Academic Editors: Carmela Rita Balistreri and Teruo Inoue
Background: Kawasaki disease (KD) is a well-known systemic inflammatory vasculitis. Endothelial dysfunction is one of most easily overlooked non-coronary complications of KD. Several studies have assessed endothelial dysfunction using flow-mediated dilatation (FMD), nitroglycerin-mediated dilation (NMD), and biomarkers (E-selectin, P-selectin, intercellular adhesion molecule-1 (ICAM-1), and vascular cellular adhesion molecule-1 (VCAM-1)). However, the results were inconsistent and incomplete. Methods: We searched five databases for eligible studies until March 8, 2022. The summarized weighted mean difference (WMD) with 95% confidence intervals (CIs) were estimated for FMD, NMD, and four biomarkers level between KD and healthy children. A meta-analysis with subgroup analysis was conducted. Results: 40 studies with a total of 2670 children (1665 KD patients and 1005 healthy children) were identified. During the acute phase, KD patients had lower FMD compared to the control group (WMD = –10.39, 95% CI: –13.80– –6.98). During the subacute phase, KD patients had lower FMD compared to the control group (WMD = –15.07, 95% CI: –17.61– –12.52). During the convalescence phase, KD patients had lower FMD and similar NMD compared to the control group (WMD = –4.95, 95% CI: –6.32– –3.58; WMD = –0.92, 95% CI: –2.39–0.55, respectively). During the convalescence phase, those KD patients without coronary artery lesion (CAL), with CAL, even with coronary artery aneurysm, had progressively lower FMD compared to healthy children (WMD = –3.82, 95% CI: –7.30– –0.34; WMD = –6.32, 95% CI: –7.60– –5.04; and WMD = –6.97, 95% CI: –7.99– –5.95, respectively). Compared to KD patients without CAL, those with CAL had lower FMD (WMD = –1.65, 95% CI: –2.92– –0.37). KD patients had higher levels of E-selectin, P-selectin, and ICAM-1 compared to healthy controls during different phases. KD patients had a higher level of VCAM-1 compared to healthy controls only during the acute phase (WMD = 61.62, 95% CI: 21.38–101.86). Conclusions: Endothelial dysfunction is present since the onset of KD and persists for years, confirmed by the measurement of FMD and biomarkers from different phases. An assumption is advanced that FMD impairment (the severity of endothelial dysfunction) may be positively correlated with CAL severity during the convalescence phase.
Kawasaki disease (KD) is a well-known, self-limited, systemic inflammatory vasculitis, usually occurs in children between 6 months and 4 years of age. Among children in developed countries, KD has become the most common form of acquired cardiac disease [1]. The incidence of KD was estimated to be approximately 72–319 per 100,000 children in Asians, and 18–21 in the USA [2].
KD can lead to infiltration of inflammatory cells into small- and medium-sized arteries, particularly the coronary arteries. Up to 25% of KD patients can develop coronary artery aneurysm (CAA) unless they receive timely treatment with intravenous immunoglobulin [3]. Giant CAA could lead to coronary artery thrombosis, stenosis, or occlusion [4].
Endothelial dysfunction in KD by endothelial biomarkers (E-selectin, P-selectin, intercellular adhesion molecule-1 (ICAM-1), and vascular cellular adhesion molecule-1 (VCAM-1)) was identified as early in 1992 [5]. Afterwards the interest of investigation gradually increased from 1994–1999 [6, 7, 8, 9] to 2004–2018 [10, 11, 12, 13, 14, 15, 16, 17, 18, 19]. Endothelial dysfunction in KD by flow-mediated dilatation (FMD) and nitroglycerin-mediated dilation (NMD) stared in 1996 [20], since then the research of FMD continued to increase from 2001 to 2021 [21, 22, 23, 24, 25, 26, 27, 28, 29, 30, 31, 32, 33, 34, 35, 36, 37, 38, 39, 40, 41, 42, 43, 44]. More recently, Routhu et al. [43], performed assessment of endothelial dysfunction in acute and convalescent phases of KD using automated edge detection software. Wen et al. [44], also focused on the predictive value of brachial artery FMD on coronary artery abnormality in acute stage of KD.
Although the cause of endothelial dysfunction in KD remains unknown, endothelial
activation and endothelial dysfunction are presumably due to increased cytokine
production (e.g., IL-1, IL-6, and TNF) by immune effector cells via the
NF-
At the present, due to the absence of pathognomonic tests (a specific diagnostic test), the diagnosis continues to rest on the identification of principal clinical findings and the exclusion of other clinically similar entities with known causes. Therefore, FMD, NMD, and endothelial biomarkers will be instrumental in support to make KD diagnosis (in particular, in suspected atypical incomplete KD) [1], because of endothelial dysfunction as an early determinant of vascular disease [47]. In this review, we will discuss the emerging evidence demonstrating the clinical significance of FMD, NMD and endothelial biomarkers to KD during different (acute/subacute/convalescence) phases.
This study was performed following a prospectively registered protocol in the PROSPERO database (CRD42022315266). This paper was reported in accordance with PRISMA guideline (Supplementary Table 1).
Two independent investigators (XY and DW) independently searched PubMed, MEDLINE, Embase, Cochrane library, and China National Knowledge Infrastructure (CNKI, China Core Journal Database) from database inception to March 8, 2022, to identify the relevant studies. The following search keywords included “Kawasaki disease” and (“flow-mediated dilatation”, “nitroglycerin-mediated dilation”, “E-selectin”, “P-selectin”, “intercellular adhesion molecule-1”, or “vascular cellular adhesion molecule-1”) [48, 49]. At the same time, we read the references of articles, trying to find potential literature which may meet the criteria.
Two researchers (XY and DW) independently screened the titles and abstracts for eligibility. Full papers were assessed to confirm disagreement in existence according to the exclusion criteria by the two researchers. Disagreements were discussed and resolved by involving a third reviewer (GS) for adjudication. Original studies were eligible if the following criteria were met: (i) observational study; (ii) the study investigated FMD/NMD or biomarkers in KD children compared to healthy participants; (iii) full text in English and Chinese available; (iv) the data of the acute phase must be acquired before intravenous immunoglobulin/aspirin treatment. Original studies were ineligible if the following criteria existed: (i) reviews, case reports, or case series; (ii) did not report the data necessary for calculating the mean and standard deviation of FMD/NMD or biomarkers level; (iii) animal studies; (iv) adult patients with a history of KD. If there were several publications from the same study, the study with the most cases and relevant information was included.
The extracted data included the first author of involved studies, year of publication, country, groups, participant number, gender, mean age, time from KD onset, treatment, measurements of endothelial function, and whether the involved studies contain coronary artery lesion (CAL) group. Numeric data were gathered directly from tables or, when presented in graphs only, were inferred by digitizing the figure with GetData Graph Digitizer 2.26 [50]. The quality assessment was performed by the Newcastle–Ottawa Scale (NOS) assessment tool with the score 0–9. A score of 6 or more were considered to be high-quality studies.
The pooled effects are presented as the weighted mean difference (WMD) with 95%
confidence intervals (CIs). Heterogeneity was assessed using the I
We had searched for potentially relevant publications from five sources. After applying the inclusion and exclusion criteria, 40 studies were identified (Fig. 1) [5, 6, 7, 8, 9, 10, 11, 12, 13, 14, 15, 16, 17, 18, 19, 20, 21, 22, 23, 24, 25, 26, 27, 28, 29, 30, 31, 32, 33, 34, 35, 36, 37, 38, 39, 40, 41, 42, 43, 44].
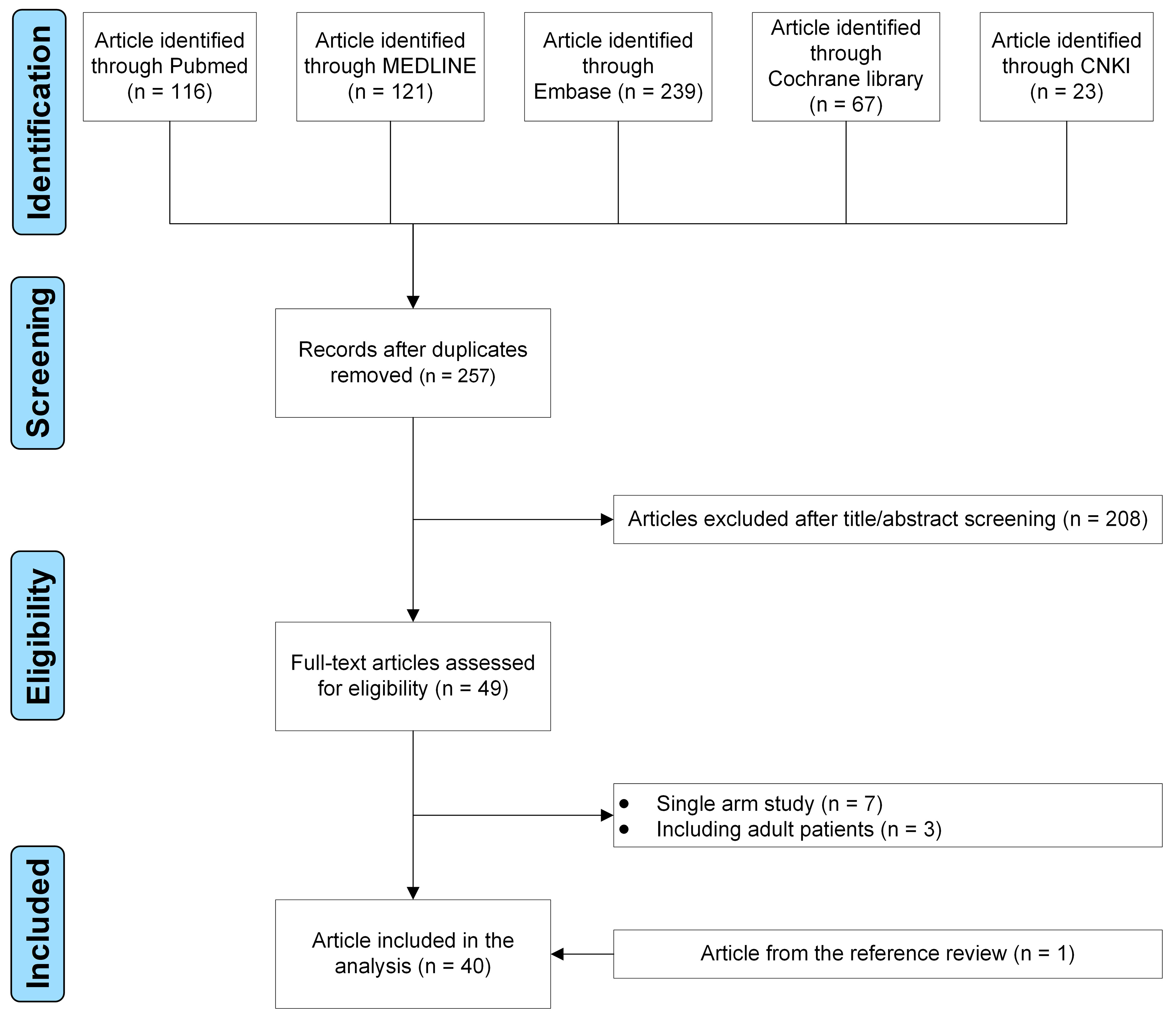
The flow diagram of the study selection process.
The baseline characteristics of the included studies are shown in Table 1 (Ref. [5, 6, 7, 8, 9, 10, 11, 12, 13, 14, 15, 16, 17, 18, 19, 20, 21, 22, 23, 24, 25, 26, 27, 28, 29, 30, 31, 32, 33, 34, 35, 36, 37, 38, 39, 40, 41, 42, 43, 44]). All studies were published between 1992 and 2021. Studies were conducted in Europe (Italy and UK), America (Canada and Chile), and Asia (China, India, Iran, Japan, Korea, and Turkey). 2670 children were included: 1665 children with KD and 1005 healthy participants. Twenty-five studies evaluated FMD, eight with NMD. Fifteen studies reported the difference of these four biomarkers between KD and control groups. Twenty of the involved studies contained CAL group. Quality assessment is shown in Supplementary Table 2. Details of flow-mediated dilation measurement in the involved studies are shown in Supplementary Table 3.
Study | Year | Country | Group | Number | Male/female | Mean age (year) | Time from KD onset | Treatment | Measurement | CAL subgroup | NOS score |
Furukawa et al. [5] | 1992 | Japan | KD | 29 | 15/14 | 1.8 |
Acute phase: 2–9 days | 29/29 received aspirin, 27/29 received IVIG | ICAM-1 | No | 8 |
Convalescent phase: 20–194 days | |||||||||||
Healthy control | 10 | 6/4 | 2.00 | – | – | ||||||
Kim et al. [6] | 1994 | Korea | KD | 24 | 13/11 | 2.8 |
Acute phase: 3–9 days | All patients received IVIG and aspirin | E-selectin | No | 8 |
Subacute phase: 15–42 days | |||||||||||
Healthy control | 10 | NR | NR | – | – | ||||||
Nash et al. [7] | 1995 | UK | KD | 59 | 32/27 | 2.5 |
Acute phase: 0–28 days | NR | E-selectin, ICAM-1, VCAM-1 | No | 8 |
Healthy control | 48 | 37/11 | 4.9 |
– | – | ||||||
Dhillon et al. [20] | 1996 | UK | KD | 20 | 12/8 | 13.0 |
Convalescent phase: 5.3–17.1 years | 18/20 received aspirin, 3/20 received IVIG | FMD, NMD | No | 7 |
Healthy control | 20 | NR | 15.0 |
– | – | ||||||
Takeshita et al. [8] | 1997 | Japan | KD | 16 | 7/9 | 1.6 |
Acute phase: 3–7 days | All patients received IVIG and aspirin | E-selectin, P-selectin, VCAM-1 | No | 8 |
Subacute phase: 11–19 days | |||||||||||
Convalescent phase: 28–41 days | |||||||||||
Healthy control | 10 | 6/4 | 3.9 |
– | – | ||||||
Schiller et al. [9] | 1999 | Italy | KD | 30 | 20/10 | 2.8 |
Acute phase: before treatment | All patients received IVIG and aspirin | E-selectin, ICAM-1 | No | 8 |
Subacute phase: 1–2 days after treatment | |||||||||||
Convalescent phase: 42–90 days | |||||||||||
Healthy control | 15 | 9/6 | 7.1 |
– | – | ||||||
Silva et al. [21] | 2001 | Canada | KD | 24 | 18/6 | 14.3 |
Convalescent phase: 11.3 |
23/24 received aspirin, 14/24 received IVIG | FMD, NMD | No | 8 |
Healthy control | 11 | 6/5 | 14.1 |
– | – | ||||||
Deng et al. [22] | 2002 | China | KD | 39 | 28/11 | 7.1 |
Convalescent phase: 1.0–10.0 years | All patients received aspirin, 34/39 received IVIG | FMD, NMD | Yes | 9 |
Healthy control | 17 | 13/4 | 7.0 |
– | – | ||||||
Qiu et al. [10] | 2004 | China | KD | 36 | 21/15 | 1–4 | Acute phase: 3–7 days | All patients received IVIG and aspirin | E-selectin, P-selectin | Yes | 9 |
Subacute phase: 11–19 days | |||||||||||
Convalescent phase: 28–41 days | |||||||||||
Healthy control | 30 | NR | NR | – | – | ||||||
Kadono et al. [23] | 2005 | Japan | KD | 24 | 13/11 | 8.3 |
Convalescent phase: 5.8 |
2/24 received aspirin | FMD | Yes | 8 |
Healthy control | 41 | 29/12 | 10.7 |
– | – | ||||||
Sun_1 et al. [24] | 2005 | China | KD | 22 | 16/6 | 5.3 |
Convalescent phase: 1.8 |
NR | FMD | Yes | 7 |
Healthy control | 16 | 11/5 | 5.5 |
– | – | ||||||
Sun_2 et al. [25] | 2005 | China | KD | 36 | 24/12 | 2.9 |
Acute phase: less than one month | NR | FMD | No | 6 |
Healthy control | 15 | 9/6 | 3.2 |
– | – | ||||||
Zhang et al. [11] | 2005 | China | KD | 26 | 20/6 | 1.8 |
Acute phase: before treatment | All patients received IVIG | E-selectin | Yes | 7 |
Subacute phase: 3 days after treatment | |||||||||||
Healthy control | 15 | 9/6 | 2.1 |
– | – | ||||||
Wang et al. [12] | 2006 | China | KD | 20 | 11/9 | 0.8–5 | Acute phase: before treatment | All patients received IVIG and aspirin | E-selectin, ICAM-1 | No | 6 |
Subacute phase: 1–3 days after treatment | |||||||||||
Healthy control | 19 | 11/8 | 0.9–5 | – | – | ||||||
Li et al. [13] | 2007 | China | KD | 34 | 23/11 | 1.1 |
Acute phase: before treatment | All patients received IVIG and aspirin | VCAM-1 | No | 7 |
Convalescent phase: NR | |||||||||||
Healthy control | 26 | 14/12 | 1.4 |
– | – | ||||||
Liu et al. [26] | 2007 | China | KD | 101 | 78/33 | 6.8 |
Convalescent phase: 4.4 |
NR | FMD | Yes | 8 |
Healthy control | 103 | 64/39 | 7.2 |
– | – | ||||||
McCrindle et al. [27] | 2007 | Canada | KD | 52 | 35/17 | 15.5 |
Convalescent phase: 11.2 |
48/52 received aspirin, 33/52 received IVIG | FMD | Yes | 9 |
Healthy control | 60 | 30/30 | 14.9 |
– | – | ||||||
Borzutzky et al. [28] | 2008 | Chile | KD | 11 | 7/4 | 10.6 |
Convalescent phase: 8.1 |
All patients received IVIG and aspirin | FMD | No | 8 |
Healthy control | 11 | 7/4 | 10.4 |
– | – | ||||||
Huang et al. [29] | 2008 | China | KD | 11 | 8/3 | 12.9 |
Convalescent phase: 10.8 |
All patients received IVIG and aspirin | FMD | Yes | 9 |
Healthy control | 11 | 8/3 | 13.0 |
– | – | ||||||
Xu_1 et al. [14] | 2008 | China | KD | 40 | 27/13 | 2.3 |
Acute phase: before treatment | NR | VCAM-1 | No | 6 |
Subacute phase: 5–7 days after treatment | |||||||||||
Healthy control | 30 | NR | NR | – | – | ||||||
Xu_2 et al. [15] | 2008 | China | KD | 40 | 27/13 | 2.3 |
Acute phase: before treatment | NR | P-selectin | No | 6 |
Subacute phase: 5–7 days after treatment | |||||||||||
Healthy control | 30 | NR | NR | – | – | ||||||
Ghelani et al. [30] | 2009 | India | KD | 20 | 13/7 | 8.4 |
Convalescent phase: 0.25–6.5 months | All patients received IVIG and aspirin | FMD | No | 8 |
Healthy control | 20 | 13/7 | 8.6 |
– | – | ||||||
Liu et al. [31] | 2009 | China | KD | 41 | 25/16 | 7.1 |
Convalescent phase: 1.5–10 years | 21/41 received aspirin, 41/41 received IVIG | FMD | Yes | 9 |
Healthy control | 22 | 13/9 | 8.4 |
– | – | ||||||
Chen et al. [16] | 2010 | China | KD | 148 | 98/50 | 2.2 |
Acute phase: 3–7 days | All patients received IVIG and aspirin | E-selectin | Yes | 9 |
Subacute phase: 11–19 days | |||||||||||
Convalescent phase: 28–41 days | |||||||||||
Healthy control | 20 | 14/6 | 2.0 |
– | – | ||||||
Straface et al. [17] | 2010 | Italy | KD | 12 | NR | 0.5–2 | Acute phase: before treatment | All patients received IVIG and aspirin | P-selectin | No | 8 |
Healthy control | 5 | NR | NR | – | – | ||||||
Duan et al. [32] | 2011 | China | KD | 31 | 22/9 | 6.2 |
Convalescent phase: 1–12.5 years | NR | FMD, NMD | Yes | 8 |
Healthy control | 21 | 14/7 | 5.7 |
– | – | ||||||
Liu et al. [18] | 2013 | China | KD | 271 | 182/89 | 2.9 |
Acute phase: before treatment | All patients received IVIG | ICAM-1 | No | 8 |
Subacute phase: 1–2 days after treatment | |||||||||||
Healthy control | 36 | 21/15 | 3.2 |
– | – | ||||||
Ishikawa et al. [33] | 2013 | Japan | KD | 24 | 14/10 | 6.5 |
Convalescent phase: 1–4.1 years | 4/24 received aspirin, 24/24 received IVIG | FMD, NMD | Yes | 9 |
Healthy control | 22 | 13/9 | 7.9 |
– | – | ||||||
Ding et al. [34] | 2014 | China | KD | 28 | 16/12 | 1.9 |
Acute phase: 1–11 days | All patients received IVIG and aspirin | FMD | No | 8 |
Subacute phase: 11–21 days | |||||||||||
Convalescent phase: |
|||||||||||
Healthy control | 28 | 16/12 | 1.8 |
– | – | ||||||
Duan et al. [35] | 2014 | China | KD | 13 | 13/0 | 5.8 |
Convalescent phase: 1.5–7 years | All patients received IVIG and aspirin | FMD, NMD | Yes | 9 |
Healthy control | 14 | 14/0 | 5.5 |
– | – | ||||||
Laurito et al. [36] | 2014 | Italy | KD | 14 | 9/5 | 10.0 |
Convalescent phase: 6.3 |
NR | FMD | Yes | 8 |
Healthy control | 14 | 7/7 | 10.2 |
– | – | ||||||
Gao et al. [37] | 2015 | China | KD | 50 | 35/15 | 2.0 |
Acute phase:1–11 days | NR | FMD | Yes | 9 |
Subacute phase: 11–21 days | |||||||||||
Convalescent phase: |
|||||||||||
Healthy control | 19 | 11/8 | 2.0 |
– | – | ||||||
Sabri et al. [38] | 2015 | Iran | KD | 16 | 7/9 | 12.1 |
Convalescent phase: 5.8 |
NR | FMD | No | 7 |
Healthy control | 19 | 10/9 | 12.6 |
– | – | ||||||
Mori et al. [39] | 2016 | Japan | KD | 67 | 36/31 | 9.5 |
Convalescent phase: 7.5 |
NR | FMD | Yes | 7 |
Healthy control | 28 | 16/12 | 8.6 |
– | – | ||||||
Parihar et al. [40] | 2017 | India | KD | 20 | 12/8 | 11.5 |
Convalescent phase: 4.5 |
All patients received IVIG and aspirin | FMD | Yes | 7 |
Healthy control | 20 | 12/8 | 11.5 |
– | – | ||||||
Ishikawa et al. [41] | 2018 | Japan | KD | 25 | 12/13 | 7.0 |
Convalescent phase: 4.1 |
All patients received IVIG and aspirin | FMD, NMD | Yes | 9 |
Healthy control | 25 | 12/13 | 6.4 |
– | – | ||||||
Pi et al. [19] | 2018 | China | KD | 44 | 29/15 | 2.6 |
Acute phase: before treatment | All patients received IVIG and aspirin | P-selectin | Yes | 8 |
Subacute phase: 7–14 days after treatment | |||||||||||
Healthy control | 23 | 16/7 | 2.4 |
– | – | ||||||
Cetiner et al. [42] | 2021 | Turkey | KD | 26 | 21/5 | 8.2 |
Convalescent phase: 1–15 years | All patients received IVIG | FMD | No | 7 |
Healthy control | 26 | 22/4 | 9.0 |
– | – | ||||||
Routhu et al. [43] | 2021 | India | KD | 16 | 12/4 | 4.0 |
Acute phase: 1–15 days | All patients received IVIG and aspirin | FMD | No | 8 |
Convalescent phase: 3–12 months | |||||||||||
Healthy control | 16 | 10/6 | 5.1 |
– | – | ||||||
Wen et al. [44] | 2021 | China | KD | 105 | 62/43 | 2.9 |
Acute phase: before treatment | All patients received IVIG and aspirin | FMD | Yes | 9 |
Healthy control | 79 | 45/34 | 3.2 |
– | – | ||||||
CAL, coronary artery lesion; FMD, flow-mediated dilatation; ICAM-1, intercellular adhesion molecule-1; IVIG, intravenous immunoglobulin; KD, Kawasaki disease; NMD, nitroglycerin-mediated dilation; NOS, New castle Ottawa Scale; NR, no reported; VCAM-1, vascular cellular adhesion molecule-1. |
Five studies with 235 KD and 157 healthy children assessed FMD in the acute
phase [25, 34, 37, 43, 44]. During this phase, KD patients had lower FMD compared
to the control group (WMD = –10.39, 95% CI: –13.80– –6.98, p
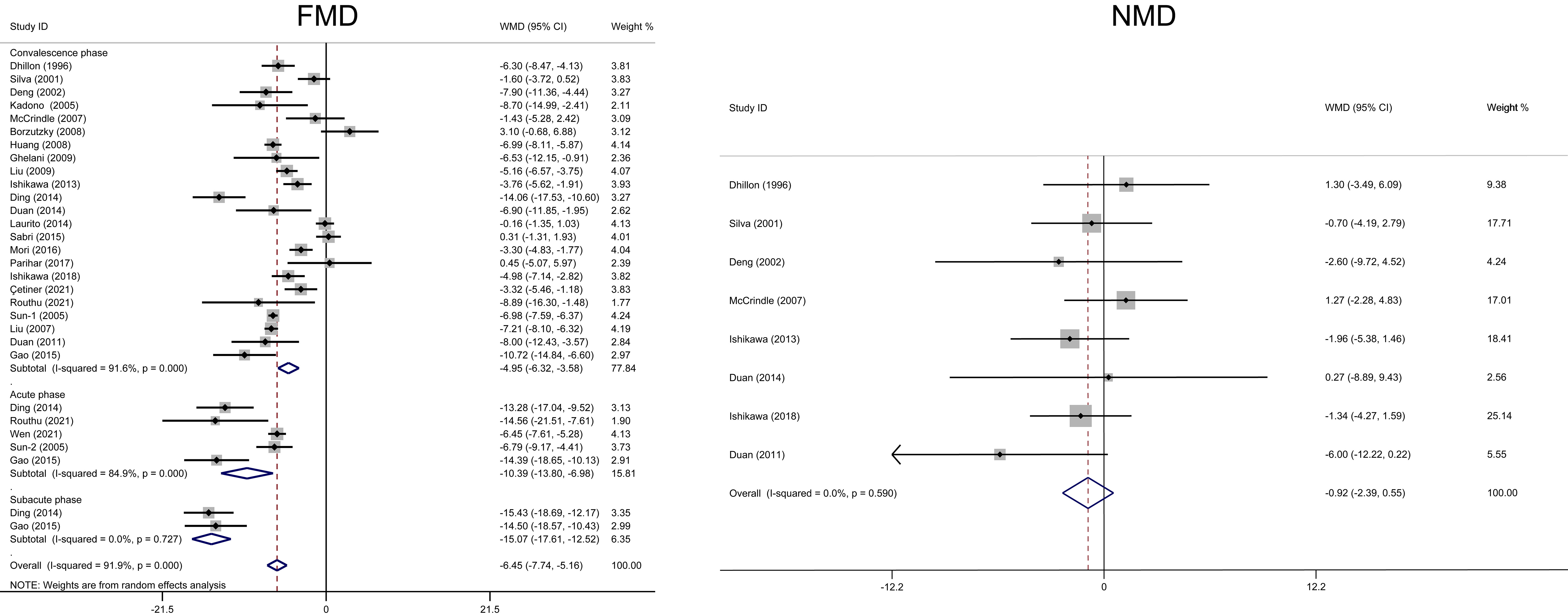
Forest plots of the meta-analysis of FMD/NMD between Kawasaki disease and healthy children during different phases. CI, confidence interval; FMD, flow-mediated dilatation; NMD, nitroglycerin-mediated dilation; WMD, weighted mean difference.
Number of studies | Test of difference | Test of heterogeneity | ||||
WMD (95% CI) | p value | I |
p value | |||
FMD (%) in the acute phase | ||||||
Language | ||||||
English | 3 | –10.89 (–16.72– –5.07) | 87.5 | |||
Chinese | 2 | –10.38 (–17.81– –2.94) | 0.006 | 89.3 | 0.002 | |
Country | ||||||
China | 4 | –9.76 (–13.29– –6.23) | 86.7 | |||
India | 1 | –14.56 (–21.51– –7.61) | - | - | ||
Occlusion position | ||||||
Foream | 2 | –9.96 (–17.45– –2.48) | 0.009 | 76.7 | 0.038 | |
Others | 3 | –11.12 (–16.92– –5.31) | 91 | |||
Occlusion’ pressure | ||||||
50 mmHg above resting SBP | 4 | –9.76 (–13.29– –6.23) | 86.7 | |||
250 mmHg | 1 | –14.56 (–21.51– –7.61) | - | - | ||
FMD (%) in the convalescence phase | ||||||
Language | ||||||
English | 19 | –4.28 (–5.88– –2.68) | 89.3 | |||
Chinese | 4 | –7.15 (–7.73– –6.57) | 9.6 | 0.345 | ||
Country | ||||||
Europe | 2 | –3.16 (–9.18–2.86) | 0.303 | 95.8 | ||
America | 3 | –0.22 (–3.04–2.61) | 0.881 | 57.6 | 0.095 | |
Asia | 18 | –5.92 (–7.22– –4.62) | 87.7 | |||
Follow-up duration | ||||||
15 | –4.67 (–6.00– –3.34) | 81.3 | ||||
1 | –8.89 (–16.30– –1.48) | 0.019 | - | - | ||
Mixed | 7 | –5.62 (–8.97– –2.27) | 0.001 | 96.7 | ||
Occlusion position | ||||||
Foream | 14 | –5.13 (–6.80– –3.46) | 93.2 | |||
Upper arm | 3 | –5.00 (–9.75– –0.26) | 0.039 | 83.7 | 0.002 | |
NR | 6 | –4.39 (–8.30– –0.47) | 0.028 | 90.2 | ||
Occlusion’ pressure | ||||||
50 mmHg above resting SBP | 7 | –5.57 (–8.93– –2.21) | 0.001 | 90.6 | ||
200 mmHg | 8 | –6.02 (–7.29– –4.75) | 59.1 | 0.017 | ||
Others | 8 | –3.20 (–5.65– –0.75) | 0.011 | 93 | ||
Occlusion duration | ||||||
5 mins | 20 | –5.18 (–6.71– –3.65) | 92.1 | |||
Others | 3 | –3.71 (–6.13– –1.28) | 0.003 | 79 | 0.009 | |
CI, confidence interval; FMD, flow-mediated dilatation; NR, no reported; SBP, systolic blood pressure; WMD, weighted mean difference. |
Only two studies with 75 KD and 47 healthy children assessed FMD in the subacute
phase [34, 37]. During this phase, KD patients had lower FMD compared to the
control group (WMD = –15.07, 95% CI: –17.61– –12.52, p
Twenty-three studies with 693 KD children and 584 healthy participants assessed
FMD in the convalescence phase. During this phase, KD patients
had lower FMD compared to the control group (WMD = –4.95, 95% CI: –6.32–
–3.58, p
Eight studies with 228 KD children and 190 healthy participants assessed NMD in
the convalescence phase. Sublingual nitroglycerin (glyceryl trinitrate) was
administrated before NMD measurement in all these eight studies. During this
phase, KD patients had similar NMD compared to the control group (WMD = –0.92,
95% CI: –2.39–0.55, p = 0.219, I
Only one study in the acute phase [44] and none in the subacute phase assessed the association of FMD measures with the severity of CAL.
During the convalescence phase, those KD patients without CAL, with CAL, even
with CAA, had progressive lower FMD compared to healthy children (WMD = –3.82,
95% CI: –7.30– –0.34; WMD = –6.32, 95% CI: –7.60– –5.04; and WMD =
–6.97, 95% CI: –7.99– –5.95, respectively; all p
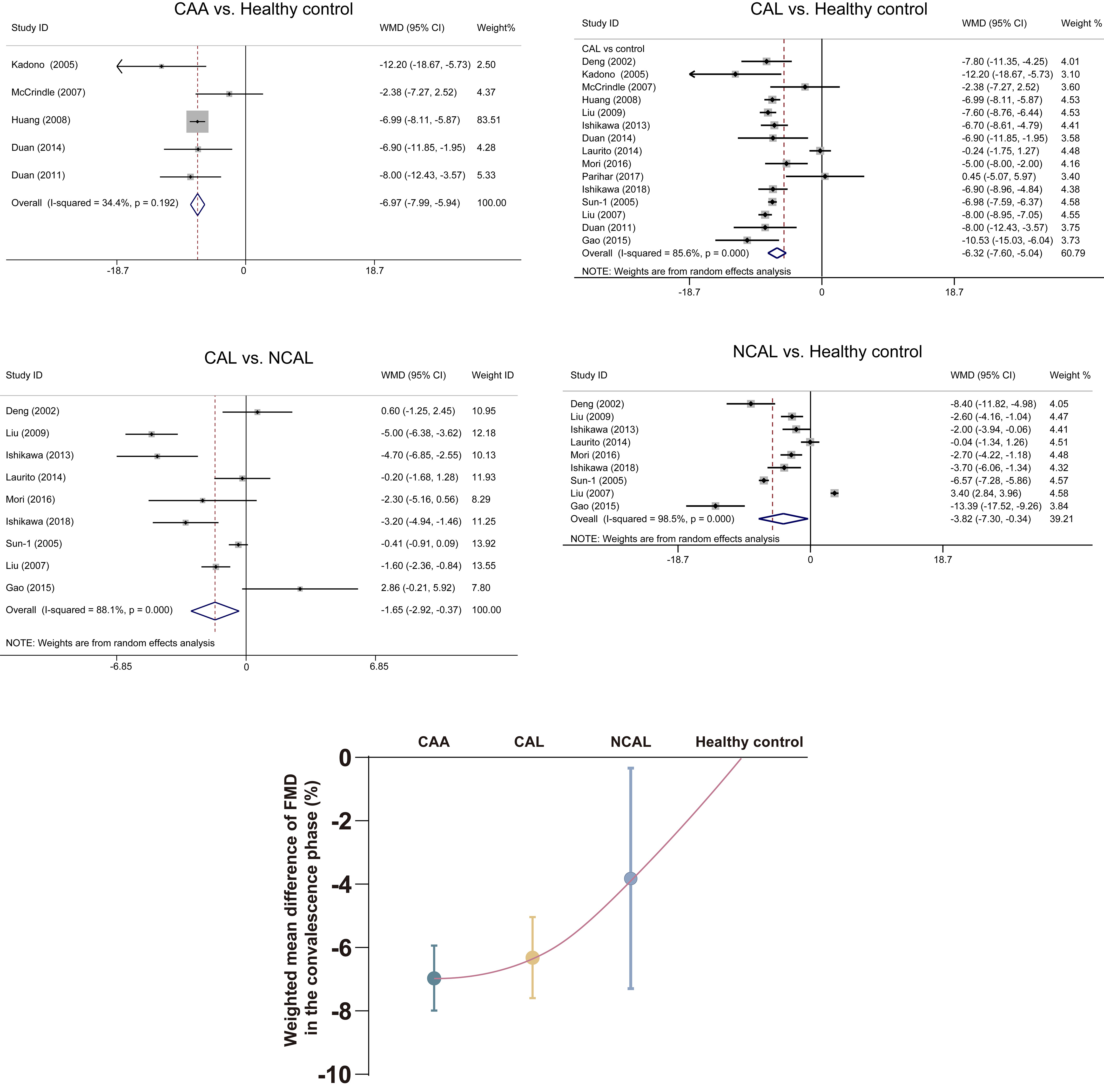
Association of FMD measures with severity of coronary artery in the convalescence phase. Data are expressed as mean with 95% CI. CAA, coronary artery aneurysm; CAL, coronary artery lesion; CI, confidence interval; FMD, flow-mediated dilatation; NCAL, no coronary artery lesion; WMD, weighted mean difference.
KD patients had higher levels of E-selectin, P-selectin, and ICAM-1 compared to the healthy control during different phases (Fig. 4). KD patients had a higher level of VCAM-1 compared to the healthy control only during the acute phase (WMD = 61.62, 95% CI: 21.38–101.86). There was no difference in VCAM-1 between KD and the healthy control group during the subacute and convalescence phases.
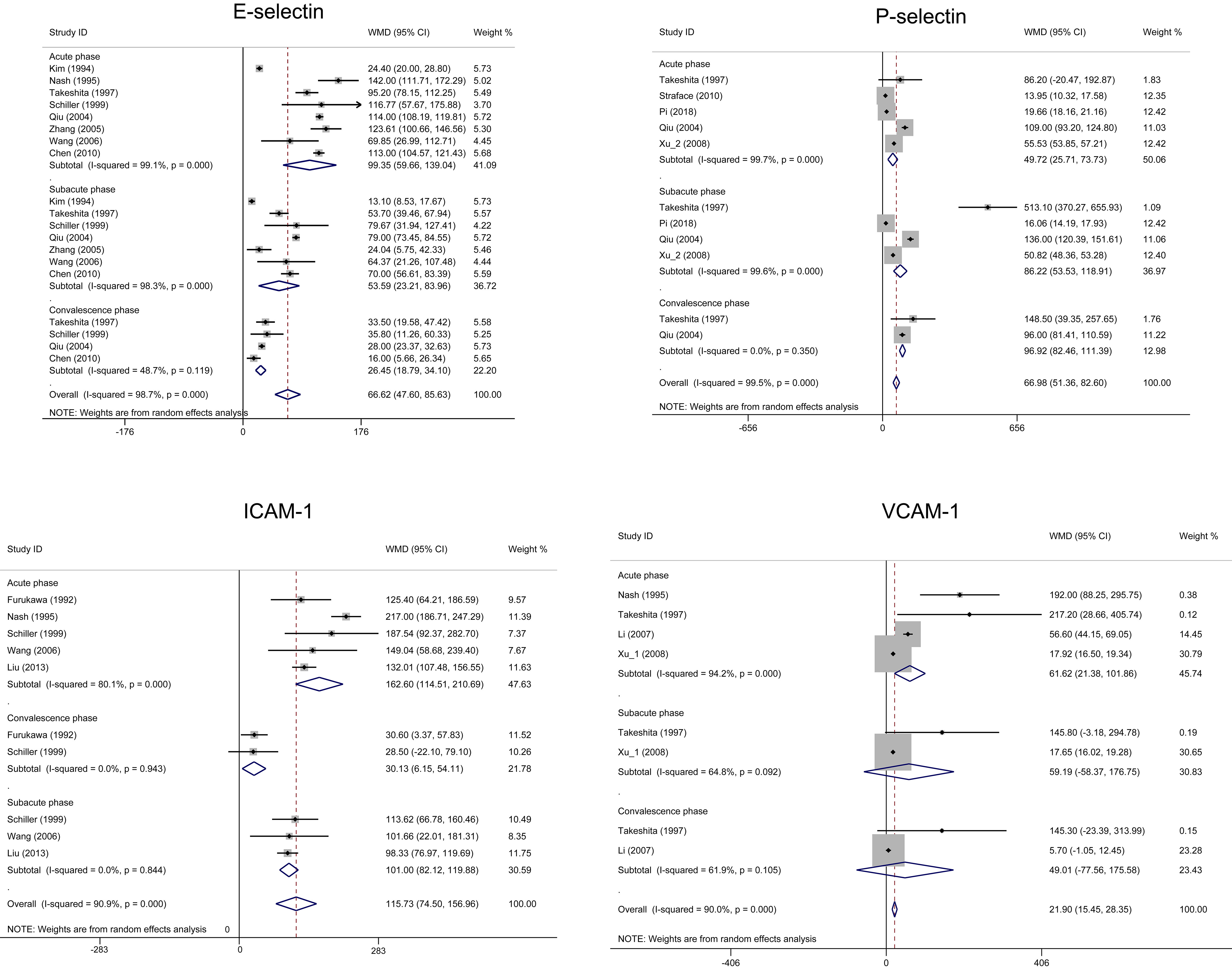
Forest plots of the meta-analysis of four biomarkers between Kawasaki disease and healthy children during different phases. CI, confidence interval; ICAM, intercellular adhesion molecule-1; VCAM, vascular cellular adhesion molecule-1; WMD, weighted mean difference.
To evaluate the robustness of the results, sensitivity analyses were performed by sequentially removing each study. No apparent change occurred for most outcomes when an individual study was omitted.
No publication bias was observed in our evaluation of the funnel plots for FMD (convalescence phase), NMD, and four biomarkers, confirmed by Begg’s and Egger’s tests (Supplementary Table 4, Supplementary Figs. 1–3). However, an obvious publication bias was revealed in our evaluation of the funnel plots for FMD (during acute phase), confirmed by Egger’s (p = 0.038) tests; Thus, the trim-and-fill method was used to adjust the publication bias. After trimming, the results were similar, indicating that the results were statistically reliable (WMD = 0.84, 95% CI: 0.66–1.02, Supplementary Fig. 4).
This is the first meta-analysis that comprehensively summarized the endothelial function alteration in KD children during different phases compared to healthy children (Fig. 5). The results were confirmed by subgroup analysis, sensitivity analysis, and publication bias test. Meanwhile, a positive correlation may exist between the degree of FMD impairment/endothelial dysfunction and the severity of CAL in KD patients during the convalescence phase.
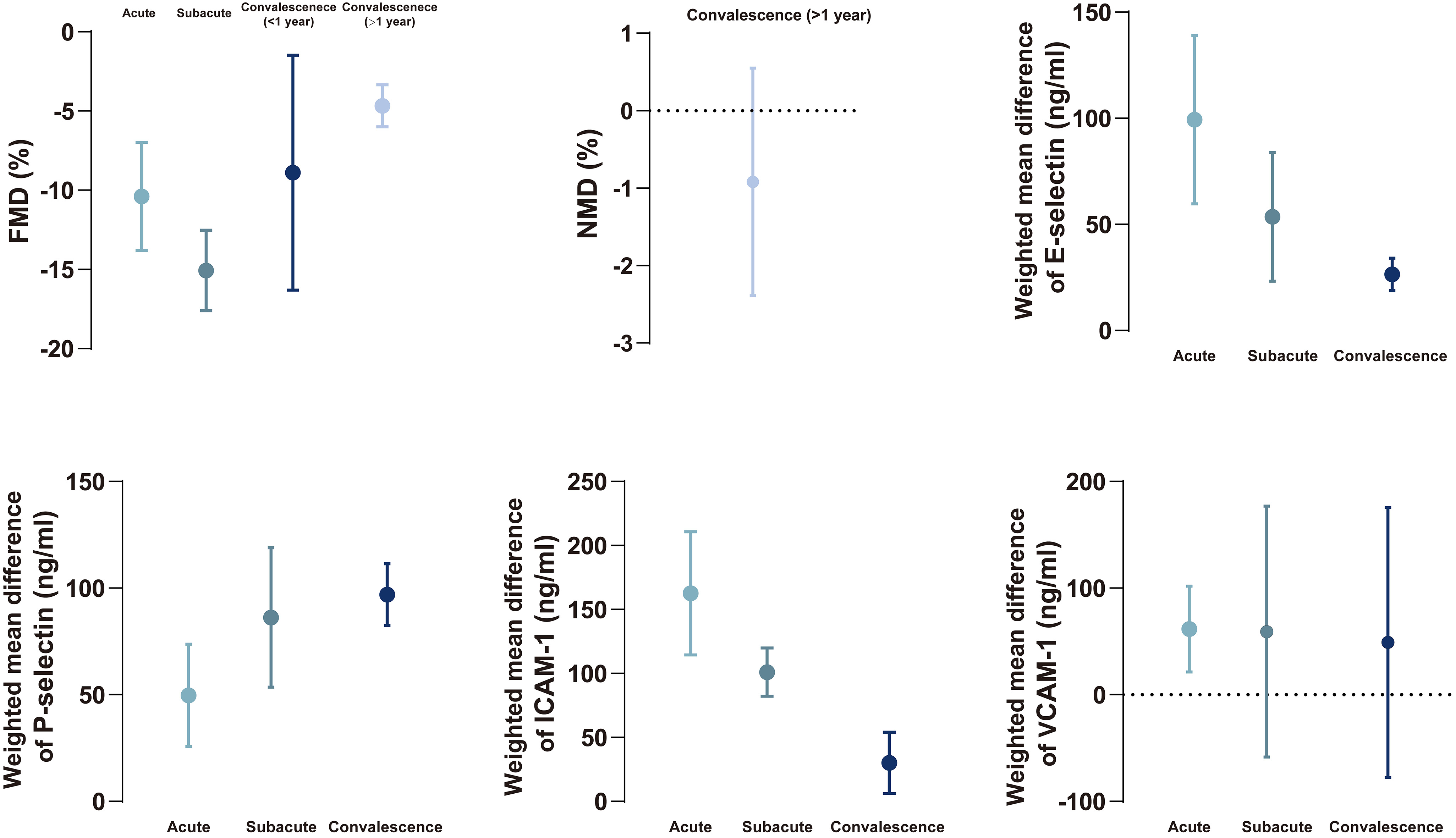
The summary of changing tendency in FMD, NMD, biomarkers between Kawasaki disease and healthy children during different phases. Data are expressed as mean with 95% confidence interval. FMD, flow-mediated dilatation; ICAM, intercellular adhesion molecule-1; NMD, nitroglycerin-mediated dilation; VCAM, vascular cellular adhesion molecule-1.
The etiology of KD is unknown. The most reasonable hypothesis is that activation of the immune system along with the release of inflammatory cytokines, destroys the intima of the artery. Histopathological findings in acute KD show widespread vascular inflammation with endothelial edema and necrosis and leukocyte infiltration, involving coronary and other medium-sized muscular arteries [51]. Endothelial dysfunction is one of the earliest manifestations of arteriosclerosis during vascular remodeling [52]. There were several noninvasive peripheral endothelial function tests, including FMD, peripheral arterial tonometry, laser-doppler flowmetry, laser-speckle contrast imaging/analysis, and near-infrared spectroscopy [53]. The most widely used test is FMD [54].
Endothelial dysfunction is characterized by vasodilatation impairment. FMD
measures changes in the endothelium-dependent vasodilator response after shear
stress and the dilation induced by the release of nitric oxide [55]. FMD provides
accurate prediction information for future cardiovascular events and is
considered the gold standard for assessing endothelial dysfunction [54]. Several
systemic reviews attempted to summarize FMD of KD during the convalescence phase
[56, 57, 58, 59, 60]. They all found that FMD decreased in patients with a history of KD.
However, none of those studies used subgroup analysis. Meanwhile, only Dietz and
her colleagues focused on the FMD of KD patients with CAL [56]. Due to CAL can be
separated into CAA group and “coronary artery dilation only” subgroup [1]. In
fact, they didn’t assess the relationship between FMD and CAA status (CAL
severity). In our results, FMD decreased in KD patients compared to the healthy
control, regardless of whether they are in the acute, subacute, or convalescent
phase. The degree of decreased FMD is phase dependent. The decrease of FMD in the
subacute phase is greater than in the acute phase, and FMD decrease is greater in
the subacute phase than in the convalescence phase. The reasons for this
difference are not clear but may relate in part to the pathophysical changes of
the arteries during different phases. In the subacute phase, luminal
myofibroblastic proliferation and laminar non-occlusive thrombosis may exist,
whereas in the acute phase, mild, transient dilatation may already occur [1], and
in the convalescent phase (in particular,
NMD, a part of the FMD protocol, is usually performed to assess endothelium-independent vasodilation and the function of vascular smooth muscle. A recent study revealed that NMD is an independent predictor of long-term cardiovascular events [61]. There was no difference in NMD between children with KD and healthy children, suggesting normal vascular smooth muscle function in patients with a history of KD and there is derangement in the vascular smooth muscle cells receptor pathway [62, 63].
E-selectin (CD62E), P-selectin (CD62P), ICAM-1 (CD54) and VCAM-1 (CD106) are cell surface adhesion molecules present on vascular endothelial cells [64, 65]. KD patients have elevated inflammatory cytokines, polyclonal B cell activation and T cell activation [66]. Those inflammatory cytokines upregulated these four biomarkers. Therefore, it has been proposed that cytokine-mediated vascular endothelial cell activation and injury is a central part of KD pathogenesis [45, 66]. In addition, oxidative stress is reported to impact vascular function by decreasing the availability of NO, leading to endothelial dysfunction [17, 41, 46]. In our results, elevated levels of E-selectin and ICAM-1 are phase dependent, with the highest levels in the acute phase and the lowest levels in the convalescent phase. The profiles of both biomarkers demonstrated that E-selectin and ICAM-1 could be used as reliable, early biomarkers for KD patients. In contrast, elevated level of P-selectin in the subacute phase is higher than that in the acute phase. The mechanism by which induced this tendence is still unknown but may be related in part to the source of release of p-selectin from both activated endothelium and activated platelets [67]. In the acute phase, the release of P-selectin may be predominately from early activated endothelial cells, whereas in the subacute phase, the release of P-selectin is derived from early activated endothelial cells, joining with the release of P-selectin by activated platelets. Therefore, P-selectin may be used as a sensitive biomarker for the subacute phase KD.
This correlation between FMD impairment degree and CAL severity needs more studies to confirm. Meanwhile, whether there is any difference in FMD between patients with persistent CAA and regressed CAA needs to be further researched. Second, decreased FMD was found in NCAL patients during the convalescence phase compared to the control group. FMD may be used as a good follow-up indicator for NCAL patients for future studies. Finally, the elevation of biomarkers of endothelial cells requires more evidence to support. The relationship between biomarkers levels and CAL severity in KD children was still unclear [68].
First, single race and effects of the involved studies can’t be ignored. The scarcity of studies with FMD during the subacute phase may affect the credibility of the conclusion. Meanwhile, only a limited numbers of NMD studies were involved in our analysis. Second, all included studies had a retrospective observational design, and the data were not sufficiently matched or adjusted for confounders. Confounding factors included severity of the disease, follow-up duration, treatment in the acute phase, lifestyle, dietary habits, etc. Third, high heterogeneity was found in most analyses. Subgroup analysis was performed. No source of heterogeneity was revealed. Fourth, the publication bias was existed in some analysis, which was adjusted by the trim-and-fill method. Five, the FMD/NMD protocol in each involved study is not exactly the same.
Endothelial dysfunction is present since the onset of KD and persists for years, confirmed by the measurement of FMD and biomarkers from different phases. An assumption is advanced that FMD impairment may be positively correlated with CAL severity during the convalescence phase.
CAA, coronary artery aneurysm; CAL, coronary artery lesion; CI, confidence interval; FMD, flow-mediated dilatation; ICAM, intercellular adhesion molecule-1; KD, Kawasaki disease; NMD, nitroglycerin-mediated dilation; VCAM, vascular cellular adhesion molecule-1; WMD, weighted mean difference.
XY and GS designed the research study. XY and DW performed the research. XY and DW analyzed the data. XY and GS wrote the manuscript. All authors contributed to editorial changes in the manuscript. All authors read and approved the final manuscript.
The study was approved by the Institutional Review Board (IRB) of the Shengjing Hospital of China Medical University (NO. 2022PS975K). The IRB waived the need for informed consent because this was a meta-analysis study based on published data.
We would like to express our gratitude to peer reviewers for their opinions and suggestions.
This research received no external funding.
The authors declare no conflict of interest.
Publisher’s Note: IMR Press stays neutral with regard to jurisdictional claims in published maps and institutional affiliations.