† These authors contributed equally.
We used correlation analysis to examine whether changes in grey matter volume in patients correlated with clinical presentation. gray matter volume was markedly reduced in neovascular glaucoma patients than healthy controls in the following brain regions: left cingulum anterior/medial frontal gyrus; left middle frontal gyrus, orbital part; left inferior frontal gyrus, orbital part; superior temporal gyrus/right frontal inferior orbital part. VBM directly suggests that neovascular glaucoma patients have changed in the volume of multiple brain regions. These changes exist in brain areas related to the visual pathway, as well as other brain areas which are not related to vision. The alteration of specific brain areas are closely related to clinical symptoms such as increased intraocular pressure and optic nerve atrophy in neovascular glaucoma patients. In conclusion, neovascular glaucoma may cause paralgesia, anxiety, and depression in patients.
Glaucoma is a leading cause of blindness globally [1], with neovascular glaucoma
(NVG) being one of the most complicated forms of this illness. The primary
pathological manifestations of patients with NVG include hyperplasia of
intraocular fibrovascular tissue, obstruction of the trabecular meshwork, and
peripheral anterior iris adhesion, which could lead to the obstruction of aqueous
humor outflow and an increase in intraocular pressure [2] (Fig. 1). NVG can cause
deficits in visual function as well as severe pain, both of which will affect
quality of life [3]. Clinically, NVG is divided into three stages: Rubeosis,
invasion of anterior chamber angle without closure and invasion of anterior
chamber angle with closure. NVG is often secondary to diabetic retinopathy (DR),
central retinal vein occlusion (CRVO), intravascular inflammation, and tumors [4, 2]. The production of vascular endothelial growth factor (VEGF) secondary to
retinal ischemia is the most common causes of NVG [5, 6]. VEGF [7, 8], Ephrin
type-A receptor 2 (EphA2) [9], platelet derived growth factor (PDGF) [10],
hypoxia-inducible factor-
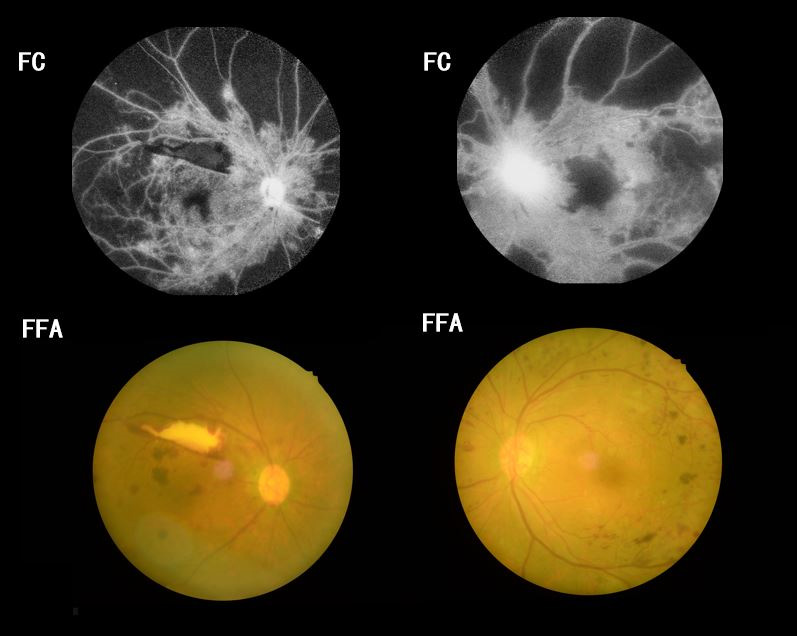
Examples FC and FFA of NVG in a patient with ischemic central retinal vein occlusion. FC, fundus camera; FFA, fluorescence fundus angiography.
Due to the complex etiology and pathogenesis of NVG, there are no precise indicators or guidelines which apply to the development and outcomes of this disease. Therefore, clinical treatment is complicated, and etiological therapies mainly included the elimination of retinal ischemia by photocoagular, cryotherapy and anti-VEGF therapy. Principles of clinical treatment include treatment of the primary diseases and timely elimination of retinal ischemia to control clinical symptoms. The core strategy of therapy involves reduction of intraocular pressure and retinal ischemic maculopathy, and eliminating neovascularization.
Magnetic resonance imaging (MRI) is well established in clinical work as a highly sensitive, non-invasive method of imaging soft tissues in the human body and observing their morphology and pathological changes. MRI offers advantages over X-ray or other imaging methods [14], and functional MRI (fMRI) allows voxel-based morphometry (VBM) for the analysis of brain morphology in fine spatial detail. VBM provides measurement of any changes in local gray matter volume (GMV), white matter volume (WMV) and the volume of the whole brain. Altered morphology in brain tissue may reflect the mechanism, development trend and clinical manifestations of disease, providing important information for practical clinical work. At present, in addition to cognitive diseases, VBM has been applied in ophthalmic diseases, including optic neuromyelitis [15], advanced monocular blindness [16], amblyopia [17], comitant strabismus [18], glaucoma [19], and acute eye pain [20] (Table 1, Ref. [15, 16, 18, 20, 21, 22, 23]).
Author | Year | Disease | Brain region with altered GMV | Brain regions with altered WHV |
Huang et al. [15] | 2016 | Optic neuritis | PoCG.L, IFG.L, ACG.L, MFG.R, IPL.R | MFG.R, SFG.R, PreCG.L, IPL.R, FFG.L, IPL.L |
Shi et al. [16] | 2019 | Advanced monocular blindness | INS.L, INS.R, ACG.R | / |
OuYang et al. [18] | 2017 | Comitant strabismus | TPOmid.L, LC, PCG.R, CUN.L, PMC.R. | TPOmid.L, TPOmid.R, PCUN.L, PMC.R. |
Lan et al. [20] | 2019 | Acute eye pain | CPL.L, Limbic Lobe-L,INS.R, INS.L, THA.L, CAU.L, CUN.R | Whole brain |
Li et al. [21] | 2012 | primary open-angle glaucoma, POAG | BA17, BA18, BA5, BA6, BA9, BA20, BA39,BA7,BA21,BA22 | / |
Chan et al. [22] | 2004 | amblyopia | OEF, PEF, FEF, SEF, PFC | / |
Li et al. [23] | 2020 | Retinal detachment | ACG.R, STG.R, ACG.R, CUN.R, HIP.L, TPOmid.L | / |
GMV, gray matter volume; WHV, white matter volume; TPOmid.L, left temporal pole: middle temporal gyrus; LC, left cerebellum; PCG.R, right posterior cingulate gyrus; CUN.L, left cuneus; PMC.R, right promotor cortex; PCUN.L, left precuneus; PoCG.L, left postcentral gyrus; IFG.L, left inferior frontal gyrus; ACG.L, left anterior cingulate gyrus; MFG.R, right middle frontal gyrus; IPL.R, right inferior parietal lobule; SFG.R, right superior frontal gyrus; PreCG.L, left precentral gyrus; FFG.L, left fusiform gyrus; INS.R, right insular lobe; INS.L, left insular lobe; STG.R, right superior temporal gyrus; HIP.L, left hippocampus; CPL.L, left cerebellum posterior lobe; INS.R, right insula; INS.L, left insula; THA.L, left thalamus; ACU.L, left caudate; CUN.R, right cuneus; OEF, occipital eye field; PEF, parietal eye field; SEF, supplementary eye field; PFC, prefrontal cortex; BA, brodmann area. |
Early studies have shown extensive and complex changes to cortical structure in glaucoma patients, and that these were significantly correlated with progression of the disease [24]. However, there is no grading system or other method for assessing changes in brain morphology in patients with NVG. VBM analysis was applied in the present research to explore whether there is a significant discrepancy of cortical volume between NVG patients and matched healthy controls (HCs), and to look for associations between those changes and the clinical manifestations and pathogenesis of NVG.
Nineteen patients (9 males, 10 females) with bilateral NVG (secondary to
ischemic central retinal vein occlusion) who had been diagnosed by the
Ophthalmology Department of the First Affiliated Hospital of Nanchang University
(Nanchang, China) were recruited to this research. Inclusion criteria for
patients with NVG included: (1) slit lamp microscopy or gonioscopy examination
shows neovascularization in the surface of iris and (or) anterior chamber angle;
(2) intraocular pressure (IOP)
The exclusion criteria for this group included: (1) the presence of acute keratitis, acute conjunctivitis or any other ocular inflammation (2) retinal ischemia caused by additional angiopathy (such as carotid stenosis); (3) a history of drugs addiction or alcoholism; (4) any condition contraindicating an MRI scan; (5) mental disorder, including but not limited to depression and bipolar disorder.
Nineteen individuals matched for age, gender and educational level met the above exclusion criteria and had no abnormal brain structure, function or metabolism evident on MRI examination.
The Medical Ethics Committee of the First Affiliated Hospital of Nanchang University (Nanchang, China) gave their approval to the study. The study design was conducted in accordance with the Declaration of Helsinki. Prior to participant signed an informed consent declaration.
All subjects underwent scanning on a 3-T MRI scanner (Siemens, Erlangen,
Germany) with an 8-channel head coil. The fast gradient echo sequence using
magnetization prepared rapid acquisition with gradient echo (MP-RAGE) was
acquired to ensure high-resolution T1-weighted images covering the entire brain.
The following were the specific parameters: repetition time (TR)/echo time (TE):
1900/2.26 ms; gap, 0.5 mm; acquisition matrix: 256
To optimize data quality, MRIcro software (Toshiba Inc., Innovista. www.MRIcro.com) was utilized to filter out irrelevant information. Matlab 7.9.0 (R2009b; The Mathsworks Inc., Natick, MA, USA) was used to run the voxel-based morphometry toolbox (VBM8, Wellcome Trust Centre for Neuroimaging, London, UK. http://dbm.neuro.uni-jena.de/vbm8/) within statistical parametric mapping (SPM version 8, Wellcome Trust Centre for Neuroimaging, London, UK. http://www.fil.ion.ucl.ac.uk/spm). VBM8 was set to partition the entire brain into cerebral gray matter, white matter, and cerebrospinal fluid as the default estimation option. Due to individual variability in brain structure, this study used the Diffeomorphic Anatomical Registration through Exponentiated Lie Algebra (DARTEL) method (implemented in VBM8) was being used to normalize images to the Montreal Institute of Neurology (MNI) standardized space, and to create universal templates for gray matter and white matter. The templates were used to standardize the gray matter and white matter of all subjects. To minimize noise in the segmented image and improve the image signal-to-noise ratio and quality, a 6 mm full width half maximum Gaussian kernel was used to smooth the modulation volume. Finally, for analysis, smoothed images were segregated into subject groups.
In the SPM8 toolbox (http://www.fil.ion.ucl.ac.uk/spm), a general linear model
(GLM) was employed. GMV was compared between groups using an independent sample t
test (
Brain regions with significant GMV value were identified as regions of interest (ROI) using REST software. The mean GMV of all voxels in each region was considered the GMV value of the ROI. Correlation analysis was used to look for any relationship between abnormal GMV values in the NVG group and their clinical features.
The hospital anxiety and depression scale (HADS), which patients completed and graded, was used to assess the level of anxiety and depression in NVG patients [25]. In the NVG group, the Visual Analogue Score (VAS) was used to assess pain perception. GraphPad prism 7 (GraphPad Software Inc., San Diego, CA, USA) was the tool we used to diagnose the relationship between HADS, VAS and GMV values, respectively.
Basic clinical data (duration of disease, IOP and best corrected visual acuity) of NVG patients and HCs were collected. SPSS 20.0 (SPSS Inc., Chicago, IL, USA) was adopted to perform independent sample t-tests comparing clinical and demographic variables between the two groups.
p values of less than 0.05 were deemed significant in all statistical analyses.
Between the NVG and HC groups, there was no significant difference in age
(p = 0.953) or handedness (p
NVG | HC | t-value | p-value | |
Male/female | 9/10 | 9/10 | N/A | |
Age (years) | 55.12 |
54.95 |
0.423 | 0.953 |
Handedness | 19R | 19 R | N/A | |
Duration (years) | 4.16 |
N/A | N/A | N/A |
Best-corrected VA-L | 0.25 |
1.05 |
5.764 | 0.021 |
Best-corrected VA-R | 0.25 |
0.95 |
5.131 | 0.026 |
OP-L | 28.12 |
14.67 |
12.842 | 0.007 |
IOP-R | 27.12 |
15.16 |
11.529 | 0.011 |
Independent t-tests (p |
In the accompanying brain regions, the VBM value in the NVG group was vastly
smaller than HCs: left cingulum anterior/medial frontal gyrus (t = 6.2185,
p
GMV | NVG group and HCs | MNI coordinates | |||
Brain areas | Peak t values | X | Y | Z | |
NVG |
|||||
1. | Temporal pole sup R/Frontal inf orb R | 7.3406 | 34.5 | 13.5 | –25.5 |
2. | Frontal inf orb L | 7.0916 | –28.5 | 7.5 | –22.5 |
3. | Frontal mid orb L | 5.6938 | –40.5 | 43.5 | –9 |
4. | Cingulum ant L/Medial frontal gyrus | 6.2185 | 0 | 37.5 | 19.5 |
GMV, gray matter volume; NVG, neovascular glaucoma; HCs, health controls; MNI, montreal nroradiology neurological institute. |
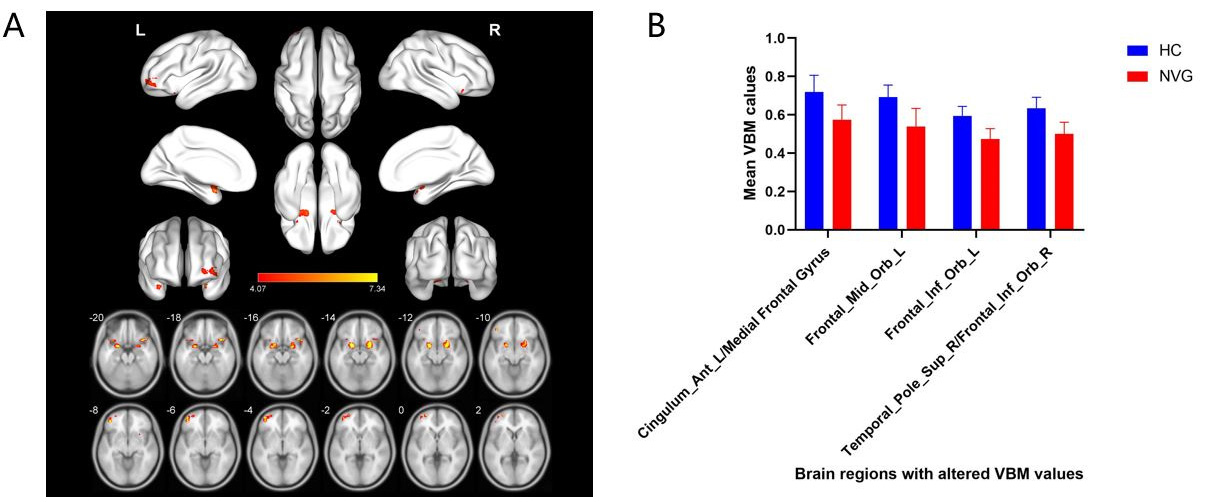
Spontaneous brain activity in NVG patients. The two groups of brain regions show statistically significant differences in terms of VBM. (A) Patients presented alternated VBM valued in Cingulum Ant L/Medial Frontal Gyrus, Frontal Mid Orb L, Frontal Inf Orb L, Temporal Pole Sup R/Frontal Inf Orb R compared with that reported in the HC group, the red areas indicate the variable VBM values. (B) The mean GMV values of two groups. VBM, voxel-based morphometry; NVG, neovascular glaucoma; Cingulum Ant L, left anterior cingulum; Frontal Mid Orb L, left middle frontal gyrus, orbital part; Frontal Inf Orb L, left inferior frontal gyrus, orbital part; Temporal Pole Sup R, right temporal: superior temporal gyrus; Frontal Inf Orb R, right frontal inferior orbital part; HC, healthy controls.
In NGV patients, GMV in the left middle frontal gyrus, orbital part was
negatively correlated with HADS (r = –0.936, p
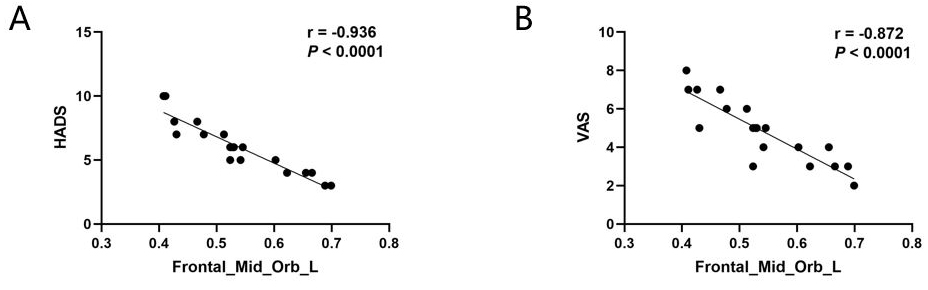
Correlation analysis between the score of HADS and the GMV
values of the Frontal Mid Orb L. (A) GMV values showed a negative correlation
with the scores of HADS (r = –0.936, p
The areas under the ROC curves were 0.871 for left Cingulum anterior/Medial Frontal Gyrus, 0.885 for left middle frontal gyrus, orbital part, 0.941 for left inferior frontal gyrus, orbital part, and 0.926 for right superior temporal gyrus/right frontal inferior orbital part (Fig. 4).
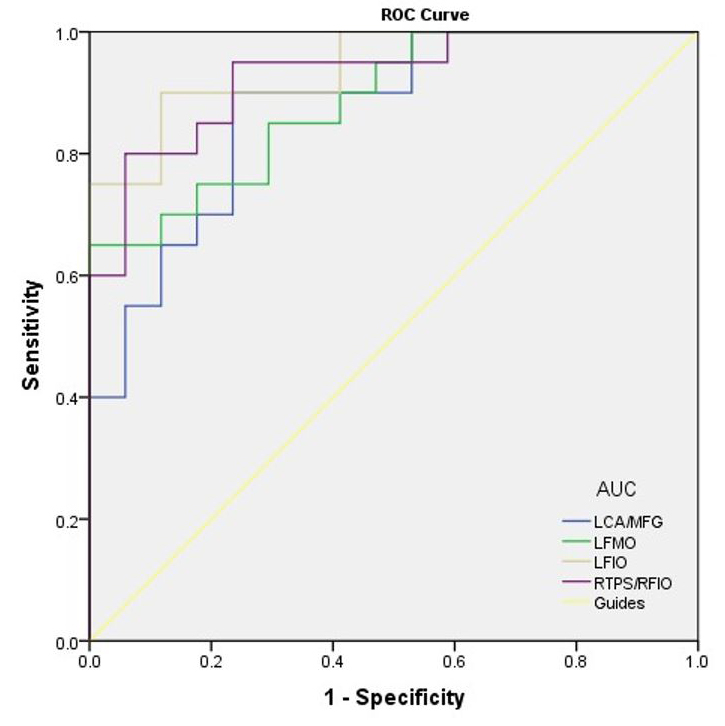
ROC curve of the VBM values in altered brain areas. The area
under the ROC curve were 0.871 (p
This is the first study of using VBM to assess gray matter volume abnormalities in NVG patients. VBM has, however, been widely used in research on both neurological and ophthalmological diseases, and has shown clear advantages in the exploration of the pathological mechanism of these diseases.
Studies have established that glaucoma patients generally show atrophy of specific brain areas or changes in functional connectivity between brain areas. Aditya et al. [19] showed varying degrees of volume reduction along the visual pathway in glaucoma. Laura et al. [26] showed reduced cortical thickness and volume in both primary visual cortex and association visual areas in glaucoma patients. Fukuda et al. [27] found decreased gray matter density (GMD) in primary open-angle glaucoma (POAG) patients, particularly in the visual cortical areas (Brodmann areas 17, 18, 19). In patients with POAG, Jiang et al. [28] found altered volume in brain regions which are highly correlated with the retinal nerve fiber layer. Similarly, Ziko et al. [29] found that VBM showed significantly decreased volume in the visual pathway and other brain areas which are related with the visual system. Li et al. [21] suggested that POAG patients with advanced disease show degenerative changes in visual-related cerebral cortex, dorsal and ventral visual pathways.
In this study, the NVG group experienced statistically significant GMV reductions when compared to the HCs in left cingulum anterior/medial frontal gyrus; left middle frontal gyrus, orbital part; and the right temporal: superior temporal gyrus/right frontal inferior orbital part.
The cingulate gyrus occupies a crucial position in the limbic system [30] and exerts an important role in emotional control and pain management. According to current studies, the anterior cingulate gyrus is involved in not only the regulation of heart rate or blood pressure, and other autonomic nerve activities [31]. But it’s also implicated in a variety of sophisticated body and visceral motor processes, as well as pain responses, attention maintenance [32], decision-making processes [33], and pain management [34]. Previous studies have demonstrated that the anterior cingulate gyrus cortex of the right dorsal horn may be related to visual cognition and emotion [35]. Results of the present study indicate decreased gray matter volume of anterior cingulate gyrus in NVG patients. This result suggesting that NVG patients may have dysfunction in visual cognitive function [22].
The frontal gyrus is an important component of the dorsal visual pathway [36]. It is concerned with recognition and judgement based on spatial information about objects and simultaneously plays an important role in optic nerve positioning, multi-retina correspondence, and the formation of scotoma of the fixation point [37]. The frontal gyrus includes superior, medial and inferior frontal gyrus, and abnormalities of its structure or function have been investigated in many ophthalmic diseases, including but not limited to diabetic retinopathy [38], strabismus with amblyopia [39], corneal ulcer [40] and ophthalmectomy [14]. In this study, we found that VBM results indicated significant GMV reduction in all of these regions (medial, superior, and inferior frontal gyrus), which are the same as Wang et al. [41] and it may be related to increased intraocular pressure (IOP).
The medial frontal gyrus is the medial side of the superior frontal gyrus, located anterior to the parasitical central lobule. Research suggests that the medial frontal gyrus is linked to the decision-making process and the subsequent execution process [42]. In addition, the frontal eye field (FEF) area in the center of the medial frontal gyrus regulates spontaneous eye movements and is also linked to emotional transfer [43]. The cortex of the medial frontal gyrus represents one of the components of the default model network which includes the inferior parietal cortex, medial and superior frontal gyrus and precuneus [44, 45]. Furthermore, it plays a fundamental role in specific emotion such as depression and anxiety [46]. The GMV of the medial frontal gyrus was diminished in this study, illustrating that patients with NVG may have visual pathways that are compromised. Jiang et al. [28] found that the frontal gyrus area of patients with primary open-angle glaucoma (POAG) shows increased volume and blood oxygenation level dependent (BOLD ) signals. They concluded that this may be due to edema of local cerebral tissue and microsomal activation, that increased blood flow may be a pathological compensatory response after structural and functional impairment, and that these results may indicate nerve damage in the frontal gyrus. This hypothesis may explain why Williams et al. [47] found increased medial frontal gyrus volume in glaucoma patients in early stage, which is contrary to this study.
The decrease in GMV volume in the superior and inferior frontal gyrus in the present study agrees with the results of other existing studies [21, 48], supporting the finding of damaged cortical visual pathways in NVG patients. These results collectively suggest that NVG patients may have abnormalities in eye movement, cognitive function and visual processing ability.
The superior temporal gyrus is involved in visual signal processing, auditory processing, auditory memory and other physiological processes. The superior and middle temporal gyri are thought to specialize in processing dorsal signals along the visual pathway [49]. Previous studies have observed abnormalities in the superior temporal gyrus in specific ophthalmopathy, including but not limited to optic neuritis [50], diabetic retinopathy [51], comitant strabismus [18], and retinal detachment [23]. In this study, the decrease in GMV in the superior temporal gyrus may indicate atrophy of temporal retinal fibers in NVG patients [29] (Table 4).
Brain region | Brain function | Anticipated results |
Cingulum anterior gyrus | Cognition; emotion; memory and other cognitive functions | Defects of cognition and emotion; abnormal pain management |
Medial Frontal Gyrus | Involving in default mode network (DMN); | Dysfunction of DMN; anxiety and depression; pain-related diseases |
Middle frontal gyrus | Consist of Frontal eye field; language processing; part of DMN | Depression and anxiety; impairment of eye motion and visual acuity; increasing pain sensitivity |
Inferior frontal gyrus | Emotional and cognitive empathy; part of DMN | Impairment of ocular movement and cognitive; depression and anxiety |
Superior temporal gyrus | Relating to visual information processing and visual search insight, affecting auditory processing and auditory memory | Abnormal visual information processing and visual impairment |
Notably, some of the studies also mentioned that beta-amyloid accumulation may be associated with altered GMV in NVG patients [52], which happens to be a typical pathological change in Alzheimer’s patients. There is still a plenty of room to be investigated.
The present study has the following limitations: (1) The participants included in this study may be insufficient. However, selection followed precise inclusion and exclusion criteria, and the differences between potentially confounding factors were not statistically significant; (2) The study samples are all derived from hospital outpatient data, which risks selection bias; (3) Due to equipment and technical limitations, the spatial resolution of imaging may not be optimal.
In summary, NVG patients show decreased GMV values in certain brain areas, the dysfunction of those brain areas may be related to the appearance of corresponding symptom in NVG patients such as the depression and anxiety.
Conceptualization, methodology, writing original draft preparation, writing reviewing and editing—HZ, YDS, RBL. Data curation, formal analysis—QMG, YCP, LJZ, HYS, QYL. Resources, methodology, supervision, projection administration, funding acquisition—YS.
All research methods were approved by the committee of the medical ethics of the First Affiliated Hospital of Nanchang University (2015016) and were in accordance with the 1964 Helsinki declaration and its later amendments or comparable ethical standards. All subjects were explained the purpose, method, potential risks and signed an informed consent form.
Not applicable.
This research was funded by National Natural Science Foundation of China, grant number 81660158, 81460092, and 81400372; Natural Science Key Project of Jiangxi Province, grant number 20161ACB21017; Health Development Planning Commission Science Foundation of Jiangxi Province, grant number 20175116.
The authors declare no conflict of interest.