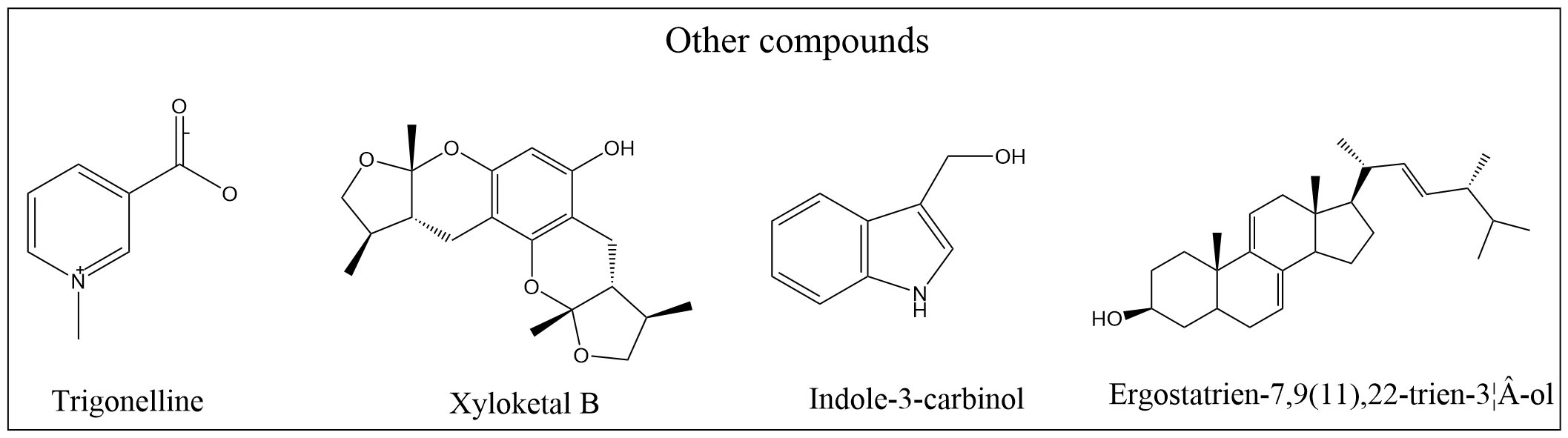
Chemical structures of some representative neuroprotective compounds (Ref. [107, 108, 109, 110, 111]).
Category | Compound | Origin | Mode of study | Experimental model | Possible mechanisms | References |
Polyphenols | Puerarin | Puerariae lobatae Radix | In vivo | tMCAO | APMK-mTOR-ULK1 pathway activation | [46] |
Pinocembrin | Propolis | In vivo | I/R injury | PDGF-CC/PDGFRα pathway activation; LC3II and Beclin1 up-regulation, p62 inhibition | [49] | |
Baicalin | Scutellaria baicalensis Georgi | In vitro; in vivo | tMCAO, OGD | ONOO–MMP-9 pathway inhibition; AQP4 inhibition; p-CaMKII inhibition | [52, 53] | |
Quercetin | - | In vivo | pMCAO | caspase-3 and PARP inhibition; parvalbumin up-regulation | [54, 56] | |
Eriodictyol | Dracocephalum rupestre Hance | In vivo | pMCAO, tMCAO | TNF-α and iNOS reduction; Bax and cleaved-caspase-3 inhibition; Bcl-2 up-regulation | [58, 59] | |
Isorhamnetin | Hippophae rhamnoides | In vivo | tMCAO | caspase-3 and MPO inhibition | [60] | |
Apigenin | - | In vivo | tMCAO | HDAC inhibition; BDNF and Syn-I up-regulation; Caveolin-1/VEGF pathway activation | [61, 62] | |
Naringin | Citrus kawachiensis | In vitro; in vivo | OGD/RO, tMCAO | ONOO |
[63, 66] | |
silymarin | Silybum marianum (L.) Gaertn | In vivo | pMCAO | Bcl-2 up-regulation; Bax inhibition | [68] | |
Sappanone A | Caesalpinia sappan L. | In vivo | tMCAO | CHOP and GRP78 reduction | [69] | |
Epigallocatechin-3-gallate | Green Tea | In vivo | pMCAO, tMCAO | PI3K/Akt/eNOS pathway activation; Nrf2 pathway activation | [70, 72] | |
Schizandrin A | Schisandra chinensis | In vitro; in vivo | OGD/R, tMCAO | AMPK-mTOR pathway activitation; AMPK/Nrf2 pathway activation | [73, 74] | |
Resveratrol | - | In vivo | tMCAO | IL-17A, IL-23, IL-10, IFN-γ and IL-4 reduction; GPx, GR, GST, SOD, CAT and Na |
[76, 77] | |
Curcumin | Curcuma longa L. | In vivo | tMCAO, BCCAO | SP1/Prdx6 pathway activation; Wnt/β-catenin pathway activation; Ngn2, Pax6 and NeuroD1 up-regulation | [79, 80] | |
Paeonol | Paeonia suffruticosa Andr | In vivo | tMCAO | astrocytes proliferation reduction; microglial activation inhibition | [81] | |
Salvianolic acid B | Salvia miltiorrhiza Bge. | In vivo | tMCAO | ROS reduction; GFAP, Iba1, IL-1β, IL-6, TNF-α and cleaved-caspase 3 reduction | [83] | |
Apocynin | Picrorhiza scrophulariiflora Pennell. | In vivo | tMCAO | NADPH oxidase inhibition, ROS reduction | [85] | |
Salvianolic acid A | Salviae Miltiorrhizae Bunge | In vivo | tMCAO | ONOO |
[87] | |
Glycosides | Cornin | Verbena officinalis L. | In vitro; in vivo | OGD, | Ang1/Tie2 axis activation; | [90, 91] |
tMCAO; | Wnt/b-catenin pathways activation; PI3K-Akt-eNOS-VEGF pathway activation | |||||
Astragaloside-IV | Astragalus membranaceus (Fisch.) Bunge. | in vivo | tMCAO | death receptor pathway and mitochondrial pathway inhibition; SIRT1 up-regulation; ac-MAPT and p-MAPT reduction | [94, 95] | |
6’-O-galloylpaeoniflorin | Paeonia lactiflora Pall. | In vitro; in vivo | OGD, tMCAO | PI3K/Akt/Nrf2 pathway activation | [96] | |
Esters | Andrographolide | Andrographis paniculata (Burm. f.) Nees. | In vivo | tMCAO | Nrf2-HO-1 pathway activation | [97] |
Ginkgolide B | Ginkgo biloba L. | In vitro | OGD/R | Bcl-2 up-regulation; Bax inhibition | [98] | |
3-n-butylphthalide | Apium graveolens L. | In vivo | pMCAO | ATP degradation Inhibition | [100] | |
Quinones | Tanshinone IIA | Salvia miltiorrhiza Bge. | In vivo | pMCAO | PI3K/mTOR/HER-3 pathway activation | [102] |
Chrysophanol | Rheum palmatum L. | In vivo | tMCAO | SOD and MnSOD activation; p-eIF2α-CHOP pathway inhibition | [103, 104] | |
Terpenoids | Glycyrrhizin | Glycyrrhiza uralensis Fisch. | In vivo | tMCAO | ONOO |
[105] |
Carvacrol | Origanum dictamnus | In vivo | tMCAO | NF-kB pathway inhibition | [106] | |
Other compounds | trigolineline | Trigonella foenum-graecum L. | In vivo | tMCAO | MPO inhibition | [107] |
Xycycline B | Xylaria sp. | In vitro; in vivo | OGD, hypoxic-ischemice brain injury model | caspase-3 reduction; Bax inhibition; Bcl-2 up-regulation | [108] | |
Indole-3-carbinol | brassica sp. | In vivo | tMCAO | SOD and CAT up-regulation | [109] | |
Ergostatrien-7,9(11),22-trien-3β-ol | Antrodia camphorata | In vivo | tMCAO | PI3K/Akt pathway activation; GSK-3 inhibition | [110] | |
Achyranthes bidentate polypeptide k | Achyranthes Bidentata Bloom | In vitro; in vivo | tMCAO, OGD | NF-κB pathway inhibition; TF and PAI-1 and MMP-2/-9 inhibition | [111] |
Polyphenols exist in many medicinal and edible plants, and possess a wide range of beneficial effects against atherosclerosis, brain dysfunction, stroke and cardiovascular diseases [112]. Polyphenols are molecules chemically characterized by the presence of at least one aromatic ring with one or more hydroxyl groups attached [113]. According to its chemical structure, polyphenols can be divided into flavonoids, lignans, stilbenes, curcuminoids and so on [2].
Bioflavonoids are well-known antioxidants with neuroprotective effects. For instance, puerarin is the main active component of Puerariae Lobatae Radix and can regulate neuronal autophagy of rats subjected to cerebral ischemia-reperfusion injury by activating the APMK-mTOR-ULK1 signaling pathway [46]. Puerarin treatment was shown to significantly reduce neuronal autophagy in the infarct penumbra of middle cerebral artery occlusion (MCAO) rats, and reduced cerebral infarction volume, cerebral edema and neurological deficits [47]. Meta analysis of 35 randomized controlled trials showed that puerarin injection can improve neurological deficit and reduce blood viscosity in patients with cerebral ischemia, and its clinical effective rate is better than aspirin [48].
Pinocembrin (PCB) is a natural flavonoid found in propolis, but also found in pepper and compositae. Administration of PCB 5 minutes before t-PA can significantly reduce ischemic BBB injury and cerebral infarction volume, reduce cerebral edema, improve survival rate, and extend the time window of t-PA treatment [49]. Other studies have shown that PCB can reduce the protein expression levels of light chain 3- II (LC3II) and Beclin1 in the hippocampal CA1 region of rats subjected to brain ischemia-reperfusion injury, increasing the level of p62, alleviating neuronal injury and cognitive decline, and reducing autophagy induced by autophagy inducer rapamycin (RAPA) [50]. Given its significant efficacy in cerebral ischemia, pinocembrin has been approved by China Food and Drug Administration (CFDA) as a new treatment drug for ischemic stroke and is currently in progress of phase II clinical trials [51].
Baicalin is a natural flavonoid found in the dried root of Scutellaria
baicalensis Georgi. Treatment with baicalin can reduce the levels of
peroxynitrite anion (ONOO-) in ischemic brain tissue, inhibit the activation of
MMP-9 and reduce the expression of AQP4 in the brain. Baicalin combined with t-PA
can expand the therapeutic window of t-PA, reduce mortality, alleviate BBB injury
and brain edema. Moreover, this flavonoid can be used as a potential candidate
for t-PA combination therapy [52]. Baicalin also decreased intracellular calcium
concentration and phosphorylation of Ca
Quercetin is a flavonol widely distributed in flowers, leaves, and fruits of different species of plants. It is an active ingredient in traditional Chinese medicines such as Bupleurum chinensis DC., Ginkgo biloba Linn. and Folium Mori. Quercetin treatment can down-regulate the expression of caspase-3 and poly ADP-ribose polymerase (PARP), reduce the cerebral infarction volume of focal cerebral ischemia animal models, decrease neuronal apoptosis, inhibit the activity of myeloperoxidases (MPO) in ischemic brain tissue and reduce the nerve injury caused by oxidative stress [54, 55]. In addition, quercetin is neuroprotective due to its modulation of paralbumin expression during ischemic brain injury [56]. 4-methylcatechol is a human intestinal metabolite of quercetin, which is an effective platelet inhibitor. It has better curative effect than the commonly used antiplatelet drug acetylsalicylic acid, which supports its application in the prevention of thrombotic stroke [57].
Eriodictyol is a flavonoid isolated from Dracocephalum rupestre Hance.
In a rat permanent middle cerebral artery occlusion (pMCAO) model, elycohol was
shown to inhibit the expression of MPO, decrease the inflammatory response, and
down-regulate the expression of TNF-
Isorhamnetin is a flavonoid isolated from ginkgo, seabuckthorn and other medicinal plants. In an experimental rat model of MCAO and reperfusion, isorhamnetin was able to reduce the activities of caspase-3 and MPO, alleviate oxidative stress, improve the recovery of neuronal function, reduce brain edema, and up-regulate the expression of tight junction proteins ZO-1 and claudin-5 to protect the integrity of BBB [60].
Apigenin is a natural flavonoid that mainly exists in celery, parsley, chamomile and other plants. Apigenin can inhibit the expression of histone deacetylase (HDAC), induce the expression of brain-derived neurotrophic factor (BDNF) and synapsin I (Syn-I) mRNA as well as reduce the cognitive impairment after cerebral ischemia-reperfusion injury in rats [61]. Apigenin can improve the neurological function of cerebral ischemia-reperfusion rats by up-regulating the Caveolin-1/VEGF pathway, and reduce pathological changes and cerebral infarction volume, and promote the proliferation of vascular endothelial cells, thereby having a protective effect on cerebral ischemia-reperfusion injury [62].
Naringin is derived from the immature or near-ripe dry peels of rutaceae plants
pomelo and grapefruit. Naringin has a strong ONOO
Silymarin is a natural flavonoid extracted from the dried fruit of Silybum marianum (L.) Gaertn, and its main components are silybin, isosilybin, silydianin and silychristin, among which silybin has the highest concentration and the strongest activity [67]. In a pMCAO rat model, silymarin was shown to inhibit apoptosis by modulating procaspase-3 level and balancing the expression of B-cell lymphoma-2 (Bcl-2) and Bax, as well as through the inhibition of autophagy by reducing the levels of LC3-II and Beclin1, thus exerting a neuroprotective effect [68].
Sappanone A is a natural high isoflavone in the legume plant Caesalpinia sappan L., which has significant anti-inflammatory and antioxidant effects. Sappanone A pretreatment can down-regulate the levels of CCAAT/enhancer binding protein homologous protein (CHOP) and glucose regulatory protein 78 (GRP78) in tMCAO rats, inhibit endoplasmic reticulum stress, reduce inflammation, oxidative stress and apoptosis, and alleviate neuronal injury after cerebral ischemia-reperfusion injury [69].
Epigallocatechin-3-gallate (EGCG) is the main component of polyphenols contained in green tea. EGCG can activate PI3K/Akt/eNOS signaling pathway, down-regulate the expression of caspase-3 and Bax, increase the expression of Bcl-2, reduce neuronal apoptosis and protect against brain injury induced by pMCAO [70]. In clinical trials, EGCG supplementation during rt-PA therapy can prolong the time window of rt-PA treatment, significantly reduce the adverse reaction of delayed administration of rt-PA and reduce the infarct volume and destruction of BBB. The underlying mechanism of EGCG in stroke involves the downregulation of MMP-2 and MMP-9 [71]. Other studies have shown that EGCG can promote angiogenesis, increase vascular density and reduce oxidative stress in tMCAO mice, which may be related to the up regulation of Nrf2 signaling pathway [72].
Lignans are plant secondary metabolites derived from the oxidative coupling of
phenylpropanoids [73]. Some lignans have been suggested to have potential in the
prevention of cardiovascular disease and, possibly, stroke [74]. Schizandrin A is
a lignin compound isolated from Schisandra chinensis, which can inhibit
autophagic death of neurons after cerebral ischemia-reperfusion injury by
regulating the AMPK-mTOR pathway, increasing the survival rate of PC12 cells
after OGD/R, and increase mechanistic target of rapamycin (mTOR) phosphorylation
[75]. Tail vein injection of Schizandrin A can improve the neurological function
of MCAO rats, reduce infarct volume, increase SOD and CAT activities, reduce ROS
production, down-regulate the transcription of COX-2 and iNOS, reduce the
inflammatory cytokines IL-1
Stilbenes are natural phenolic defence compounds occurring in a number of
different plant species that possess a wide range of beneficial activities,
including the capability to protect against oxidative stress [77]. Resveratrol is
a polyphenolic compound found in Cassia tora Linn., Veratrum
nigrum Linn., Reynoutria japonica Houtt. and other medicinal plants.
Studies have shown that the protective effect of resveratrol in IS is related to
the regulation of the brain-gut axis [78]. Resveratrol can regulate the balance
of T-helper 17/regulatory T cells (Th17/Tregs) and T-helper 1/T-helper 2(Th1/Th2)
in intestinal lamina propria and the expression of proinflammatory cytokines
(IL-17A, IL-23, IL-10, IFN-
Curcumin is the main active component of Curcuma longa L., curcumin
treatment can activate specific protein 1 (SP1), up-regulate the expression of
peroxiredoxin 6 (RDX6) after 24 hours of cerebral ischemia and reperfusion
injury, increase the number of PRDX6-positive neurons, reduce oxidative stress
and exert a neuroprotective role [82]. Curcumin can alleviate the cognitive
impairment caused by bilateral common carotid arteries occlusion (BCCAO), promote
the proliferation of neural stem cells, promote the differentiation maturation of
newly generated neural cells into neurons, increase the expression of
neurogenesis related proteins (including Ngn2, Pax6 and NeuroD 1) and up regulate
Wnt/
Paeonol is an active ingredient present in the root bark of Paeonia suffruticosa Andr. (Ranunculaceae) and the root or whole herb of Cynanchum paniculatum (Bunge) Kitagawa (Asclepiadaceae). Studies have shown that paeonol treatment can significantly reduce behavioral disorder, cerebral infarction volume and brain edema after 72 hours of ischemia-reperfusion injury, as well as alleviate the neuronal damage in ischemic core and boundary areas after 28 days of reperfusion [84].
Salvianolic acid B (Salb) is a water-soluble component extracted from Salvia miltiorrhiza Bge. Salb is the most abundant and bioactive compound in salvianolic acid, accounting for approximately 70% of its content [85]. In a tMCAO model, SalB was shown to improve cerebral blood flow, reduce platelet aggregation, inhibit EAA neurotoxicity, reduce free radicals and improve energy metabolism. These effects were linked with inhibition of astrocytes and microglia, to prevent brain injury and promote the recovery of the motor function after cerebral ischemia/reperfusion injury in rats [86].
Apocynin is an o-methoxyphenol compound isolated from the rhizomes of Picrorhiza scrophulariiflora Pennell. Apocynin can reduce NADPH oxidase activity and ROS production after cerebral ischemia in rats, thereby preventing the activation of glial cells, down-regulating inflammation and pro-apoptotic markers, and alleviating mitochondrial injury after cerebral ischemia injury in rats [87, 88].
Salvianolic acid A (SAA) is a water-soluble phenolic acid isolated from the roots of Salvia miltiorrhiza Bge. SAA can significantly reduce the cerebral infarct area and brain water content of tMCAO rats, reduce neurological deficits and pathological changes, and enhance the anti-inflammatory and antioxidant capacities of tMCAO rats [89]. SAA was shown to improve the sensorimotor and persistent memory impairments caused by focal ischemia in the unilateral frontal cortex of rats, suggesting that SAA has therapeutic potential in the treatment of IS-related memory impairment [90]. In addition, SAA can inhibit the production of ONOO-, up-regulate the phosphorylation levels of protein kinase B (AKT), forkhead transcription factor (FKHR) and extracellular signal-regulated kinase (ERK), increase glucose metabolism after cerebral ischemia, reduce neurological deficits, and protect the brain from ischemia/reperfusion injury [91].
Cornin is an iridoid glycoside isolated from the fruits of Verbena
officinalis L., which can induce angiogenesis in vitro by increasing
the proliferation, invasion and lumen formation [92]. Subsequent studies
confirmed that cornin can reduce BBB leakage and promote angiogenesis and
functional recovery after stroke by increasing the proliferation of rat arterial
smooth muscle cells (RASMC) and the expression of Wnt5a,
Astragaloside IV(AS-IV), the main active component of Astragalus membranaceus (Fisch.) Bunge., was shown to exert neuroprotective effects in a rat cerebral ischemia-reperfusion injury model. It can modulate Fas, Fas ligand (FasL), caspase-8 and Bax/Bcl-2, reduce the protein levels of apoptotic cytokines Bid, cleaved caspase-3 and Cytochrome C (Cyto C), and reduce the volume of cerebral infarction and neuronal apoptosis [94]. In addition, AS-IV can up-regulate the expression of SIRT1 in tMCAO rats, reduce the levels of acetylated MAPT (ac-MAPT) and phosphorylated MAPT (p-MAPT), and improve neurological deficits after ischemia-reperfusion, indicating that AS-IV may play a neuroprotective effect by regulating the SIRT1/MAPT pathway [95].
Galloylpaeoniflorin (GPF) is an effective ingredient extracted from Paeonia lactiflora Pall. or Paeonia veitchii Lynch. GPF pretreatment can increase the levels of phosphorylated Akt and nuclear factor-erythroid 2-related factor 2 (Nrf2) in an OGD cell model, as well as reduce the cerebral infarction volume and improve neurological deficits of rats subjected to cerebral ischemia-reperfusion injury [96].
Andrographolide is a diterpenoid lactone and it is the main active component of Andrographis paniculata (Burm. f.) Nees. By regulating the Nrf2-HO-1 signaling pathway, andrographolide can inhibit the formation of free radicals and destruction of the BBB in MCAO rats [97].
Ginkgolide B (GB) is a diterpene lactone compound isolated from Ginkgo biloba L. leaves. Pretreatment with GB was shown to down-regulate the expression of Bax, up-regulate the expression of Bcl-2, alleviate the damage of neurons and endothelial cells induced by oxygen-glucose deprivation/reoxygenation (OGD/R), and reduce the apoptosis rate of cells [98].
3-n-butylphthalide (NBP), also known as apigenin, was originally isolated from the seeds of Apium graveolens L., and was shown to protect against acute ischemic stroke [99]. NBP can inhibit the degradation of ATP and reduce the production of ATP-degradation metabolites adenosine, inosine, hypoxanthine and xanthine. Hypoxanthine and xanthine are the substrates that produce oxygen free radicals, suggesting that NBP can reduce the generation of superoxide, reduce lipid peroxidation and neuronal apoptosis [100]. NBP can down-regulate the expression of intercellular adhesion molecule-1 (ICAM-1) and protease activated receptor-1 (PAR-1) in cerebral vascular endothelial cells and improve cerebral blood flow after cerebral ischemia-reperfusion in a rat model of tMCAO and photothrombus-induced permanent cerebral ischemia [101].
Tanshinone IIA is extracted from the dried rhizome of Salvia
miltiorrhiza Bge., and is commonly used in the treatment of cerebrovascular
diseases. Tanshinone IIA can promote the recovery of cerebral
ischemia-reperfusion injury, increase glucose uptake and energy supply of neurons
by targeting GLUT1, and reducing the expression of inflammatory factors COX-2,
IL-1
Chrysophanol (CHR) is an active component extracted of Rheum palmatum
L. CHR can reduce neuronal damage caused by nitric oxide, decrease the level of
3-nitrotyrosine (3-NT) and the expression of caspase-3 in ischemic brain tissue.
It can also increase the activity of manganese dependent superoxide dismutase
(MnSOD), interrupt the feedback circuits of ROS/RNS and SOD, and inhibit the
production of ROS in ischemic brains, which indicates that CHR can alleviate the
nitrification/oxidative stress injury caused by ischemia-reperfusion injury
[103]. It has been shown that CHR treatment inhibits GRP78, phosphorylated
eukaryotic initiation factor 2
Glycyrrhizin (GL), a triterpenoid isolated from the root of Glycyrrhiza uralensis Fisch., is a natural high mobilitygroupbox 1 (HMGB1) inhibitor. In a rat model of IS, GL can reduce the expression of NADPH oxidase in the brain, inhibit the production of ONOO-, reduce the release of HMGB1, alleviate the BBB damage and neuronal apoptosis, and reduce the mortality [105].
Carvacrol is a phenolic monoterpene widely found in natural aromatic plants. In
a model of focal cerebral ischemia/reperfusion injury in rats, carvacrol could
inhibit the level of inflammatory cytokines and the activity of MPO, inhibit the
expression of iNOS, cyclooxygenase-2 (COX-2) and nuclear NF-
Trigonelline is a plant alkaloid extracted from the seeds of Trigonella foenum-graecum L., a leguminous plant. Administration of 100 mg/kg trigonelline immediately after cerebral ischemia in rats can reduce cerebral infarction, improve motor and neurological deficit scores, and significantly inhibit the expression of myeloperoxidase mediated by reduced glutathione in the cerebral cortex [107].
Xyloketal B is a natural compound isolated from mangrove fungi in the South China Sea. It can protect cortical neurons from OGD-induced cell death, reduce the volume of cerebral infarction and improve the recovery of the neural function in rats with hypoxic-ischemic brain injury [108].
Indole-3-carbinol (I3C) is a natural compound with antithrombotic activity extracted from Brassica plants. I3C can increase the levels of SOD and CAT, increase cerebral blood flow, inhibit ADP-induced platelet aggregation, and reduce the volume of ischemic cerebral infarction in tMCAO rats. In addition, I3C pretreatment shortened the length of carrageenan-induced tail thrombosis in rats, suggesting its preventive antithrombotic effect [109].
Ergostatrien-7,9(11),22-trien-3
Achyranthes bidentata polypeptide K (ABPPk) is an active ingredient isolated
from Achyranthes Bidentata Bloom. It can reduce the oxidative damage of
brain endothelial cells induced by ischemia, inhibit the expression of tissue
factor (TF), plasminogen activator inhibitor-1 (PAI-1) and NF-
The application history of natural compounds in traditional medicine and their excellent safety make them attractive in the development of human disease therapeutics. Clues to the relationship between the intake of natural compounds and beneficial effects on stroke come from epidemiological studies. A prospective study involving 74961 participants showed that the intake of fruits and vegetables was negatively correlated with the risk of stroke, suggesting that phytochemicals in fruits and vegetables may reduce the incidence of ischemic stroke by weakening the key pathological processes leading to stroke [114]. In addition, fruit polyphenol supplements have been shown to improve the cognitive and functional recovery of patients with ischemic stroke [115]. Results from a meta-analysis considering 11 prospective cohort studies suggested that high dietary intake of flavonoids may moderately reduce the risk of stroke [116]. Different prospective studies have also examined the relationship between dietary flavonoid subclasses and stroke. Higher dietary flavonol intake has been associated with a reduced risk for stroke [117]. The increased intake of flavanones has been associated with diminished risk of brain ischemia in women [118]. In a cohort study, eating foods rich in quercetin reduced the risk of thrombotic stroke [119]. Pyrogallol, a human metabolite of EGCG in green tea, can inhibit platelet aggregation in human blood, indicating that green tea may play a protective role in brain by inhibiting platelet aggregation [57].
Neuroprotective natural compounds can inhibit the key pathogenic process of ischemic brain injury, and can be used as a powerful candidate for stroke treatment. A growing body of evidence from cell culture models and animal models of brain ischemia suggests the brain protective potential of a variety of natural compounds [120]. Human ischemic stroke is extremely diverse in its manifestations, causes, and anatomic localization, whereas an experimental ischemic stroke is highly reproducible, well controllable, and standardized, allowing more precise analysis of stroke pathophysiology and drug effects, which is an indispensable tool to study stroke [121]. However, there are differences in results between animal models of stroke and humans. The reasons for this difference are: differences in brain function and structure between humans and rodents, difficulty in controlling the time window of clinical treatment after stroke as precisely as in animal experiments and the lack of basic risk factors and pathological processes of the disease in animal models. Long-term, large-scale and well-designed clinical trials are therefore needed.
Stroke is a common clinical disease of difficult treatment that is characterized by its high morbidity, disability, and mortality. The pathogenesis of stroke is complex, and it involves the regulation of a variety of genes and signaling pathways, which ultimately lead to brain damage and dysfunction. Existing drug therapy or surgical methods have some issues, such as a narrow treatment window, high risk of complications and high failure rate.
Natural products are an important source of drug discovery, being often multi-targeted, regulating multiple pathways, and displaying potential advantages in the treatment of stroke. Natural products can play a neuroprotective role by intervening in excitotoxicity, oxidative and nitrifying stress, inflammation and other pathological processes, thus deserving further investigation. The combination of natural products and other treatment methods can synergize to prolong the treatment time window and reduce stroke complications. Therefore, future research should explore the use of natural products in the treatment of stroke. Moreover, it is also necessary to conduct pharmacokinetics, pharmacodynamics and toxicological studies of natural products. Finally, the potential interaction of natural products with rt-PA should be examined before being used as a combination therapy.
FDA, Food and Drug Administration; t-PA, tissue plasminogen activator; IS,
ischemic; HS, hemorrhagic stroke; iNOS, inducible nitric oxide synthase; Glu,
glutamic acid; Asp, aspartic acid; RNS, reactive nitrogen species; NADPH,
nicotinamide adenine dinucleotide phosphate; BBB, blood-brain barrier;
TNF-
JiaL, TZhao, YL, HQ and MX contributed to literature search; JunL, PS, YF drafted and wrote the article; XWang, CL, TZheng, RC, XWei, YX and JW contributed to the discussion of the review. All authors contributed to editorial changes in the manuscript. All authors read and approved the final manuscript.
Not applicable.
We thank three anonymous reviewers for excellent criticism of the article.
This study was supported by the National Natural Science Foundation of China (NSFC, Nos. 81874419, 81673719 and 81303074), National Health Commission of the people’s Republic of China (No. 2017ZX09301064002) and Youth Innovation Team of Shandong University of Traditional Chinese Medicine.
The authors declare no conflict of interest.