†These authors contributed equally.
Medicinal plant breviscapine is shown to exhibit a protective role in tissue damage after cerebral hemorrhage. The effects of breviscapine on neurological deficit score, brain tissue water content, brain pathological tissue changes, blood-brain barrier bidirectional regulation, and inflammatory factors after cerebral hemorrhage in rats were observed. Western blot and real-time quantitative polymerase chain reaction were performed to explore how Periostin and nuclear factor kappa-B pathway-related factors protein expression contribute to the protective effects of breviscapine on brain injury. Breviscapine inhalation could reduce neurological deficit scores and brain tissue water content. Hematoxylin-eosin staining showed that breviscapine could improve the pathological changes of brain tissue and alleviate brain damage. Breviscapine reduced the abnormal increase of Evans blue content caused by a cerebral hemorrhage, and could significantly inhibit the levels of inflammatory factors interleukin-6 and tumor necrosis factor-α. Also, breviscapine significantly inhibited the expressions of Periostin and nuclear factor kappa-B pathway-related factors after cerebral hemorrhage, and alleviate brain damage by down-regulating Periostin expression and inhibiting nuclear factor kappa-β signaling pathway.
The medicinal plant breviscapine is a flavonoid active ingredient purified from the plant scorpion flower (Gao et al., 2017) and is mainly composed of scutellarin and a small amount of apigenin-7-O-β-d-glucuronide, with scutellarin accounting for more than 90%. Scutellarin has the effects of improving cerebral circulation and increasing cerebral blood flow, while apigenin-7-O-β-d-glucuronide can accelerate drug transport and increase drug absorption (Zeng and Cai, 2017). Zhang et al. (2007) discovered that breviscapine could effectively reduce the inflammatory response after brain death, thereby protecting the liver from damage, and down-regulate the level of malondialdehyde (MDA) and increase the activities of ATPase and superoxide dismutase (SOD) in mitochondria (Zhang et al., 2011). Guo et al. (2014) found that breviscapine injection can improve neurological deficits and reduce infarct volume and water content in rats with cerebral ischemia-reperfusion injury. Studies showed that breviscapine could relax blood vessels, improve microcirculation, blood supply, and flow to the heart and brain, regulate blood lipids, reduce blood viscosity, and eliminate oxygen free radicals to protect against ischemia-reperfusion injury (Bao et al., 2018; Jiang et al., 2017; Lin et al., 2016).
Periostin, which is an extracellular matrix protein involved in normal bone remodeling and biology of different cancer cell growths and metastases, is overexpressed in oral, thyroid, breast and gastric cancers (Bonnet et al., 2016; Izuhara et al., 2016; Landry et al., 2018; Lu et al., 2014). Moreover, Siriwardena et al. (2006) showed that Periostin is abnormally elevated in oral squamous cell carcinoma and promotes the metastasis of cancer. In primarily cultured malignant melanoma cells, high-expressed Periostin is mainly derived from tumor cells induced by cancer-associated fibroblasts, suggesting that high expression of Periostin is associated with malignant progression of the tumor (Tilman et al., 2007). Elevation of Periostin is related to the severity and pathogenesis of chronic inflammation and plays a crucial role in the pathogenesis of atopic dermatitis (Izuhara et al., 2017). Studies have shown that Periostin may have a toxic effect on damaged brain tissue, as it has been observed to aggravate the blood-brain barrier disruption, cause brain edema significantly, and damage nerve function (Dong et al., 2017; Liu et al., 2017). Breviscapine can reduce the inflammatory response after brain injury, but it is not clear whether it is related to Periodin.
Cerebral hemorrhage refers to brain tissue damage and dysfunction caused by the sudden rupture of blood vessels in the brain, and it has high morbidity, mortality, and recurrence rate (Joice et al., 2009; Ziai and Carhuapoma, 2018). Secondary brain injuries are local cerebral perfusion disorders caused by enlarged hematoma, the formation of edema around the hematoma, damaged blood-brain barrier, and permeability changes (Balog et al., 2016; Ito et al., 2010). After cerebral hemorrhage, under ischemia, hypoxia or other conditions, the inflammatory cytokines and their vasoactive substances produced by various cells in the brain will increase the permeability of the blood-brain barrier and aggravate edema around the hematoma, therefore continuously increased intracranial pressure will eventually exacerbate conditions and even lead to death (Corps et al., 2015; Hiebert et al., 2015; Min et al., 2017). Therefore, how to reduce the damage of the blood-brain barrier, improve the function of the blood-brain barrier, thereby reducing brain edema is also one of the keys to the treatment after cerebral hemorrhage.
At present, there are few reports conducted on the mechanism of breviscapine inhalation in cerebral hemorrhage. Therefore, by exploring the effects of breviscapine inhalation on brain injury and cerebral edema after cerebral hemorrhage in rats, the current study found that in the treatment of cerebral hemorrhage in rats, breviscapine inhalation can effectively improve brain microcirculation and protect brain cells. The present findings provide a theoretical basis and experimental basis for the clinical treatment of cerebral hemorrhage.
One hundred and twenty Sprague Dawley (SD) rats (300-400 g) were used. The rats were fasted but provided with access to water 12 h before the model preparation. All the rats were housed in a specific pathogen-free environment at room temperature 21-23 °C in relative humidity of 44%-70% under 12 h light/dark circle for 12 hours (h). Breviscapine injection (140111) was purchased from Shanghai First Biochemical Pharmaceutical Co., Ltd. The 120 SD rats were randomly divided into the Sham group, Sham + breviscapine group, Model group, and Model + breviscapine group by digital table method, with 30 rats in each group, and five additional mice in each group were used to supplement mice died during the establishment of the model.
Our primary research included IL6 levels, TNF-α levels, histological inflammatory cells, and related inflammatory pathways, and experiments such as neurological deficit scores and blood-brain barrier permeability are measured as “by-products.”
Calculated according to the per capita weight of 60 kg, breviscapine intravenous injection was administrated at 10-20 mg/d per person, and the clinical dose is used at 0.16-0.32 mg/kg per day. As the average weight of SD rats was 350 g, the administration volume was 0.5 ml/head (Chen et al., 2012), the maximum clinical dose was 1 mg/kg, and the drug was injected three times a day. Ovalbumin (SLBK1399V, Sigma, USA) was prepared with a sensitizing solution at the concentration of 2 mg/ml as a positive control, with physiological saline used as a solvent. Breviscapine injection was diluted to 0.7 mg/ml, with physiological saline used as a sensitizing solution.
The rats in Sham + breviscapine group and the Model + breviscapine group inhaled breviscapine for 15-20 min each time via the oral and nasal inhalation exposure system (IES-N, Shanghai Ruiman Information Technology Co., Ltd., CA) 12 h before modeling, and the aerosol particle size spectrometer (3330, TSI, USA) was used for analysis. The aerosol was inhaled at 1, 2, and 3 days after modeling. The reason for administration before modeling was to prevent early inflammation in rats and prevent it from interfering with later data, and the related experiments of the breviscapine after modeling are the main ones.
After the SD rats were anesthetized, the right femoral artery and right femoral vein were intubated with polyethylene catheters filled with heparin saline, and heart rate, mean arterial pressure (MAP) and bleeding were monitored. The caudate nucleus was located according to the stereotactic atlas of the rat brain (0.2 mm before the anterior condyle, 3.0 mm beside the right side of the midline, and 5.5 mm deep). Three locations in the tail vein were marked, and keratinous phosphorus within 1cm around the tail vein was scraped off to prepare skins. The tongues of the rats were pulled using hemostatic forceps, and incisors teeth were fixed on the incisor hooks, raised the mandibular angle to adjust the distance between the two ear rods, and set the interauricular line, 2.4 mm lower than the interauricular line. The anterior and posterior fontanelles were steadily kept at the same level as the head of the rat. Next, the head was fixed using a variety of tools such as hemostats to expose the rat's head fully. The head was prepared for skin and iodophor disinfection. Microsampler was placed on the brain stereotaxic instrument, and the needle tip was adjusted to mark the tail shell nucleus. The tail artery was cut off to collect 50 μl non-anticoagulated autologous arterial blood from the rats. The needle into the nucleus of the caudate nucleus was rapidly inserted to inject the non-anticoagulated autologous arterial blood collected at a constant rate and sutured the incision after the injection. The sham group was injected with 50 μl of physiological saline, and the rest of the operation was similarly operated as the model group. After the operation, the rats were placed in a prone position, and the head height was placed on a sloping foam board to prevent sputum clogging. The rats were observed for their breathing, and suction was implemented when necessary, the rats were consciously caged and fed ( Xue and Del Bigio, 2000; Yang et al., 1994).
The score was rated according to the Zea Longa neurological deficit 5-point method (Longa et al., 1989) as follows: 0 point (no neurological deficit signs); 1 point (when the tail is upside down, the left forelimb close to the chest wall or cannot stretch); 2 points (when walking, turn to the side to chase the tail, and the left forelimb long drag or contracture); 3 points (dump to one side when standing or walking); 4 points (disturbance of consciousness). The rats scored 1 to 3 points were successfully enrolled in the model and used in the next experiment, while those scored 0, 4, and dead rats were excluded. The samples were tested or sampled at 12 h, 3 d, and 7 d after surgery. Rat brain tissues were taken using rapid craniotomy method after general anesthesia with 40 mg/kg sodium pentobarbital (B5646-50mg, ApexBio, USA, intraperitoneal) and 0.1 mg/kg Jingsongling (xylidinothiazole, BD161432, Bidepharm, CA, IM).
The dry and wet specific gravity method was used to detect the water content of brain tissue. The right brain tissues before coronal incision were taken (three rats in each group), and the wet tissues were placed in an electric blast drying oven (SB-YH0235, Shanghai Yiheng, CA). After dried at 95 °C to constant weight, the dry weight was measured. BWC (%) = [(brain wet weight - dry weight) ÷ wet weight] × 100%, accurate to 0.001 g.
Three rats from each group were used for HE staining. The samples were tested or sampled at 12 h, 3 d, and 7 d after surgery. Rat brain tissues were taken by applying rapid craniotomy method after general anesthesia with 40 mg/kg sodium pentobarbital and 0.1 mg/kg Jingsongling. The brain tissues were fixed in 4% paraformaldehyde for 24 h and sectioned into about 2 mm in the coronal position and paraffin-embedded. Next, the tissues were sliced into a thickness of 5 μm, dewaxed, and conventionally stained by hematoxylin-eosin (HE). Briefly, the sections were stained by HE for 20-30 s, by hydrochloric acid ethanol for 5 s, by ammonia water for 5 s, and by eosin for the 20 s and observed under a microscope. The sections were then immersed in 70% ethanol for 5 min, soaked in 90% ethanol for 5 min, in anhydrous alcohol, in xylene I, in xylene II for 15 min, and in xylene III for 5 min. After sealing, the pathological changes of brain tissues around the hematoma were observed under a microscope (CX23, OlympusCorporation, Japan), photographed and recorded.
Four rats were randomly selected from each group at 12 h, 3 d, and 7 d, and weighed 1 h before perfusion fixation. 0.5% Evans blue (20170412, Solarbio, CA) was injected into via tail vein at 6 ml/kg body weight. After anesthetizing the rats by 40 mg/kg sodium pentobarbital, the thoracic cavity was opened, and the heparin saline (1024E027, Solarbio, CA) 200-300 ml were intracardially perfused (the perfusion can be terminated when the fluid in the right atrium became clear). The brain was decapitated and divided into left and right parts using a blade from the middle sagittal suture. After cutting, the brains were immersed in 3 mL trichloroacetic acid solution (2016113001, chronchemicals, CA), incubated at 4 °C for 24 h, centrifuged at 3000 g for 20 min, and the OD of supernatant was detected at a wavelength of 620 nm. The trichloroacetic acid solution was used as a blank control. The software (Oringen 7.0) was used for data analysis, the EB standard curve was plotted, and the EB content was calculated.
Four rats were randomly anesthetized at 12 h, 3 d, and 7 d after the operation. Blood (5 ml) was collected through abdominal aorta, and the blood samples were stored at -20 °C for testing. The serum was separated by centrifuging at 12,000 g for 5 min, and inflammatory factors, including interleukin-6 (IL-6, ml063159, Shanghai Enzyme-linked Biotechnology, CA) and tumor necrosis factor-α (TNF-α, ml022566, mlbio, CA), were measured using an ELISA kit.
The lysis of each group of cells and tissues, as well as the extraction of total RNAs, were carried out at 4 °C using the Trizol method and all the consumables and reagents used in the extraction process were subjected to Diethyl pyrocarbonate (DEPC) treatment. mRNAs were reverse-transcribed using TaqMan Reverse Transcription Reagents (N8080234, ThermoFisher, USA). RT-qPCR was performed according to the manufacturer's instructions of the opticon RT-PCR detection system (ABI 7500, Life Technology, USA). The thermal cycle was set as follows: pretreated at 95 °C 10 min, followed by 45 cycles at 95 °C for 30 seconds (sec), at 60 °C for 1 min, and finally at 60 °C for 1 min, and preserved at 4 °C. The relative expression levels were calculated using the 2-ΔΔCt where Ct is the number of cycles that the fluorescence signal in each reaction tube goes through when it reaches a set domain, and Δ represents the difference between the objective gene Ct value and the internal reference gene Ct value. Using this method, the target gene relative to the housekeeping gene can be directly obtained where β-actin and histone H2 serve as housekeeping genes for mRNA. The primers sequence are in Table 1.
Gene-ID | Forward primers | Reverse primers |
---|---|---|
TNF-α | 5’-AAGCTGTCTTCAGGCCAACA-3’ | 5’-GCAAAAGAAAAGGGGGAGGC-3’ |
Periostin | 5’-TGCAAAAAGACACACCTGCAA-3’ | 5’-CCGAAGTCAATGGGGCTCTT-3’ |
IL-6 | 5’-AGCGATGATGCACTGTCAGA-3’ | 5’-GGAACTCCAGAAGACCAGAGC-3’ |
p65 | 5’-AGGCACCAGAGGGAGGATTT-3’ | 5’-GAGAAGGAAAACTGCGAACCG-3’ |
β-actin | 5’-CTTTGCAGCTCCTTCGTTGC-3’ | 5’-CCTTCTGACCCATTCCCACC-3’ |
H2 | 5’-TGTCGGCAAAGGGGACTACT-3’ | 5’-TCGCGGCATCTCTTACGTTT-3’ |
A RIPA lysate (89901, ThermoFisher, USA) containing protease inhibitor and phosphatase inhibitor was used to extract the total proteins from SD rat tissues. The Protein Assay Kit (A53227, ThermoFisher, USA) was used to detect protein concentration. 30 μg proteins was separated on 10% SDS-polyacrylamide gels and transferred to PVDF (HVLP04700, Millipore, USA), which was blocked by 5% non-fat milk powder at 37 °C for 1 h, shaken for 2 h at room temperature, and then incubated with specific antibodies (TNF-α (42 kDa; Rabbit; 1: 1000; ab66579; Abcam, UK); IL-6 (23 kDa; Mouse; 1: 1000; ab9324; Abcam, UK); Periostin (100 kDa; Rabbit; 1: 1000; ab14041; Abcam, UK); p65 (65 kDa; Rabbit; 1: 1000; #8242; CST, USA); histone H2 (15 kDa; Rabbit; 1: 1000; #2595; CST, USA) or β-actin (42 kDa; Mouse; 1: 1000; ab8226; Abcam, UK)) overnight at 4 °C. The target bands were incubated with an HRP-conjugated secondary antibody (1: 5000; ab205718/ab205719; Abcam, UK) for 2 h at room temperature. Finally, SignalFire ECL reagent (6883, Cell Signaling Technology, USA) was used to measure the signals, and imageJ (version 5.0, Bio-Rad, USA) was used to analyze and count the gray value of the band.
Kolmogorov-Smirnov test was used to test the normality of the data, and the data conformed to the normal distribution. The data were shown as the mean ± SD. A comparison between the groups was conducted using a one-way analysis of variance, followed by the Dunnett-test in GraphPad Prism 7.0 (Graph-Pad Software Inc). Statistically significant was defined as P < 0.05.
As previously described (Xue and Del Bigio, 2000; Yang et al., 1994), the current experiment constituted a model of cerebral hemorrhage through rapidly injecting autologous arterial blood into the caudate-putamen. After successful evaluation of modeling, rats showed reduced activity and behavioral changes, such as turning to one side while walking. A total of 4 rats died in the experiment, two of which died due to intestinal edema and obstruction, while another two died 2 to 3 days after modeling. After modeling, a total of 2 rats were evaluated as 0 points and were therefore excluded from the experiment. The stable and qualified ones randomly supplemented the excluded mice from the experiment.
The effects of breviscapine inhalation on brain injury after cerebral hemorrhage in rats were evaluated by Zea Longa neurological deficit 5-point method. The results showed that there were no apparent neurological deficits in the Sham group or Sham + breviscapine group. Compared with the Sham group, the neurological deficit scores in the Model group were significantly increased at 12 h, 3 d, and 7 d after surgery, while the neurological deficit scores in the Model + breviscapine group were substantially lower than those in the Model group (P < 0.001, Fig. 1A). Also, we investigated the effects of breviscapine inhalation on brain edema after cerebral hemorrhage in rats, and the data found no significant change in brain water content in the Sham group or Sham + breviscapine group. Compared with the Sham group, the brain water content of the Model group was increased significantly at 12 h, 3 d, and 7 d after surgery, and peaked at 3 d, while the water content of brain tissue in Model + breviscapine group was significantly lower than that in Model group (P < 0.001, Fig. 1B).
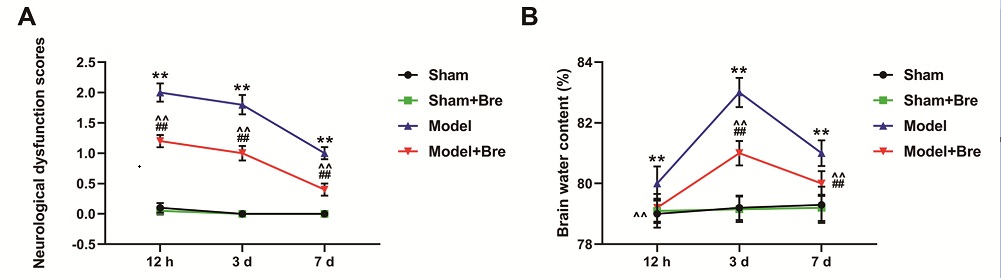
Effects of breviscapine inhalation on brain damage and brain edema after cerebral hemorrhage in rats. (A) neurological deficit scores and (B) brain tissue water content at 12 h, 3 d, and 7 d after surgery, in Sham group, Sham + breviscapine group, Model group, and Model + breviscapine group (Bre: breviscapine; **P < 0.001 vs. Sham; ##P < 0.001 vs. Sham + breviscapine; ^^P < 0.001 vs. Model).
Three days after the operation, a small number of inflammatory cells infiltrated around the needle canal in the Sham group and the Sham + breviscapine group, and the surrounding nuclei and cytoplasm were uniformly stained. However, in the Model group, the cells disorderly arranged, and were swollen and degenerated, the nuclear structures were blurred, the resolvable nuclei were significantly reduced, moreover, the gaps around the cells and blood vessels were widened, accompanied by a large amount of inflammatory cell infiltration. Compared with the Model group, the cytoplasm and tissue edema, nuclear condensation, and inflammatory cell aggregation of the brain tissues of the rats in the Sham + breviscapine group were relatively alleviated (Fig. 2).
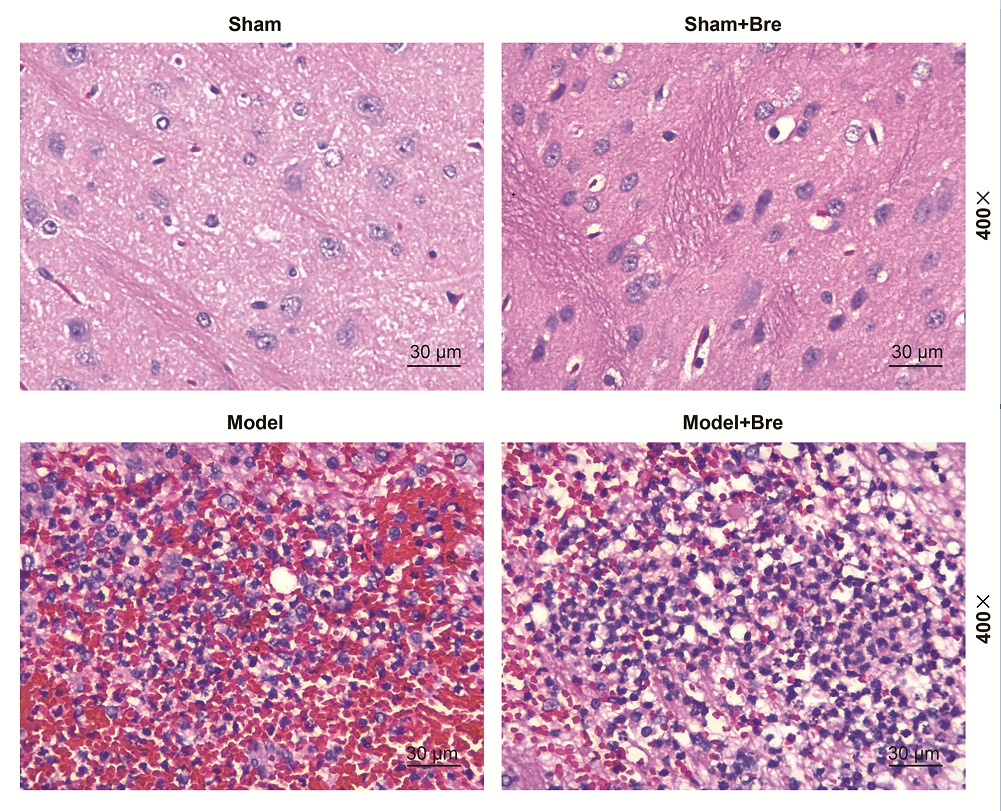
Representative HE staining and immunostained images of Sham group, Sham + breviscapine group, Model group, Model + breviscapine group in the brain tissue (Bre: breviscapine; HE: hematoxylin-eosin. Magnification x 400, Bar = 30 μm).
The results of the EB test showed no significant changes at postoperative EB levels in the Sham group or the Sham + breviscapine group. Compared with the Sham group, the EB content in the Model group was increased significantly at 12 h, 3 d, and 7 d after surgery, and peaked at 3 d, while the EB content in the Model + breviscapine group was significantly lower at each time point (P < 0.001, Fig. 3A). To investigate the effects of breviscapine inhalation on inflammatory factors after cerebral hemorrhage in rats, ELISA results showed that IL-6 and TNF-α levels were significantly elevated in the Model group, while the Model + breviscapine group significantly inhibited inflammation reaction in the Model group (P < 0.05, Fig. 3B, C). WB and qPCR were performed further to detect the levels of inflammatory factors in brain tissues, and the results were consistent with those of ELISA. Compared with the Sham group, IL-6 and TNF-α levels in Model group were increased significantly at 3 days after surgery, and the level of IL-6 and TNF-α in the Model + breviscapine group was substantially lower than that in Model group (P < 0.05, Fig. 4A).
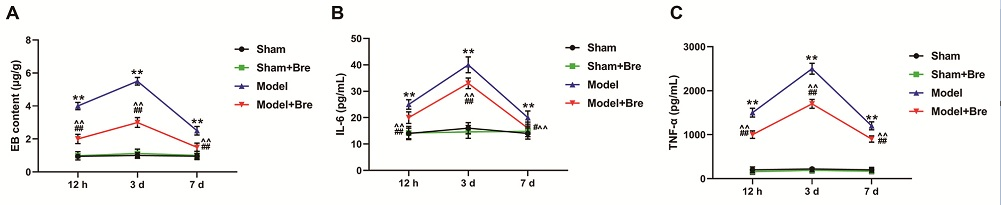
Effects of breviscapine inhalation on the blood-brain barrier and inflammatory factor levels after cerebral hemorrhage in rats. (A) Evans blue (EB) was used to detect EB levels in the Sham group, Sham + breviscapine group, Model group, and Model + breviscapine group at 12 h, 3 d, and 7 d after surgery. ELISA was used to detect (B) IL-6 level and (C) TNF-α level in the Sham group, the Sham + breviscapine group, the Model group, and the Model + breviscapine group at 12 h, 3 d, and 7 d after surgery (Bre: breviscapine; **P < 0.001 vs. Sham; #P < 0.05 vs. Sham + breviscapine; ^^P < 0.001 vs. Model).
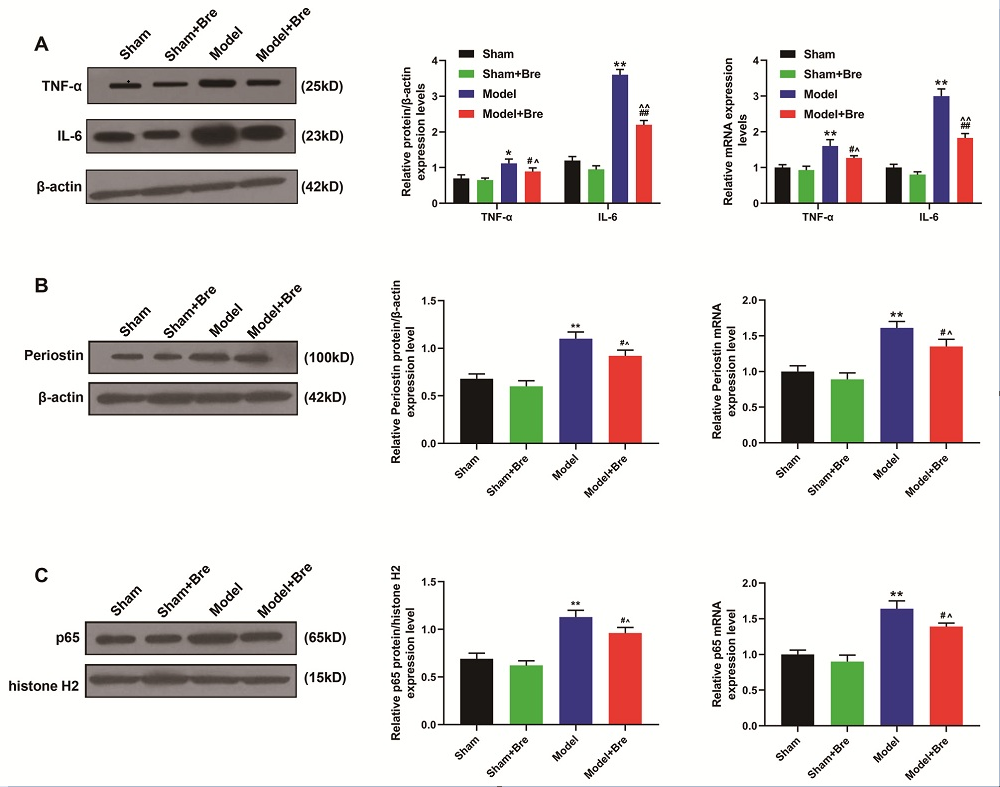
Effects of breviscapine inhalation on the levels of inflammatory factors, Periostin and NF-κB pathway after cerebral hemorrhage in rats. (A) WB and RT-qPCR were used to detect IL-6 and TNF-α levels in the Sham group, Sham + breviscapine group, Model group, and Model + breviscapine group at 12 h, 3 d, and 7 d after the surgery. WB and RT-qPCR were used to detect (B) Periostin levels and (C) p65 levels at 3 days during postoperation in the Sham group, the Sham + breviscapine group, the Model group, and the Model + breviscapine group (RT-qPCR: Real-time Quantitative PCR; Bre: breviscapine; *P < 0.05 vs. Sham; #P < 0.05 vs. Sham + breviscapine; ^P < 0. 05 vs. Model).
To investigate the protective mechanism of breviscapine inhalation after cerebral hemorrhage in rats, WB and qPCR were conducted to detect Periostin protein and nuclear factor kappa-B (NF-κB) pathway-related factors (p65). The results showed that the expressions of Periostin and p65 in the Model group were significantly higher than those in the Sham group and the Sham + breviscapine group 3 days after surgery, while the Model + breviscapine group can reverse the increase in Periostin and p65 expressions caused by the Model group (P < 0.05, Fig. 4B, C).
Cerebral hemorrhage is a common and frequent disease, accounting for about 10%-30% of cerebrovascular diseases (Balog et al., 2016; Sansing, 2016). Injury after cerebral hemorrhage is initially a result of mechanical damage caused by hematoma enlargement, but simple hematoma compression is not the main cause of severe brain injury (Ziai and Carhuapoma, 2018). Secondary brain injury after cerebral hemorrhage is considered to be an important factor leading to brain tissue damage (Min et al., 2017; Ortega-Perez and Amaya-Rey, 2018). Studies have shown that breviscapine has pharmacological effects such as inhibition of inflammation, and it also functions to regulate blood lipids and oxidation resistance (Zhang et al., 2007). Gao et al. (2017) explored the role of breviscapine in cardiovascular and cerebrovascular diseases and reported that breviscapine has positive effects on vasodilation, ischemia-reperfusion injury, and anti-inflammatory effects. For example, they found in the literature that dose-dependent breviscapine relaxes norepinephrine-induced vasoconstriction without affecting endothelial function. In the study of breviscapine on the lung, scholars found that it can inhibit the expression of IL-18 and ICAM-1 and protect the lungs from inflammation. However, the specific mechanism of breviscapine inhalation in brain injury and cerebral edema after cerebral hemorrhage in rats has not been found. Ischemic injury can cause significant changes in neurobehavioral performance in rats (Kim et al., 2017). According to the evaluation of post-modeling rat cerebral hemorrhage, breviscapine inhalation can significantly improve the neurological deficit score by blocking the inflammatory signaling pathway. Yang et al. (1994) have shown that cerebral edema occurs 1-2 hours after cerebral hemorrhage, peaks at 2d-3d, and then gradually decreases with time, which is consistent with the results of the current study. Our experiments also showed that breviscapine inhalation significantly reduced the degree of cerebral edema in rats.
The formation of edema after cerebral hemorrhage is related to many factors, and the destruction of the blood-brain barrier is one of the critical links (Zheng et al., 2016). The blood-brain barrier, which is a complex structure located between brain tissues and blood (Zheng et al., 2016), is composed of vascular endothelial cells, astrocytes, and the like (Liu et al., 2014). The extracellular matrix secreted by the cells constitutes the basement membrane to maintain the stability of the basic structure and then controls the entry and exit of water, electrolytes, and macromolecular substances via various components such as transport proteins (Keep et al., 2018). After cerebral hemorrhage, blood-brain barrier dysfunction and structural damage will increase permeability, and water under the action of osmotic pressure through the damaged blood-brain barrier into the brain to form cerebral edema and aggravate the condition (Xi et al., 2001). We detected the EB content by Evans blue and verified that the peak of EB content coincided with the peak period of cerebral edema, and breviscapine can significantly reduce the damage of the blood-brain barrier, relieve cerebral vasospasm and improve brain microcirculation.
HE pathological experiments showed that breviscapine could inhibit the expressions of intercellular adhesion factors in inflammatory mediators and inflammation, reduce brain edema after cerebral hemorrhage, improve the ischemia and hypoxia of peripheral nerves of a cerebral hemorrhage, and promote the recovery of nerve function (Pengyue et al., 2017). The latest advances in molecular biology provide a new theoretical basis for us to understand the pathological mechanism of cerebral hemorrhage better (Gross et al., 2019). Molecular experiments showed that IL-6 and TNF-α levels are significantly increased after cerebral hemorrhage in rats (Jiang et al., 2017). Jiang et al. (2017) established a rat model of cerebral trauma caused by cortical control and injected breviscapine. It was found that breviscapine significantly improved neurobehavioral dysfunction after injection. And IL-6 expression was significantly down-regulated after breviscapine treatment. Some scholars have also found that breviscapine improving brain damage may be related to its inhibition of the levels of IL-6 and TNF-α (Wang et al., 2009). However, they only studied the effect of breviscapine on the myocardium and did not involve the pathological and molecular levels of brain tissue inflammation. Our experiments have demonstrated that breviscapine can significantly reduce inflammatory factor levels and reduce inflammation in brain tissue after cerebral hemorrhage in rats.
The extracellular matrix is an essential component of organ structure and function and is dynamically regulated by fibroblasts under normal conditions (Lu et al., 2014). As an extracellular matrix protein, Periostin plays a vital role in the development of various organ tissues (Izuhara et al., 2016; Landry et al., 2018). In an animal experiment, Liu et al. (2017) found that Periostin is up-regulated in brain tissues after subarachnoid hemorrhage, leading to early brain injury and that the underlying mechanism may be related to the activation of downstream signaling pathway and the interaction of cell-adhesin-C. In rats with cerebral hemorrhage, up-regulating Periodin can aggravate neurological deficits, cerebral edema, stimulate glial cell inflammation, and promote neuronal apoptosis (Matsunaga et al., 2015). Dong et al. (2017) found that compared with the control group, the serum Periostin protein concentration was significantly increased, and the Periostin concentration was independently related to the GCS score, indicating that the increase in serum Periostin protein concentration reflects the severity and mortality of trauma after TBI.
We examined the effects of cerebral hemorrhage and breviscapine inhalation on the changes of Periostin protein in rats, and the results showed that Periostin protein was abnormally increased after cerebral hemorrhage. This result is consistent with the results of Liu et al. (2014). More importantly, it was found that breviscapine significantly reduced the expression of Periostin, suggesting that breviscapine may lessen the degree of brain damage by regulating the expression level of Periostin. However, the specific molecular mechanism of breviscapine and Periodin in rats with cerebral hemorrhage remains obscure. Koh et al. (2016) found that Periostin regulates intestinal inflammation by activating NF-κB signaling, suggesting that Periostin is a potential target for inflammatory bowel disease. P65 is one of the subunits of the NF-κB family (DiDonato et al., 2012). Under the action of inflammatory factors, IKKε/TBK1 can phosphorylate p65 after activation to activate NF-κB to amplify the inflammatory cascade (DiDonato et al., 2012; Oeckinghaus et al., 2011). In our research we found that p65 expression was increased after cerebral hemorrhage in rats. This result also verified that Periodin could regulate the inflammation of cerebral hemorrhage by activating p65 in the NF-κB signaling pathway, and found that breviscapine inhalation significantly reduced the expression of p65, suggesting that breviscapine may improve brain damage through participating in the NF-κB pathway. Although our study found that breviscapine inhalation for brain injury may be related to Periodin and its NF-κB signaling pathway, further research is needed to examine its mechanism of action.
In conclusion, after cerebral hemorrhage, breviscapine inhalation treatment can significantly improve the degree of brain damage, which is possibly achieved by down-regulating the expression of Periostin to inhibit the NF-κB signaling pathway. The current findings provide a new theoretical basis for clinical breviscapine treatment of cerebral hemorrhage.
Substantial contributions to conception and design: ZC, CW. Data acquisition, data analysis, and interpretation: YL, XL, CY. Drafting the article or critically revising it for relevant intellectual content: ZC, XZ, XL. Final approval of the version to be published: All authors.
Agreement to be accountable for all aspects of the work in ensuring that questions related to the accuracy or integrity of the work are appropriately investigated and resolved: XL.
All animal experiments were performed under the guidelines of the China Council on Animal Care and Use. The Committee approved this study of Experimental Animals of The First Affiliated Hospital of Zhejiang Chinese Medical University (S201901006). Possible efforts have been made to minimize the pain and discomfort to the animals. The animals’ experiments were performed in the First Affiliated Hospital of Zhejiang Chinese Medical University.
This work was supported by the Traditional Medical Science and Technology Project of Zhejiang Province [Grant Number 2017ZA049]. Thanks for the financial support.
The authors declare no competing interests.