The threshold for conscious perception of stimuli within the environment varies from individual to individual. Functional neuroimaging studies have suggested that insular cortex activity is positively correlated with perceptual awareness. However, few studies have tested the relationship between awareness and structural variability in the insula. The purpose of this study was to examine structural differences in brain morphology related to perceptual awareness of fearful faces. This study hypothesized that there would be a positive correlation between insular grey matter volume and scores on the forced-choice awareness check task. The forced-choice awareness check task was designed to assess awareness for the presence and location of backward masked fearful and neutral faces, masked with neutral faces. The participants responded by indicating the side on which the masked fearful face appeared, or whether there were two neutral faces. The task included a total of 60 trials. T1-weighted magnetic resonance images were collected to measure grey matter volumes. Individuals that were more aware of backward masked fearful faces had greater grey matter volume in the insula, middle cingulate, anterior temporal pole, ventral striatum, and hippocampus.
The environment is filled with potential threats, and responding quickly to such threats is necessary for survival. Spatial attention has been shown to orient to threatening faces during unmasked tasks where awareness was not restricted (Armony:2002, Pourtois:2004) and when awareness was restricted by means of backward masking (Carlson:2008, Mogg:2002, Fox:2002). Research has suggested that a network of brain regions including the amygdala, ventral anterior cingulate cortex (ACC), and visual cortex, is responsible for orienting spatial attention toward backward masked fearful faces (Carlson:2009). In addition, this attentional bias toward backward masked fearful faces is correlated with variability in the gray matter volume of a more dorsal ACC region (Carlson:2012). Typically, backward masking is an effective method for restricting awareness of a stimulus; however, not all humans have the same level of conscious perceptual awareness (Pessoa:2005).
Pessoa et al. (Pessoa:2005) conducted a study examining the restriction of awareness to facial expressions using a backward masking technique. They varied the duration that the target face (fearful, happy, or neutral; 17, 33, and 83 ms) and the masking face (neutral; 116, 100, and 50 ms) were presented. Participants were asked to indicate whether a fearful face was presented. Their results revealed that most of the participants could correctly identify the fearful face when the target face was presented for 83 ms, 36% were able to detect the fearful face at 33 ms, and few were able to identify the fearful face at 17 ms. Another study indicated that one out of 15 participants was able to correctly identify fearful faces that were presented for 33ms and then backward masked (Carlson:2009). These studies suggest that there are individual differences in perceptual awareness of fearful facial expressions.
Neuroimaging research suggested that the insula is functionally involved in awareness. Craig (Craig:2009) offered a detailed review of the ways the insula contributes to aspects of awareness, e.g. body movements, self-recognition, emotion, attention, and perception of time. In emotional awareness, the insula is activated while viewing emotional facial expressions (i.e. disgust) and while experiencing the emotion (i.e. smelling an unpleasant odor (Craig:2009, Hennenlotter:2005)). Critchley et al. (Critchley:2002) used functional magnetic resonance imaging to investigate awareness of angry faces in a conditioned fear paradigm. They determined that activity in the insula was modulated by awareness of threatening stimuli. Although Critchley et al. (Critchley:2002) examined differences in insular activity related to awareness and conditioned fear, few studies have addressed any corresponding anatomical differences in grey matter volume. However, one recent study by Getov et al. (Getov:2015) examined individual differences in grey matter volume in the insula, in terms of preconscious evaluation of dominant faces. They found that reduced grey matter volume in the insula was correlated with slower responses to dominant faces (i.e. less perceptual awareness).
Together, the ACC and anterior insular cortex (AIC) make up the salience network (Menon:2010). This network is involved in identifying salient stimuli in the internal and/or external environment, to guide behavioral responses. Menon and Uddin (Menon:2010) acknowledged multiple roles that the AIC plays in the salience network. The AIC is involved in switching between the default mode network and the central executive network. They also indicated the difference between the functional connectivity of the AIC and the mid-posterior insula. In particular, the AIC is functionally connected to the pregenual ACC and the anterior and posterior mid-cingulate cortex (aMCC and pMCC); whereas, the mid-posterior insula is functionally connected to the posterior mid-cingulate cortex (Taylor:2009).
Given that previous research found evidence suggesting that there are structural differences in the ACC associated with attentional bias to fearful faces, (Carlson:2012) and that the ACC and AIC are major structures involved in the salience network, it is reasonable to question if there are structural differences in the AIC and ACC associated with awareness of fearful faces. The purpose of this study was to examine the relationship between AIC and ACC grey matter volume and differences of perceptual awareness. Discrepancies in grey matter volume could help to explain the individual differences in perceptual awareness of emotional facial expressions. We hypothesized that there would be a positive correlation between AIC and ACC grey matter volume and scores on the forced-choice awareness check task. Grey matter volumes were examined using voxel-based morphometry (VBM). In addition, cortical thickness and gyrification were examined using surface-based morphometry (SBM) on structural magnetic resonance images (sMRI).
The current study included 42 participants (25 male; 17 female), ranging in age from 19 to 25 years old (M = 21.2, SD = 1.3). Thirty-seven of these individuals were right-handed. All potential participants underwent screening, to check for metal in their bodies. This study was approved by the Institutional Review Board of Stony Brook University. Monetary compensation was awarded to all participants (
The awareness task, programed and presented using E-Prime (Psychology Software Tools, Pittsburg, PA), took place in a controlled testing area outside of the scanner. The task was displayed using a 60 Hz PC monitor. Greyscale images of the initial (i.e., masked) fearful and neutral faces (half male) were taken from four unique individuals (Gur:2002). A fifth identity from the same facial database was used as a backward mask. The mask image displayed a female with an open-mouthed happy facial expression. At the beginning of each trial, a white fixation cue (+) at the center of the screen was shown against a black background for 1000ms. Next, a pair of facial stimuli were concurrently presented (33 ms) to the left and right of the point of fixation. The stimuli were subtended roughly 5 × 7° of visual angle and partitioned from one another by 14° of visual angle. A mask, which was offset by 1° of visual angle on the vertical axis, immediately replaced the initial stimuli for 100 ms. Participants were prompted to report whether they saw a fearful face on the left, on the right, two neutral faces (see Fig. 1). Before the task began, all participants were informed that they would be reporting facial expressions from the first of two sets of faces, presented in rapid succession during each trial. The task covered 20 trials of each type, for a total of 60 trials.
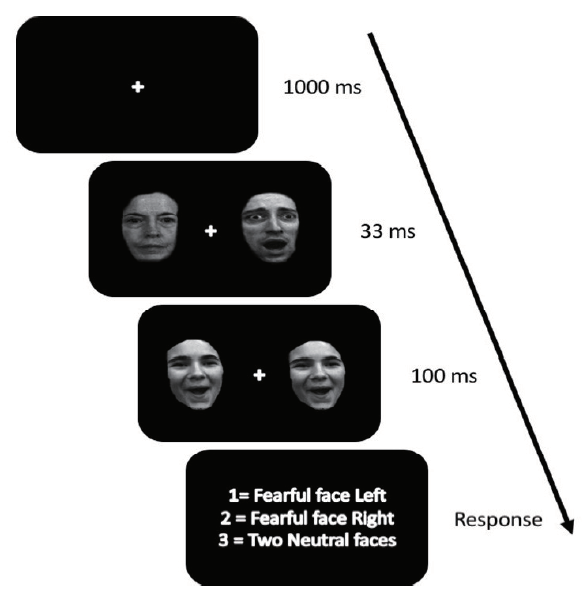
This figure shows the progression of the awareness check task. There was a fixation cue for 1,000 ms, then two faces (either fearful or neutral) for 33 ms which were immediately replaced by two happy faces for 100 ms. Then a prompt appeared asking the participants if they saw a fearful face on the right, the left, or there was no fearful face.
T1 images with the parameters: TR = 1900 ms, TE = 2.53, flip angle = 9°, FOV = 176 × 250 × 250 mm, matrix = 176 × 256 × 256, and voxel size = 1 × 0.98 × 0.98 mm, were obtained using a 3 Tesla Siemens Trio whole body scanner. Automated user-independent voxel-wise measurement of correlations between individual differences and localized brain volumes were made possible using VBM. Comparison with manual volumetric analysis has consistently validated the VBM method, (Woermann:1999) which transforms distinct structural MR volume images into a collective stereotactic space. The differences in relative volume of individual brain regions can be subsequently assessed through general linear model statistics.
The VBM procedure utilized in this study resembles previously described methodologies (Ashburner:2000). The anterior commissure was initially used as a point of origin, to manually align volume brain images prior to processing in SPM8, using recognized techniques (http://www.fil.ion.ucl.ac.uk/spm). Visual inspection of three-dimensional T1-weighted MPRAGE images was used to identify artifacts and structural anomalies. Next, grey matter, white matter, and cerebrospinal fluid were segmented from the initial MR images, prior to a second visual examination. Brain images were normalized onto standard MNI space. Voxel-wise data about regional tissue was permitted through a modulation step (Ashburner:2000). An 8-mm FWHM Gaussian smoothing kernel was used while averaging across participant data, in order to generate tissue probability maps. Summed global signal of segmented images of grey and white matter allowed for the assessment of full brain volume. Regression analysis was performed on smoothed grey matter images using accuracy on the awareness task as the predictor variable. Age and whole brain grey matter volume were used as covariates. A whole brain threshold of p < .001 and 20 voxels was initially used and a small volume correction (SVC) was then applied to the AIC (8 mm sphere centered at $\pm$ 34, 20, 4 (Carlson:2010, Critchley:2004)) and the ACC (4 mm sphere centered at 16, 40, 20 and -8, 36, 16 (Carlson:2012)). A regression was used to create standardized residuals of insular grey matter volume, controlling for age and whole-brain grey matter volume. Masks for Regions of Interest Analysis software (Bertram:2003) was used to identify MNI coordinates in the whole brain analysis. An exploratory analysis was conducted to examine other brain areas related awareness using an uncorrected whole brain threshold of p < .001 and 20 voxels.
After completing VBM, the T1-weighted images were used for SBM in the CAT12 software (http://www.neuro.uni-jena.de/cat/). The data were segmented for surface and thickness estimations. The data was smoothed using a kernel smoothing filter of full width at half maximum (FWHM) of 15 mm. The gyrification index was extracted based on the absolute mean curvature. A regression analysis was used to examine differences in cortical thickness and gyrification, separately, while controlling for age and whole-brain grey matter volume. A threshold of p < .001 and the neuromorphometrics atlas with a left and right insula mask in SMP12 was used to determine differences in the insular. An unmasked exploratory analysis was done with an uncorrected threshold of p < .001 in CAT12.
All 42 participants scored within chance level (M = 33.88%, SD = 4.76, range = 25% - 43%, t(42) = 0.75) in the forced-choice awareness check task. This may indicate that the fearful faces were displayed below conscious level for all the participants. Although no one scored outside chance level, there was peri-threshold variability of awareness scores.
After applying the SVC 8 mm sphere centered at x = $\pm$ 34, y = 20, z = 4, we found significant results for the left AIC, t(38) = 3.77, r = .552, $p_{FWE-corr}$ = .014, $voxels_{-uncorr}$ = 21 and for the right AIC, t(38) = 3.81, r = .603, $p_{FWE-corr}$ = .013, voxels$_{-uncorr} $= 108 (see Fig. 2). The results indicated that individuals who scored higher on the awareness task had greater grey matter volume in the bilateral AIC. No significant differences between awareness scores and bilateral ACC. An exploratory whole-brain analysis indicated that the additional following areas had greater grey matter volume in participants that scored higher on the awareness check task (see Table 1).
Region | Hemisphere | MNI coordinates | Voxels | t-value | ||
---|---|---|---|---|---|---|
x | y | z | ||||
Middle Cingulate | L R | 10 | 16 | 36 | 135 | 4.55 |
Anterior Insula/Inferior Frontal Gyrus | R | 46 | 26 | -6 | 108 | 4.49 |
Anterior Insula | L | -34 | 28 | 0 | 21 | 3.85 |
Anterior Temporal Pole | L | -30 | 10 | -28 | 55 | 4.05 |
Posterior Insula | R | 42 | -4 | -12 | 191 | 3.93 |
Ventral Striatum/Bed Nucleus of the Stria Terminalis | L R | 0 | 2 | 2 | 88 | 3.74 |
Hippocampus | R | 20 | -10 | -16 | 44 | 3.82 |
The table shows the results from the whole brain analysis. The whole-brain threshold was p < .001 and 20 voxels. R = Right and L = Left.
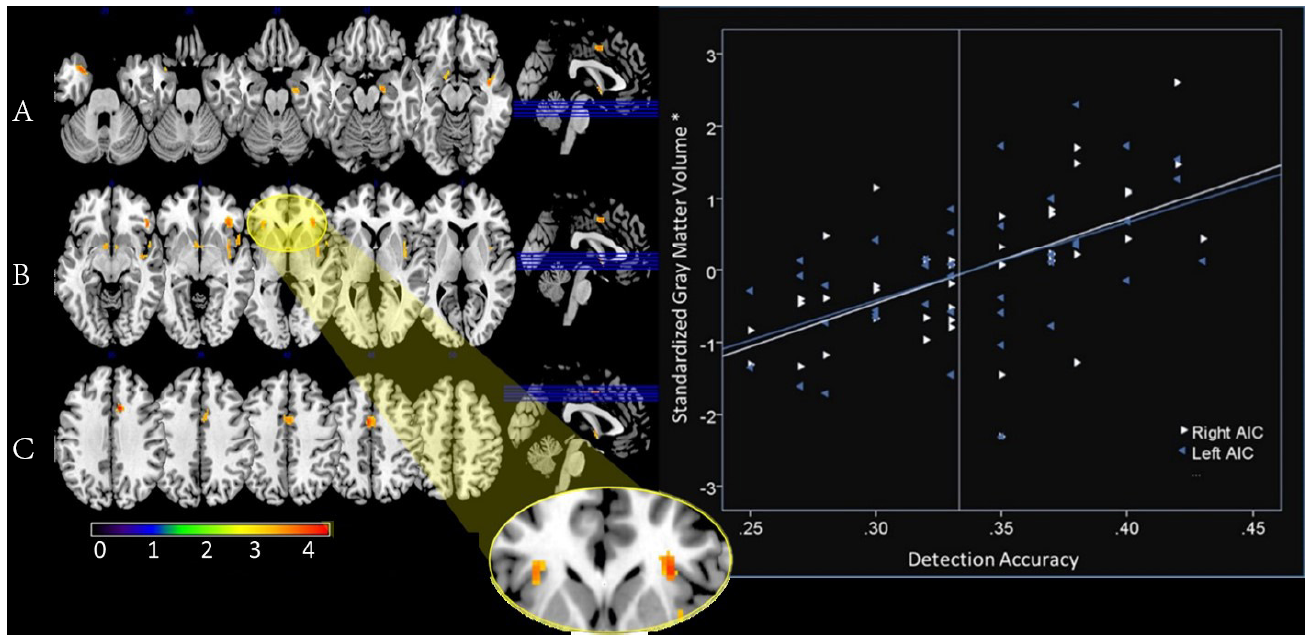
Left: This figure shows the results of the whole brain analysis with p value threshold < .001 and a voxel threshold of 20 voxels. Numbers above the brain slices indicate y axis coordinates. Color bar on bottom indicates t-value. The highlighted areas are reported in the Table 1. These are the areas correlated with greater grey matter volume and greater scores on the forced-choice awareness check task. Y coordinates from left to right A) -29, -25, -21, -17, -13; B) -9, -5, -1, 3, 7; C) 35, 39, 42, 46, 50. Right: The graph shows the correlation between insula grey matter volume and scores on the awareness check task. Left AIC, r = .552, and right AIC, r = .603. *A regression was used to create standardized residuals of the insula grey matter volume controlling for age and whole-brain grey matter volume.
The data did not yield any significant differences in cortical thickness and gyrification in the insula. Additionally, a whole brain exploratory analysis did not yield any significant differences at p < .001.
The results suggest that there is a significant relationship between AIC, but not ACC, grey matter volume and performance scores on the awareness check task with fearful faces. Specifically, there was greater grey matter volume in the AIC in participants that had greater accuracy on the awareness task, which indicated that the volume of the AIC may contribute to differences in perceptual awareness of fearful facial expressions. There was no correlation between awareness scores and cortical thickness and gyrification. Participants performed at similar recognition rates to fearful faces in the backward masking paradigm, as previously reported in the literature (Carlson:2008, Mogg:2002, Fox:2002). Performance rates in some of the participants were considered to be peri-threshold, indicating that in terms of statistics, all participants performed at chance level. Our results were not consistent with previous research, which demonstrated that some individuals could recognize fearful faces as quickly as 17ms (Pessoa:2005). However, even within the peri-threshold awareness scores, there was a positive correlation with AIC grey matter volume (see Fig. 2).
Previous research has shown that the insula is involved in response to negative emotional stimuli and awareness (Craig:2009, Hennenlotter:2005). Our data further suggest that there is a relationship between increased grey matter volume in the AIC and awareness of fearful faces in a forced-choice awareness task. This variation in grey matter could provide an explanation of individual differences in performance on awareness tasks (Pessoa:2005). It seems reasonable to suggest that the insula is sensitive to environmentally and evolutionally salient stimuli, fearful faces, and that an increase in grey matter leads to increased performance in recognition of fearful faces at lower levels of awareness. Recent research in rodents using MRI measures of gray matter volume in conjunction with confocal microscopy of ex vivo brain sections indicates that differences in VBM measures of gray matter volume are linked to differences in dendritic spine density in the underlying tissues (rather than a number of other potential mechanisms) (Keifer:2015). Thus, it appears that variability in MRI measures of gray matter volume are most likely reflective of differences in dendritic spine density. Given this and given that dendritic spine morphology is reflective of the relative strength of afferent connectivity, our results may indicate that AIC input connectivity (i.e., higher numbers of dendritic spines) is relatively greater in individuals scoring higher on the awareness task, which would be consistent with the role of the AIC as an integrative hub for multimodal inputs related to the subjective experience of awareness across sensory domains (Craig:2009). On the other hand, we did not observe a relationship between awareness scores and cortical thickness nor gyrification measures. In a post-mortem study of multiple sclerosis patients, MRI measures of cortical thickness correlated with cell density and myelination, (Kolasinski:2012) which are related to the number of computational units in a local network and the output connectivity of the local network, respectively. Thus, when the pattern of results in the current study is interpreted in the context of studies assessing the cellular level correlates of MRI-based morphometry measures, it appears that increased awareness is specifically linked to increased input connectivity in the AIC rather than an increase in the number of cells in or output from the AIC.
The VBM results of this research add to the literature describing the structural role of the AIC in the salience network (Ham:2013). Whereas previous research suggested that the AIC was functionally involved in attention toward salient stimuli (Menon:2010, Taylor:2009, Ham:2013). Our results suggest that structural differences within this system are involved in awareness of salient stimuli. Carlson et al. (Carlson:2012), noted that ACC grey matter volume positively correlated with the direction of spatial attention toward fearful facial expressions; however, they did not find that differences in AIC grey matter volume were related to spatial attention, which is independent of awareness. In the current study, whole-brain analysis revealed a positive correlation between the MCC and awareness scores, but there was no relationship in the ACC (see Table 1). This is consistent with Taylor et al. (Taylor:2009), who suggested that the AIC was functionally connected to the MCC. Additionally, our finding that structural variability within the AIC, and potentially the MCC, is linked to the level of awareness for backward masked fearful faces suggests that broader salience network may underlie this behavior.
Although the results of this study provide new evidence that structural differences in the AIC contribute to differences in perceptual awareness toward fearful facial expressions, they did not come without limitations. The forced-choice awareness check task was not specifically designed to manipulate awareness. Instead, we used previously collected and published data to examine structural differences in awareness. Future research should design a study that varied the amount of time that the masked faces were displayed, similar to Pessoa et al. (Pessoa:2005), and collect MRI data. This would parse out the variations in individual perceptual awareness. Another limitation was that only fearful facial expressions were used. Future research could use other facial expressions for the masked faces, such as happy and angry. Nevertheless, the results provide novel evidence that AIC GMV is related to individual difference in awareness toward fearful facial expressions.
The authors thank Eddie Harmon-Jones and Greg Hajack for acquiring the data used here.
The authors declare no competing interests.