Academic Editors: Ichiro Wakabayashi and Klaus Groschner
Background: Hypertension is one of the most prevalent diseases in the
United States, affecting an estimated 3.5% of children and adolescents. It can be
adversely affect most organ systems but is particularly detrimental
to the heart and vascular systems. The repercussions can be gauged through well-established
measures of cardiovascular function including left ventricular mass index (LVMI), left
ventricular hypertrophy (LVH), carotid intima media thickness (cIMT), and aortic stiffness.
Cardiovascular function is also affected by underlying etiologies of hypertension
including chronic kidney disease, polycystic kidney disease, coarctation of the aorta, adrenal disorders, renal artery stenosis, obstructive sleep apnea,
as well as various drugs and medications (decongestants, stimulants, Non-steroidal Anti-inflammatory Drugs (NSAIDs), and
steroids). Methods: An exhaustive literature search was conducted for
clinical data regarding pediatric hypertension. Sixty-seven articles were incorporated
with data on 189,477 subjects total. The data was then extracted and categorized
as relating to hypertension incidence, LVMI, LVH, cIMT, and/or aortic stiffness.
Results: The prevalence of pediatric (
Hypertension (HTN) is one of the most prevalent diseases in the United States
and is estimated to affect 34% of adults as well as 3.5% of children and
adolescents [1, 2, 3]. According to the 2018 American Academy of Family Physicians
guidelines, standard systolic blood pressure (BP) is defined as 90–110 mmHg and
diastolic BP is 55–75 mmHg, whereas the mark for hypertension in children older
than 13 is
Hypertension can be categorized as either primary (essential) or secondary. Primary hypertension is defined as chronic elevation in blood BP without a specified cause. In 90–95% of cases, modifiable and nonmodifiable risk factors play a major role in its development. Risk factors in pediatric patients with primary hypertension include maternal smoking, family history of hypertension, sedentary lifestyle, overweight/obesity, poor diet, unmanaged stress, male sex, and low birth weight [1, 2, 3, 4]. Secondary hypertension, present in 5–10% of cases, is defined as an elevation in BP due to a specific cause or structural disorders such as chronic kidney disease, polycystic kidney disease, coarctation of the aorta, adrenal disorders, renal artery stenosis, obstructive sleep apnea, and as a result of a number of drugs and medication [5]. The World Heart Foundation has identified early diagnosis and treatment of high BP, especially in children and adolescents, vital to improving cardiovascular health and preventing long term morbidity and mortality [6]. Left untreated, sustained hypertension can result in earlier onset of CVD, kidney dysfunction and/or end organ damage. This literature review assesses current data on the incidence of pediatric and adolescent hypertension, associated cardiovascular parameters, as well as the effects of secondary hypertension on cardiovascular parameters.
A literature search utilizing CINAHL (1980–2021), Cochrane (1980–2021), Medline/PubMed (1986–2021), and Web of Science (1965–2021) was conducted. Keywords included “pediatrics” AND “hypertension”, “blood pressure”, “LVH”, “LVMI”, “cIMT”, “AASI”, “PWV”, and “PP”. No restriction on time or geographic location was used. The search was further narrowed to only include relevant studies in the English language. A total of 28,645 articles were found from the initial search.
Screening strategies are shown in the Patient/Population/Problem, Intervention/exposure, Comparison, and Outcome chart, or PICOS (Supplementary Table 1). The studies were included if primary data on a hypertensive pediatric cohort was reported. Animal studies, systematic reviews, and abstract-only literature that did not assess the significance hypertension in pediatric patients were excluded. After removing duplicate studies and excluding studies that did not meet the inclusion criteria, a total of 67 studies remained. Supplementary Fig. 1 details a flow chart with the inclusion and exclusion citations created following the Preferred Reporting Items for Systematic Reviews and Meta-Analyses (PRISMA) guidelines.
Data extraction was conducted by two independent individuals via full article examinations. If the studies were eligible, the sample sizes or individual cases of the patient populations were pooled together with respect to the hypertensive parameter category. From the included studies, the extracted data included number of subjects, age, sex, study design, study geography, and specified cardiovascular parameters. These results were compared with the reference ranges observed in the healthy subjects. All the analyses were performed using Microsoft Excel.
Our meta-analysis evaluates the incidence of pediatric and adolescent hypertension from 1994–2018 over 46 studies (n = 187,663) and has demonstrated a stable frequency of 4% (Range: 1–13%) (Table 1) [7, 8, 9, 10, 11, 12, 13, 14, 15, 16, 17, 18, 19, 20, 21, 22, 23, 24, 25, 26, 27, 28, 29, 30, 31, 32, 33, 34, 35, 36, 37, 38, 39, 40, 41, 42, 43, 44, 45, 46, 47, 48, 49, 50, 51, 52, 53]. Song et al.’s [54] systematic review over 47 articles (n = 94,675) demonstrated that the prevalence of childhood hypertension has increased from approximately 75% to 79% among children between ages 6 to 19 years from 2000 to 2015. This rapid increase is a cause for concern as children and adolescents with hypertension are prone to developing lifelong cardiovascular disease [49, 50, 51, 52, 53, 54].
Last name | Year | Sample sizes | Cases | Age range | Frequency |
Verma M, et al. [7] | 1994 | 2560 | 28 | 5–15 | 1% |
Adrogue HE, et al. [8] | 2001 | 14686 | 147 | 10–15 | 1% |
Sorof JM, et al. [9] | 2002 | 2460 | 70 | 12–16 | 3% |
Iman S, et al. [10] | 2003 | 2910 | 198 | 5–13 | 7% |
Rezende DF, et al. [11] | 2003 | 607 | 15 | 7–14 | 2% |
Sorof JM, et al. [12] | 2004 | 5102 | 221 | 10.3–19.4 | 4% |
Subhi MD, et al. [13] | 2006 | 1427 | 25 | 6–12 | 2% |
Ataei N, et al. [14] | 2007 | 6038 | 48 | 13–18 | 1% |
Chiolero A, et al. [15] | 2007 | 5207 | 191 | 10–14 | 4% |
McNiece KL, et al. [16] | 2007 | 6790 | 638 | 11–17 | 9% |
Ostrowska-NL, et al. [17] | 2007 | 25309 | 1240 | 7–18 | 5% |
Savitha MR, et al. [18] | 2007 | 503 | 31 | 10–16 | 6% |
Taksande A, et al. [19] | 2008 | 2643 | 152 | 6–17 | 6% |
Moore WE, et al. [20] | 2009 | 1829 | 42 | 5–17 | 2% |
Stergiou GS, et al. [21] | 2009 | 765 | 14 | 6.1–17.9 | 2% |
Katona E, et al. [22] | 2011 | 10213 | 258 | 15–18 | 3% |
Leung LC, et al. [23] | 2011 | 6093 | 88 | 6–18 | 1% |
Wang R, et al. [24] | 2011 | 1140 | 46 | 6–14 | 4% |
Steinthorsdottir SD, et al. [25] | 2011 | 970 | 30 | 4–14 | 3% |
Acosta AA, et al. [26] | 2012 | 1010 | 25 | 15.4 | 2% |
Rinaldi AEM, et al. [27] | 2012 | 903 | 29 | 6–14 | 3% |
Hong B, et al. [28] | 2012 | 4175 | 400 | 11–17 | 10% |
Kumar J, et al. [29] | 2012 | 990 | 34 | 10–19 | 3% |
Xu YJ, et al. & Zheng YS, et al. [30, 31] | 2012 | 2438 | 138 | 7–14 | 6% |
Cinteza E, et al. [32] | 2013 | 4866 | 358 | 3–17 | 7% |
Ujunwa FA, et al. [33] | 2013 | 2694 | 146 | 10–19 | 5% |
Basiratnia M, et al. [34] | 2013 | 2000 | 236 | 11–17 | 12% |
Meng L, et al. [35] | 2013 | 6304 | 197 | 3–18 | 3% |
Baradol RV, et al. [36] | 2014 | 2800 | 33 | 10–16 | 1% |
Outdili Z, et al. [37] | 2014 | 5207 | 113 | 10.1–14.9 | 2% |
Patil RR, et al. [38] | 2014 | 958 | 29 | 6–16 | 3% |
Bloetzer C, et al. [39] | 2015 | 5207 | 113 | 10–14 | 2% |
Menghetti E, et al. [40] | 2015 | 2007 | 124 | 6–17 | 6% |
Derezinski T, et al. [41] | 2015 | 416 | 51 | 14 | 12% |
Saury-Paredes LA, et al. [42] | 2016 | 259 | 16 | 5–11 | 6% |
Zhang X, et al. [43] | 2016 | 7781 | 465 | 6–18 | 6% |
Badeli H, et al. [44] | 2016 | 2072 | 144 | 7–17 | 7% |
de Oliveira L, et al. [45] | 2017 | 481 | 31 | 14–19 | 6% |
Okpokowuruk FS, et al. [46] | 2017 | 200 | 7 | 3–17 | 4% |
Ajayi IO, et al. [47] | 2017 | 1760 | 226 | 3–17 | 13% |
Bloetzer C, et al. [48] | 2017 | 5207 | 113 | 10–14 | 2% |
Cheung EL, et al. [49] | 2017 | 21062 | 569 | 10–19 | 3% |
Krzywinska-WM, et al. [50] | 2017 | 4941 | 435 | 10–18 | 9% |
Balsara SL, et al. [51] | 2018 | 2094 | 146 | 10–19 | 7% |
Deren K, et al. [52] | 2018 | 1024 | 17 | 12–17 | 2% |
Rodrigues PR, et al. [53] | 2018 | 1555 | 58 | 6–9 | 4% |
Total/Average | 187663 | 7735 | 4% |
Risk factors associated with hypertension can be divided into non-modifiable and modifiable. Nonmodifiable risk factors include age, family history, genetic predisposition, sex, race and ethnicity. BP increases with age and most adults develop hypertension by the age of 70 [55, 56, 57, 58, 59, 60]. Modifiable risk factors include obesity, diabetes mellitus, diet, physical activity, alcohol, and tobacco use, all known risks to children and adolescents. Poor dietary habits including high salt intake and low potassium intake are also correlated with elevated BP in children and adolescents [61, 62, 63].
The relationship between BP and CVD applies primarily to systolic BP, especially in children and adolescents [64, 65]. BP has proven to be a major risk factor for subsequent CVD development independent of other CVD risk factors [65]. Left unchecked, sustained hypertension can damage the cardiovascular system and kidneys, including left ventricular mass(LVM), carotid intima media thickness (cIMT), aortic arterial stiffness (AAS), pulse wave velocity (PWV), peripheral vascular disease (PVD). This review identifies these events/outcomes and analyzes their relationship with elevated BP as well as the correlation between hypertension and CVD.
Cardiac output is the product of stroke volume (SV) (volume of blood pumped by each heart beat) and heart beats per minute. SV is dependent on the force of contraction and resistance in the vascular system. BP is the product of the cardiac output and peripheral resistance. Consequently, inadequate volume regulation, enhanced vasoconstriction and changes in the arterial wall (including increased resistance and/or decreased luminal diameter) can contribute to hypertension [66, 67]. This balance in arterial tone is also affected by intravascular volume and neurohumoral systems. Maintenance of homeostatic BP levels involves a complex interplay of various elements of an integrated neurohumoral system such as the renin-angiotensin-aldosterone system (RAAS), natriuretic peptides, sympathetic nervous system (SNS) and the immune system [68].
An elevated cardiac output is more commonly seen in the pediatric population
while increased vascular resistance and vascular stiffness is more prevalent in
hypertensive adults [66, 67, 68, 69, 70, 71]. Increased vascular stiffness can be
attributed to increased
In contrast, high blood pressure in children is often due to an underlying secondary cause. Thus evaluation of pediatric hypertension is more comprehensive and includes comorbidities, risk factors, and evidence of target organ damage [72, 73]. Renal parenchymal abnormalities account for roughly 75% of secondary hypertension in children, followed by renovascular abnormalities. Therapeutic and illicit drugs (e.g., corticosteroids, decongestants) as well as stimulants like caffeine or attention deficit disorder medications can be iatrogenic contributors [73]. Less common causes include pediatric tumors (Wilms tumor, neuroblastoma, and pheochromocytoma), Williams syndrome, Turner syndrome, endocrinopathies (e.g., hypercortisolism, hyperaldosteronism, and diabetes), congenital adrenal hyperplasia, and systemic lupus erythematosus [72, 73].
Left ventricular (LV) mass is a meausure of weight of the LV and includes the
additive effect of BP on the heart. Increased blood pressure raises the afterload
and requires the cardiac tissue to produce more force. To compensate the heart
undergoes hypertrophy increasing in size and mass. Thus, primary hypertension
among children and adolescents is often associated with the sequela of LV hypertrophy
(LVH) and accordingly left ventricular mass index (LVMI) [74]. LVH is a
compensatory reaction which starts as physiologi-cal but may become pathological;
large increases in LVMI indicate target organ damage so it is often used as a surrogate
outcome for cardiovascular risk in children and adolescents [75, 76].
LVMI is calculated using echocardiography and/or cardiac MRI and is used to quantify
hypertension-induced LVH. An increased risk in cardiac mortality is associated
with an LVMI of
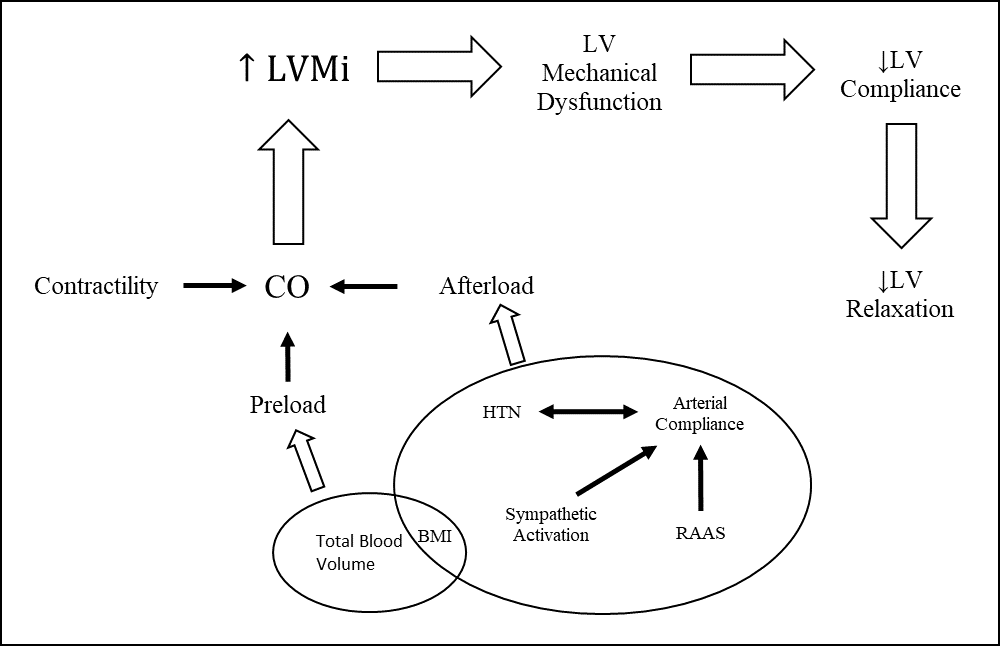
Mechanisms associated with development of LVH and Diastolic Dysfunction. Left ventricle remodeling due to hypertension leads to hypertrophy of cardiomyocytes with eventual fibrosis and diastolic dysfunction.
The strain of a hypertensive LV can be visualized using echocardiography by
measuring the relative wall thickness and mass. This can be compared to the wall
thickness and mass of a normotensive cohort. Our meta-analysis over seven studies
(n = 661) observed a statistically significant increase (p
Last name | Year | Age | Sample size | LVMI (g/m |
Control |
Mirchandani et al. [82] | 2014 | 8–18 | 109 | 42.3 | - |
Mir et al. [83] | 2016 | 35 | 32.9 | 28.8 | |
Meng et al. [84] | 2015 | 9–15 | 232 | 34 | 28 |
Lande et al. [85] | 2006 | 12–17 | 35 | 36 | - |
Sorof et al. [86] | 2003 | 11–16 | 32 | 46.8 | 31.4 |
Bjelakovic et al. [87] | 2015 | 10–16 | 94 | 46.6 | 38.2 |
Stabouli et al. [88] | 2009 | 5–18 | 124 | 36.8 | 29.5 |
Total/Average | 661 | 39.3 | 30.1 |
Several studies have shown LV diastolic dysfunction in children with primary
arterial hypertension can be measured via tissue Doppler imaging (TDI) [89].
Zamojska et al. [89] evaluated systolic and diastolic function in
children (n = 64) and found that the LV myocardial workload (based on
echocardiographic evaluation with the use of standard and tissue Doppler
echocardiographic parameters) was higher in children with hypertension (0.46
Stabouli et al. [88] assessed the LVM in normotensive, prehypertensive
and hypertensive children and adolescents (n = 124). They demonstrated that LVMI in the
prehypertensive cohort was significantly higher (29.5
The intima and media are the innermost layers of the artery and are prone to mechanical and physiologic dysfunction due to intravascular or extravascular forces. High BP can directly induce the carotid wall’s hypertrophy (intimal/medial thickening) as hemodynamic factors (e.g., local distending pressure, pulsatile load, and shear stress) induce intrinsic changes in the arterial walls. The carotid artery intima-media thickness is often evaluated in hypertensive individuals to gauge amassed endothelial deposits [90, 91, 92]. The presence or progression of endothelial deposition is more commonly referred to as atherosclerosis and its onset can begin as early in childhood or adolescence. Fatty streaks have been observed in the aorta, coronary and/or carotid arteries of patients as young as 2 years old [91].
Our meta-analysis of seven studies (n = 580) found a statistically significant
increase in cIMT in hypertensive children and adolescents
compared to normotensive controls (0.55 mm vs. 0.49 mm, p
Last name | Year | Age | Sample size | cIMT (mm) | Control |
Mir et al. [83] | 2016 | 35 | 0.46 | 0.35 | |
Meng et al. [84] | 2015 | 9–15 | 232 | 0.49 | 0.46 |
Litwin et al. [93] | 2004 | 6–20 | 110 | 0.45 | 0.41 |
Gil et al. [94] | 2008 | 13–18 | 32 | 0.62 | 0.5 |
Lande et al. [85] | 2006 | 12–17 | 35 | 0.67 | 0.63 |
Sorof et al. [86] | 2003 | 11–16 | 32 | 0.72 | 0.63 |
Antoniewicz et al. [95] | 2006 | 5–20 | 104 | 0.47 | 0.43 |
Total/Average | 580 | 0.55 | 0.49 |
Litwin et al. [93] demonstrated mechanical dysfunction in the arterial
wall (e.g., distensibility) in hypertensive children. The cross-sectional
compliance (change in volume to change in pressure) and
Arterial stiffness can result from arteriosclerosis and/or atheromatosis. Arteriosclerosis occurs when there is an increase in the rigidity and thickness of the arterial wall often leading to hypertension. Atheromatosis is a change in arterial inflammation, which leads to increased lipid deposition in the arterial walls and endothelial dysfunction [96]. The less compliant central vasculature changes hemodynamic flow and arterial pressure, thereby influencing cardiac function and coronary perfusion. Arterial stiffness plays a significant role in the development of CVD in the elderly population, and its role in the pediatric population is rapidly evolving, particularly in children with obesity [96]. The development of minimally invasive measurements of arterial stiffness, such as ambulatory arterial stiffness index (AASI), pulse wave velocity (PWV) and pulse pressure (PP), has been beneficial in diagnosing and predicting CVD.
AASI measures arterial stiffness via ambulatory BP monitoring (ABPM) during 24
hours of normal activity, providing prognostic information on cardiovascular
mortality and target organ damage [97, 98, 99]. Arterial stiffness has a nonlinear
relationship to distending pressure where an increase in mean arterial pressure
is associated with an exponential increase in arterial stiffness. In individuals
with elastic arteries, systolic and diastolic BPs vary according to changes in
mean arterial pressure such that systolic and diastolic BPs rise with activity
and fall during rest. However, individuals with stiff, inelastic vasculature are
less able to respond to changes in physiologic requirements [97]. Our
meta-analysis on pediatric AASI over 5 studies (n = 573) demonstrates elevated average
24-hour systolic BP and diastolic BP in hypertensive vs normotensive children at
129.56 mmHg vs. 99.73 mmHg and 73.69 mmHg vs. 69.81 mmHg, respectively (Table 4)
[21, 100, 101, 102, 103]. Simonetti et al. [100] assessed a hypertensive pediatric cohort (n =
114) and reported higher AASI values in hypertensive children compared
to normotensive participants (0.370
N | Years | 24-hour (mmHg) | |||||
Sample | Age | SBP | DBP | MAP | PP | ||
Stergiou et al. [21] | Normotensive | 66 | 12.8 | 115.10 | 64.70 | - | 50.40 |
Hypertensive | 16 | 14 | 135.60 | 74.30 | - | 61.30 | |
Simonetti et al. [100] | Normotensive | 71 | 12.1 | 109.50 | 66.40 | 80.70 | 43.40 |
Hypertensive | 114 | 12 | 125.50 | 77.90 | 93.70 | 47.60 | |
Kollios et al. [101] | Normotensive | 45 | 10.09 | 108.78 | 64.42 | 95.64 | - |
Hypertensive | 10 | 11.2 | 123.70 | 71.30 | 102.33 | - | |
Skrzypczyk et al. [102] | Normotensive | - | - | - | - | - | - |
Hypertensive | 177 | 15.04 | 128.17 | 70.84 | 89.95 | 57.53 | |
Skrzypczyk et al. [103] | Normotensive | 20 | 15.55 | 119.85 | 65.55 | 83.70 | 54.20 |
Hypertensive | 54 | 15.12 | 134.85 | 74.11 | 94.33 | 60.78 | |
Total/Average | Normotensive | 202 | 12.635 | 99.73 | 69.81 | 76.85 | 46.90 |
Hypertensive | 371 | 13.472 | 129.56 | 73.69 | 95.08 | 56.80 | |
Abbreviations: SBP, Systolic blood pressure; DBP, Diastolic blood pressure; MAP, Mean arterial pressure; PP, Pulse pressure. |
Univariate analyses between outcome and potential predictors | ||||||
Last Name; Year | Sample Size | Age | Outcome | Predictor | r | p-value |
Sorof et al. 2002 [9] | 37 | 13.5 |
LVMI | Ambulatory SBP index | 0.43 | 0.008 |
24-hour SBP | 0.34 | 0.037 | ||||
24-hour SBP load | 0.38 | 0.02 | ||||
Daytime SBP load | 0.37 | 0.025 | ||||
Nighttime SBP | 0.33 | 0.048 | ||||
Nightime SBP load | 0.38 | 0.021 | ||||
Sorof et al. 2003 [86] | 32 | 13.9 |
Weight | 0.42 | 0.02 | |
BMI | 0.49 | 0.005 | ||||
Urbina et al. 1995 [104] | 160 | 9–22 | SBP | 0.21–0.27 | 0.05 | |
Daniels et al. 1995 [105] | 201 | 6–17 | LVM | Age | 0.72 | - |
Height | 0.81 | - | ||||
Weight | 0.84 | - | ||||
Body Surface Area | 0.87 | - | ||||
Lean body mass | 0.86 | - | ||||
Fat Mass | 0.54 | - | ||||
SBP | 0.58 | - | ||||
DBP | 0.48 | - | ||||
Sorof et al. 2003 [86] | 32 | 13.9 |
Weight | 0.5 | 0.003 | |
BMI | 0.43 | 0.014 | ||||
LVMI | 0.54 | 0.001 | ||||
Interventricular septal thickness | 0.58 | 0.001 | ||||
Posterior wall thickness | 0.54 | 0.001 | ||||
Lande et al. 2006 [85] | 35 | 12–17 | cIMT | 24-h SBP load | 0.51 | 0.009 |
24-h DBP load | 0.5 | 0.01 | ||||
Daytime mean SBP | 0.43 | 0.003 | ||||
Nighttime DBP index | 0.4 | 0.04 | ||||
Daytime systolic load | 0.54 | 0.005 | ||||
Daytime diastolic load | 0.56 | 0.004 | ||||
Daytime diastolic index | 0.54 | 0.005 | ||||
Daytime mean diastolic index | 0.54 | 0.005 | ||||
Daytime mean DBP | 0.54 | 0.005 | ||||
Sorof et al. 2002 [9] | 37 | 13.5 |
Interventricular Septal Thickness | 24-hour SBP | 0.43 | 0.008 |
Wake SBP load | 0.44 | 0.007 | ||||
Sleep SBP | 0.39 | 0.017 | ||||
Left Ventricular Posterior Wall | 24-hour SBP | 0.41 | 0.012 | |||
Wake SBP load | 0.39 | 0.016 | ||||
Sleep SBP | 0.41 | 0.012 | ||||
Abbreviations: LVMI, Left ventricular mass index; LVM, Left ventricular mass; cIMT, carotid-intima media thickness. |
Pulse Wave Velocity (PWV) measures the speed at which pressure waves travel through blood vessels. Changes in the vessel’s ability to dilate, particularly as the arterial wall becomes stiffer, increase the rate at which the waves travel through the circulatory system. The increased rate of arterial pressure causes an increase in the rate of the reflected wave back to the right atrium. To compensate, ventricular systolic pressure increases [106]. An increase in the elastic modulus, vessel wall thickness and blood density with a decrease in vessel radius is associated with a higher PWV.
Due to variations in the segmental lengths at which pulse wave velocity can be
measured, direct comparisons amongst multiple pediatric/adolescent studies are
limited. Individual studies have shown statistically significant
differences in PWV between hypertensive and normotensive cohorts. Stergiou
et al. [21] observed an increased PWV (7.4
Pulse pressure (PP) is determined by the direct force of ventricular ejection and the viscoelasticity of arteries and can be calculated as SBP-DBP. It can also be calculated indirectly by wave reflections [107]. Wave reflections are formed when forward-moving blood is partially reflected back from arterial obstructions. Increased arterial stiffness causes the vessel’s wave reflections to become larger and arrive earlier in the systole, increasing PP [108]. Endothelial stress and arteriosclerosis increase PP as loss of vascular compliance increases SBP (while simultaneously decreasing DBP) [108]. Arteriosclerosis increases arterial stiffness, driving hypertension and increases in PP. Elevated PP has been associated with adverse cardiovascular outcomes and death, hence PP is a rough estimate to gauging arterial stiffness in relation to cardiovascular complications [109]. Overall, measures of aortic stiffness including AASI, PWV, and PP are all limited by the lack of pediatric references based on height, weight, age, and ethnicity. There is also a lack of standardization in AASI and PWV measurements and variation in the equipment used [107, 108, 109].
Our meta-analysis of PP over 5 studies (n = 573) compares the value
between hypertensive and normotensive children at 56.80 mmHg vs. 46.90 mmHg,
respectively (Table 4) [21, 102, 100, 101, 103]. Stergiou et al. [21] noted
positive associations in bivariate coefficients between PP and 24-hour AASI
(r = 0.50), LVM (r = 0.68). LVMI (r = 0.33), PWV
(r = 0.36), stroke volume (r = 0.36) and peripheral resistance
(r = 0.12). Simonetti et al. [100] also found consistent
increases between 24-hour PP and AASI (r
Secondary hypertension is common in pediatric patients with studies reporting a prevalence of 75–85% [110, 111, 112, 113]. The underlying causes typically vary with age; coarctation of the aorta and renal disorders are more common in children up to 6 years old whereas renal parenchymal disease is more likely to affect those between 6 to 10 years old [114]. Pediatric patients are typically referred to subspecialists for a comprehensive evaluation of secondary causes of hypertension, however, rising cases of obesity and essential hypertension are becoming more common. The 2020 update of the American Academy of Pediatrics (AAP) Recommendations for Preventative Pediatric Health Care suggests that young children with stage 1 or stage 2 hypertension as well as those with difficult to treat hypertension should be evaluated for secondary causes of hypertension [115]. More importantly, causes of secondary hypertension in children who are overweight, have stage 1 hypertension, or otherwise healthy children with new-onset hypertension should not be ruled out prematurely [116].
Chronic kidney disease (CKD) and hypertension are deeply interdependent pathophysiologic states as sustained hypertension can worsen kidney function, and, loss of kidney function can drive hypertension (Fig. 2). Sympathetic overactivity, RAAS dysregulation, salt retention and volume overload are all directly affected by and mediate both disorders [117] (Fig. 3).
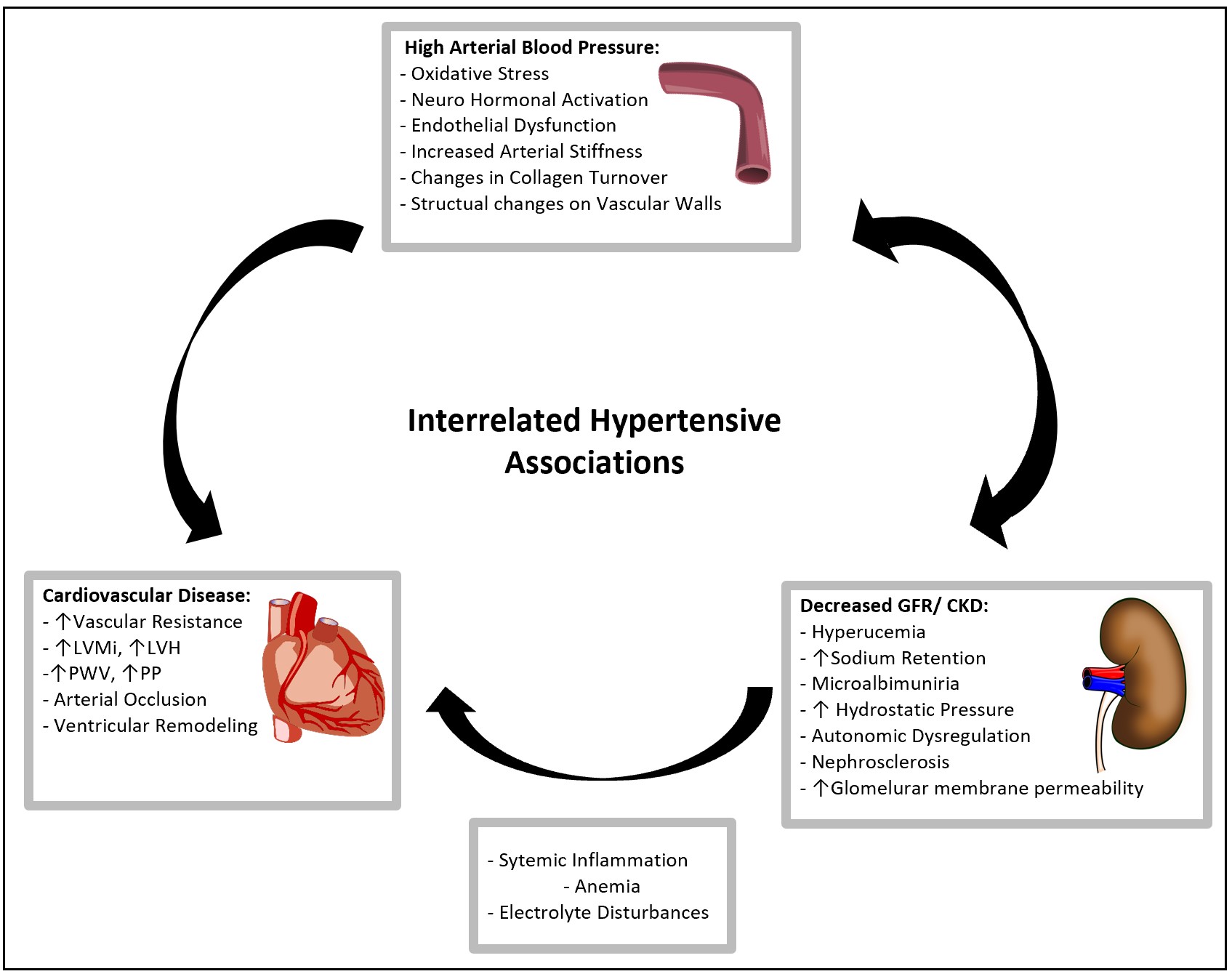
Interrelated Hypertensive Associations. A representation of the linked mechanisms of Hypertension, CKD, and CVD. Elevated blood pressure accelerates the interaction of endothelial dysfunction, oxidative stress, vascular atrophy and RAAS dysregulation which exacerbates the progression of cardiovascular disease.
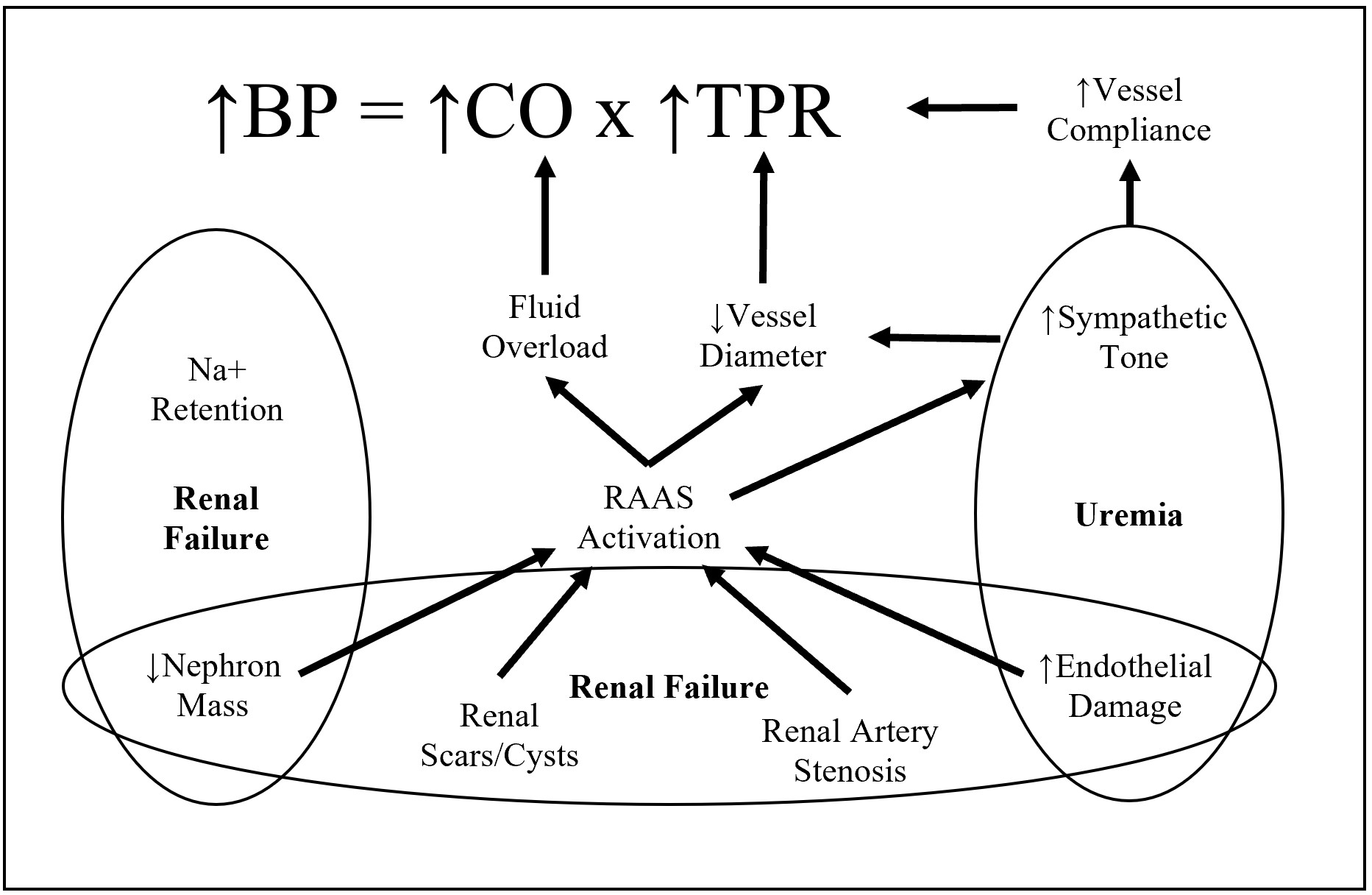
Factors associated in hypertension due to chronic kidney disease. A representation of the interdependent mechanisms of CKD and Hypertension. CKD, characterized by renal failure and uremia results in sympathetic overactivity of RAAS and eventual increase of cardiac output and total peripheral resistance.
CKD is associated with sympathetic overactivity, which stimulates RAAS, reducing blood flow in the peritubular capillaries downstream of sclerosed glomeruli. Glomeruli then increase renin secretion which increases circulating angiotensin II levels, driving increased systemic vascular resistance and BP [118]. Since there are fewer functioning glomeruli in CKD, the remaining glomeruli hyperfilter, increasing systemic arterial pressure and pulse pressure [118]. The overall net loss of GFR reduces sodium excretion rates, while angiotensin II also promotes sodium reabsorption in the proximal tubule and collecting duct. This consequently leads to an increase in extracellular volume, vasoconstriction and increased peripheral resistance, which the body attempts to counteract with release of the natriuretic hormone.
This association between progression of CKD and hypertension is emphasized by
Raina et al.’s [119] statistical analyses of a crosssectional pediatric
CKD cohort (n = 620). They demonstrated a significant positive correlation between
PP and LVMI (R
These associations are also supported by Reynolds et al. [120] who
demonstrated that non-glomerular CKD progression risk increases 3-fold and glomerular
CKD progression risk increases 2-fold if time-varying systolic BP supersedes the 90th
percentile in pediatric patients. Dionne et al. [121] analyzed longitudinal
ambulatory BP in a pediatric cohort (n = 679) with CKD via mean arterial pressure (MAP).
In children with non-glomerular CKD, MAP
Polycystic kidney disease is one of the most com-mon inherited kidney diseases [124]. It is caused by mu-tation in either PKD1 or PKD2 genes, of which PKD1 ac-counts for 85% of the cases [125].
Enlarging renal cysts can cause abdominal pain, hypertension, recurrent urinary tract infections (UTI) and renal stones. Common extrarenal manifestations include polycystic liver disease, in-tracranial aneurysms, valvular heart defects and abdominal hernias [126]. Patients with PKD generally develop symptoms in the adult age group [124], but this disease can manifest at any age [127]. Moreover, Very Early Onset (VEO) PKD (diagnosis in-utero or within 18 months of delivery) is associated with worse clinical outcomes such as development of early onset hypertension,CVD and ultimately End Stage Renal Disease (ESRD) [128].
Hypertension is a prominent feature of Autosomal dominant polycystic kidney disease (ADPKD) and is seen in the pediatric population. Marlais et al. [129] observed that the prevalence of hypertension in children with ADPKD is 20%, whereas, prevalence in the general is estimated to affect 3–5% pediatric population [130]. Various mechanisms have been proposed for the development of hypertension in ADPKD. In particular, the activation of RAAS by the enlarging of renal cysts is believed to play a major role [131]. Additionally, activation of RAAS and the favoring intrarenal ischaemia affects the sodium sensitivity and activity of the SNS which is known to augment over stimulation of the SNS in ADPKD patients and accelerate the onset of hypertension [132].
The most common cardiovascular outcome associated with PKD are
cardiac hypertrophy and cardiac remodeling [132]. An increase in the left
ventricular wall thickness is frequently detected in ADPKD patients and has a
significant correlation with borderline (blood pressure 75–95th percentile) and
high (
This data illustrates the increased prevalence of hypertension in pediatric patients with PKD and the increased risk of CVD in PKD patients. Furthermore, hypertension is common in these patients even before the onset of renal insufficiency. Effective hypertension treatment is a key factor determining patient morbidity and mortality, and early aggressive therapeutic intervention to mitigate the progression of CVD is paramount for improving long term outcomes.
Coarctation of the aorta is the fifth most common congenital heart defect with an incidence of 1 in 2500 live births [135]. It involves a narrowing of the aortic isthmus, often with subsequent tubular hypoplasia. The etiology is currently unknown and likely multifactorial; familial cases with associated gene deletions have been reported [136], but and evidence-based unifying theory has yet to be outlined.
Narrowing of the aorta in coarctation increases the pressure load on the proximal vessel in comparison to the aorta distal of the isthmus. The heightened mechanical stress induces rapid gene expression for collagen production, leading to remodeling of the vessel and increased resistance to pressure-induced dilatation [137]. Stiffening of the vessel contributes to an isolated systolic hypertension (with increased pulse pressure) similar to what is seen in vascular calcinosis. This hypertensive effect disseminates throughout the upper vasculature and commonly presents in infants as a drastic blood pressure difference between the upper and lower extremities.
Sezer et al. [135] investigated the effects of
stenting procedure on left ventricular function, aortic stiffness,
elasticity and systemic hypertension in children with coarctation of the aorta
and found that the enduring cardiovascular effects can not always be reverted.
The elasticity of the ascending aorta was found to be lower (6.4
There are several adrenal disorders which can cause hypertension in
pediatric populations. These disorders can be split into dysfunction or
hyperactivity of the adrenal cortex or the adrenal medulla. The prevalence of
adrenal medulla disorders ranges between 1:2500 and 1:6500, of which 20% is of
pediatric patients. The typical disorder of the adrenal medulla is
pheochromocytoma, paragangliomas, or secretions of norepinephrine,
epinephrine, dopamine and associated metabolites resulting in hypertension [140].
The disorders affecting the adrenal cortex and causing low renin hypertension
include 1
While norepinephrine and epinephrine induce hypertension by increasing
endothelial contraction, aldosterone is a mineralocorticoid hormone involved with
inflammation, vascular remodeling and oxidative stress resulting in organ damage,
and derangement of fluid or electrolyte balances. Aldosterone drives salt retention,
inflammation, and vasoconstriction as a protective response against dropping blood
pressures. However, unregulated levels of aldosterone can cause
endothelial damage by decreased nitric oxide synthase activity while increasing
superoxide anion generation. These free radicals, in combination with the
additional function of aldosterone retaining water/salt and inducing inflammation
through transcription of nuclear factor-
The systemic effects of aldosterone has been extensively studied in both adult
and pediatric populations. A study using cardiac MRI imaging of 35 patients with
primary hyperaldosteronism found decreased left ventricular ejection
fraction compared to non-hypertensive controls (59.0
Renovascular HTN is elevation in BP due to the narrowing of renal blood vessels. It is the most common cause of secondary HTN, responsible for 5–25% of HTN in children [145]. Renovascular hypertension results in activation of RAAS secondary to reduced blood flow to part or all of one or both kidneys [146, 147]. The underlying mechanism of the various derivations of renovascular hypertension revolves around decreased perfusion to the kidney and activation of the RAAS pathway. Prolonged ischemia increases the amount of renin expressing cells in the kidney which ultimately raises blood pressure via vasoconstriction in the heart and kidney, sympathetic nervous stimulation, aldosterone stimulation, and fi-broblast stimulation (thus thickening the vascular wall, myocardium, and fibrosis) [147, 148]. Renovascular HTN can result from multiple disorders pathologies including renal artery stenosis, fibromuscular dysplasia (FMD), arteritides such as Takayasu arteritis (TA) or mid aortic syndrome, extrinsic compression of a renal artery, and renal artery infarction [146, 147, 148, 149]. Renovascular hypertension prevelence is related to geographic location where FMD is more commonly found in North America and Europe while TA is more prevalent in Asia and South Africa [150, 151].
Renovascular hypertension should be suspected when blood pressure control is refractory to hypertensive medication or when an abdominal bruit is observed [150, 151].
Lu et al. [151] assessed 14 pediatric patients diagnosed with renovascular hypertension and observed a mean blood pressure of 187/127 mmHg at the time of diagnosis. Congestive heart failure was found in a subset of the cohort which highlights the importance of measuring blood pressure in pediatric clinical practice. Compared to adults where 70–80% of patients have largely non-correctable atherosclerotic lesions, children with renovascular hypertension typically have correctable lesions [152].
Obstructive sleep apnea (OSA) affects approximately 1–5% of children and is characterized by partial or complete obstruction of the upper airway and subsequent disruption in sleep and/or proper gas exchange [153]. The sequalae from OSA in children can include failure to thrive, enuresis, behavioral problems, poor scholarly performance, and cardiopulmonary disease [154]. The correlation between pediatric OSA and secondary hypertension has been well documented and the pathophysiology with the most current literature will be discussed subsequently [155, 156, 157, 158, 159].
The most common etiology of obstruction in children with OSA is adenotonsillar hypertrophy [154]. However, the role of obesity, upper airway inflammation, neurological hypotonia, and craniofacial anatomy is also significant. Regardless of the cause, repeated nighttime obstructions may lead to intermittent hypoxia, hypercapnia, and sleep fragmentation [158]. These factors further lead to the observable clinical signs and symptoms including, snoring, frequent awakenings, witnessed apneic episodes, enuresis and cognitive/behavioral problems. Pulmonary and systemic hypertension is of specific importance in this population due to its large potential for morbidity and mortality in the setting of untreated OSA, Data has demonstrated a dose-specific relationship between severity of OSA and potential for secondary hypertension [160, 161]. Furthermore, LVH and heart failure have been reported in children with severe OSA [157, 162].
Li et al. [157] studied 306 children aged 6–13 years and found significantly higher awake and nocturnal BP values in those with OSA, when compared to healthy children. The severity of OSA was directly correlated with worsening of BP values, with moderate to severe OSA patients having an increased risk for nocturnal systolic (OR 3.9, 95% CI 1.4–10.5) and nocturnal diastolic (OR 3.3, 95% CI 1.4–8.1) values [157]. The end organ manifestations of hypertension secondary to OSA often appear cardiac in nature. In 2019, Hanlon et al. [162] analyzed 61 obese or overweight children with OSA and found 71.7% of this population to had clinically apparent LVH on echocardiography. Children with OSA also had a significantly increased risk of LVH (85.7% vs. 59.4%, p = 0.047). After adjusting for age, sex, race, and BMI, OSA was still associated with 4.11 times increased odds of displaying LVH on echocardiogram (95% CI 1.15–14.65; p = 0.030) [162]. Multiple other studies also document the associations between OSA and ventricular pathology [156, 157, 163, 164, 165].
Few studies have evaluated the effect of OSA treatment on cardiovascular
parameters in children. Cincin et al. [165] studied myocardial
performance and anatomy before and after adenotonsillectomy in 30 patients with
diagnosed OSA. They demonstarted an improvement in both right ventricular
(RV) (0.515
NSAIDs are anti-inflammatory drugs with the potential of resulting in harmful side effects for the pediatric population [168]. Misurac et al. [169] reports a long-term study performed at their institution showing a 2.7% prevalence of NSAID induced AKI. The inhibition of prostaglandin production that occurs through NSAID use affects the kidney’s ability to modulate its GFR, especially when dehydrated. The resulting hypoperfusion of the glomerulus can result in medullary ischemic injury leading to acute renal failure. NSAIDs also have the propensity to increase sodium and water retention resulting in worsening of hypertension which could result in secondary complications such as heart failure and lower leg edema. Out of 1015 patients included in the study by Misurac et al. [169], 27 pediatric patients were diagnosed with NSAID-associated AKI. Further stratification of the data showed that 21 of those patients with NSAID-associated AKI had acute tubular necrosis either by clinical course or biopsy results. Additional research and randomized controlled trials are needed to full investigate the all-encompassing effects NSAID’s and stimulants in pediatric hypertension. With the high incidence of sinusitis in the pediatric population, the increased prescription of decongestants specifically targets the pediatric population [170]. The alpha-1 agonist activities of these decongestants can result in a dangerous side-effect of medication-induced hypertension which must be accounted for.
The importance of maintaining a healthy lifestyle is emphasized by the fact that confounding variables, most notable being obsese/overweight, may mask underlying causes of secondary hypertension. Obese and overweight body mass index (BMI) offen occur due to poor nutrition and lack of physical activity and have a significant influence on the onset of hypertension in pediatric patients and can augment cardiovascular implications [171].
The pathophysiology of hypertension in obesity and overweight children can be attributed to the hyperactivity of the sympathetic nervous system, and heart rate variability can serve an important non invasive marker. Heart rate variability can be categorized into low frequency (LF) indicating sympathovagal activity and high frequency (HF) indicating vagal activity which compose a normalized ratio called the sympathovagal balance [172]. A group of obese children and adolescents showed that the LF/HF ratio was significantly higher in obese children than controls which indicates heart sympathetic overactivity resulting in a higher systolic blood pressure [172]. Insulin resistance has also been implicated in the pathogenesis of secondary hypertension arising from obesity. Insulin resistance associated with obesity is known to inhibit glucose uptake but preserve the renal sodium retention effects causing a chronic volume overload and blood pressure elevation [171]. These mechanisms are associated with increased blood pressure and CVD including endothelial dysfunction, Left ventricular hypertrophy, and myocardial changes. Additionally, poor nutrition primarily characterized by excessive sodium intake can onset hypertension and cardiovascular outcomes. Sodium intake is more prevalent and detrimental to the progression of hypertension in obese children compared to healthy controls. Specifically, for every 1000 mg increase in sodium intake per day, the risk for elevated BP in obese children compared to normal weight children was 74% compared to 6%, respectively. Sodium intake is associated with increased risk of cardiovascular implications primarily CVD, stroke, and LVH [171, 173].
Obesity as a result of poor exercise and nutrition are detrimental risk factors
that have a significant influence on the progression of CVD and cardiovascular
implications. Berenson et al. [174] of the Bogalusa Heart Study observed
a significant association between increased BMI and systolic blood pressure with
fatty streaks and plaques in the coronary arteries (r = 0.60, p
Obesity and nutrition have significant effects on the progression of CVD. In addition, the prevalence and severity of obesity is drastically increasing in children. Encouraging fitness and quality nutrition in pediatric patients in addition to earlier screening for hypertension in patients with poor nutrition and fitness levels may be advantageous in managing the progression of CVD. Overall, current studies of long-term cardiovascular outcomes in children with hypertension are limited; however, there is growing evidence that childhood hypertension continues into and worsens throughout adulthood. In the Bogalusa Heart Study, children with increased BP were 2–3 times more likely to develop essential hypertension as young adults [177]. Similarly, Zhou et al. [178] found that in a 19.1 year follow up, untreated and uncontrolled hypertensive adults were at an increased risk for CVD (coronary artery disease, arrythmias, heart failure, heart valve dysfunction, heart attack, and stroke related mortalities) compared to patients who were normotensive or treated for hypertension. HTN not only affects coronary vessels but significantly affects cerebral and systemic vasculature. Thus, untreated hypertensive patients not only had a greater CVD specific mortality (HR = 1.77) compared to treated patients, but also had an increased cerebrovascular disease (cerebral vessel ischemia, stenosis, thrombosis, embolism, or hemorrhage) mortality (HR = 2.53) and all-cause mortality (hazard ratio = 1.40) [178]. The severity and progression of CVD, cerebrovascular disease, and all-cause mortality and morbidity due to untreated hypertension highlights the importance of maintaining BP homeostasis, especially in pediatric patients.
Sustained hypertension can lead to a number of adverse cardiovascular outcomes, all of which are accentuated in the pediatric population due to their longterm complications. Not only are functional parameters dysregulated in the cardiovascular system, but exponentiating target organ damage can also develop—especially in the renal system. Fortunately, these cardiovascular parameters including LVMI, LVH, cIMT, AASI, PWV, and PP provide noninvasive measures of early CVD. The well-established literature outlines the degree of correlation of each parameter and emphasizes its importance to cardiovascular health. Thus, prompt detection and intervention are imperative to prevent and control hypertension, especially in pediatric populations with often overlooked underlying conditions, to minimize the risk of longterm cardiovascular disease.
RR—designed the framework for this paper and actively revised all sections. AK, RS, NV, PK, BS, ND, KY, and MM—all equally contributed to this manuscript in developing a section. AN—proofread and edited this paper. KK and RR—revised the content for accuracy.
Not applicable.
We would like to express our gratitude to all those who helped us during the writing of this manuscript. Thanks to all the peer reviewers for their opinions and suggestions.
This research received no external funding.
The authors declare no conflict of interest.