†These authors contributed equally.
Academic Editor: Jerome L. Fleg
Background: To assess whether intraoperative monitoring and
intervention of regional cerebral oxygen saturation levels can reduce the
incidence of postoperative cognitive dysfunction in patients undergoing
cardiovascular surgery and contribute to patient prognosis. Methods: The
Cochrane Library, PubMed, and the Web of Science were systematically searched for
relevant randomized controlled trials involving the effects of cerebral oxygen
saturation on the cognitive function of patients after cardiovascular surgery
from January 1, 2000 to May 1, 2022. The primary outcome was the incidence of
postoperative cognitive dysfunction. The secondary outcomes were length of
hospital stay, length of intensive care unit (ICU) stay, length of mechanical ventilation, length of
cardiopulmonary bypass, and other major postoperative outcomes such as renal
failure, infection, arrhythmia, hospital mortality, and stroke. Data were pooled
using the risk ratio or standardized mean difference with 95% confidence
interval (CI). The original study protocol was registered prospectively with
PROSPERO (CRD42020178068). Results: A total of 13 randomized controlled
trials involving 1669 cardiovascular surgery patients were included. Compared
with the control group, the risk of postoperative cognitive dysfunction was
significantly lower in the intervention group (RR = 0.50; 95% CI: 0.30 to 0.85;
p = 0.01; I
Postoperative cognitive dysfunction (POCD) is a common postoperative
complication, manifested mainly as cognitive impairment, memory loss, and
executive dysfunction, which can lead to adverse reactions such as prolonged
hospital stay and decreased quality of life [1]. Several studies have shown that
POCD occurs after various operations, and the incidence of cardiovascular surgery
is higher than that of non-cardiac surgery [2]. In recent years, the incidence of
POCD in cardiovascular surgery patients has steadily increased, however, due to
the lack of understanding of POCD and its risk factors, there is currently no
effective prediction and treatment methods [3]. The incidence of POCD in cardiac
surgery can also be high, up to 70%, despite optimal oxygen levels [4].
Presently, decreased intraoperative cerebral oxygen saturation has been confirmed
to be related to postoperative neurological dysfunction and neurobehavioral
deterioration [5]. Therefore, regional cerebral oxygen saturation (rScO
Near-infrared spectroscopy (NIRS), a noninvasive method for monitoring cerebral
oxygen saturation in real-time, is widely used during perioperative procedures
[6]. In order to ensure sufficient cerebral perfusion and minimize the risk of
these neurocognitive complications, anesthesiologists use cerebral oxygen
saturation monitoring [7]. Although cerebral oxygen saturation monitoring has
been used as a clinical auxiliary technology for more than 20 years, there is a
lack of sufficient consensus on the management of cerebral oxygen saturation
during the perioperative period [8]. Some prospective randomized controlled
trials have sought to assess the effect of cerebral oxygen saturation on
postoperative cognitive outcomes. Nevertheless, the results remain inconclusive
[9]. In addition, some randomized controlled studies have confirmed the
predictive value of rScO
Studies have shown that in cardiac surgery patients, besides inflammation,
cardiac arrest or complicated by sepsis, the reduction of rScO
The study was conducted according to the Preferred Reporting Items for Systematic Reviews and Meta-Analyses Protocols (PRISMA) statement [15]. The protocol has been registered in the International Prospective Systematic Reviews Registry database (CRD42020178068).
The PubMed, Web of Science and Cochrane Library were searched from January 2000 to May 2022. The related searching words were as follows: [(Cognitive Decline) OR (Cognitive Dysfunction) OR (Cognitive Impairment) OR (Mental Deterioration) OR (Mild Cognitive Impairment) OR (Mild Neurocognitive Disorder)] AND [(Cardiovascular Disease) OR (myocardial infarct) OR (coronary heart disease) (myocardial infarct)] AND (regional cerebral oxygen saturation) in the title/abstract. Moreover, citations from articles were retrieved in order to identify relevant studies that were not included in the initial literature search. A search of clinicaltrials.gov was also conducted to identify any ongoing RCTs with results expected in the near future. The detailed search strategy is presented in the Supplementary Material in the form of a word document.
Inclusion criteria were pre-specified according to the PICOS approach (See the
Supplementary Table 1 for details). Inclusion criteria: (1) The
literature is a randomized controlled trial which must include the intervention
group that receives rScO
Data extraction was performed independently by 2 reviewers (WLC and LZK). Any disagreements were resolved by consensus or by consulting SXG. The data extraction content includes: first author, year of publication, sample size, research type, research object, intervention measures, control measures, primary and secondary outcomes, surgical method, cardiopulmonary bypass time, mechanical ventilation time, number of POCD cases, and major postoperative complications.
According to the “Randomized Trial Bias Risk Assessment Tool” in the Cochrane Handbook, the quality of the included literature is evaluated [16]. The evaluation content includes allocation hiding, randomization method, blinding method between investigator and subject, blinding method of result evaluator, and selective reporting of results, data completeness, and other possible biases in seven areas. The overall risk of bias judgement can be rated as low risk of bias, unclear risk of bias, and high risk of bias [16].
The primary outcome was the incidence of POCD. The secondary outcomes were length of postoperative hospital stay and intensive care unit (ICU) stay. Additional outcomes were cardiopulmonary bypass (CPB) time and mechanical ventilation time and major postoperative complications such as stroke, renal failure, infection, arrhythmia, and hospital mortality.
All data analyzed by Review Manager (RevMan) version 5.4 (The Cochrane Collaboration, Copenhagen, Denmark) and Stata SE 16.0 (Stata Corporation, College Station, TX, USA). The risk ratio (RR) with 95% confidence intervals (CI) were
estimated for dichotomous data, and standard mean difference (SMD) with 95% CI
for continuous data, respectively. In order to take into account methodological
and clinical heterogeneity, the fixed and random effect models were employed to
assemble the data [16]. The Q-test and I
A total of 2603 articles were retrieved from the database, and after deduplication, 1862 article titles and abstracts were evaluated, and then 34 articles were read in full to determine whether they met the criteria for inclusion in the study. Among them, 21 articles were excluded. 9 articles were omitted because they were not randomized controlled trials. 5 articles were excluded because of a lack of relevant outcome indicators. 6 articles were eliminated because cerebral oxygen saturation was not monitored and intervened and 1 article was removed because it’s not cardiovascular surgery (Fig. 1). Thirteen trials were finally included [5, 11, 17, 18, 19, 20, 21, 22, 23, 24, 25, 26, 27].
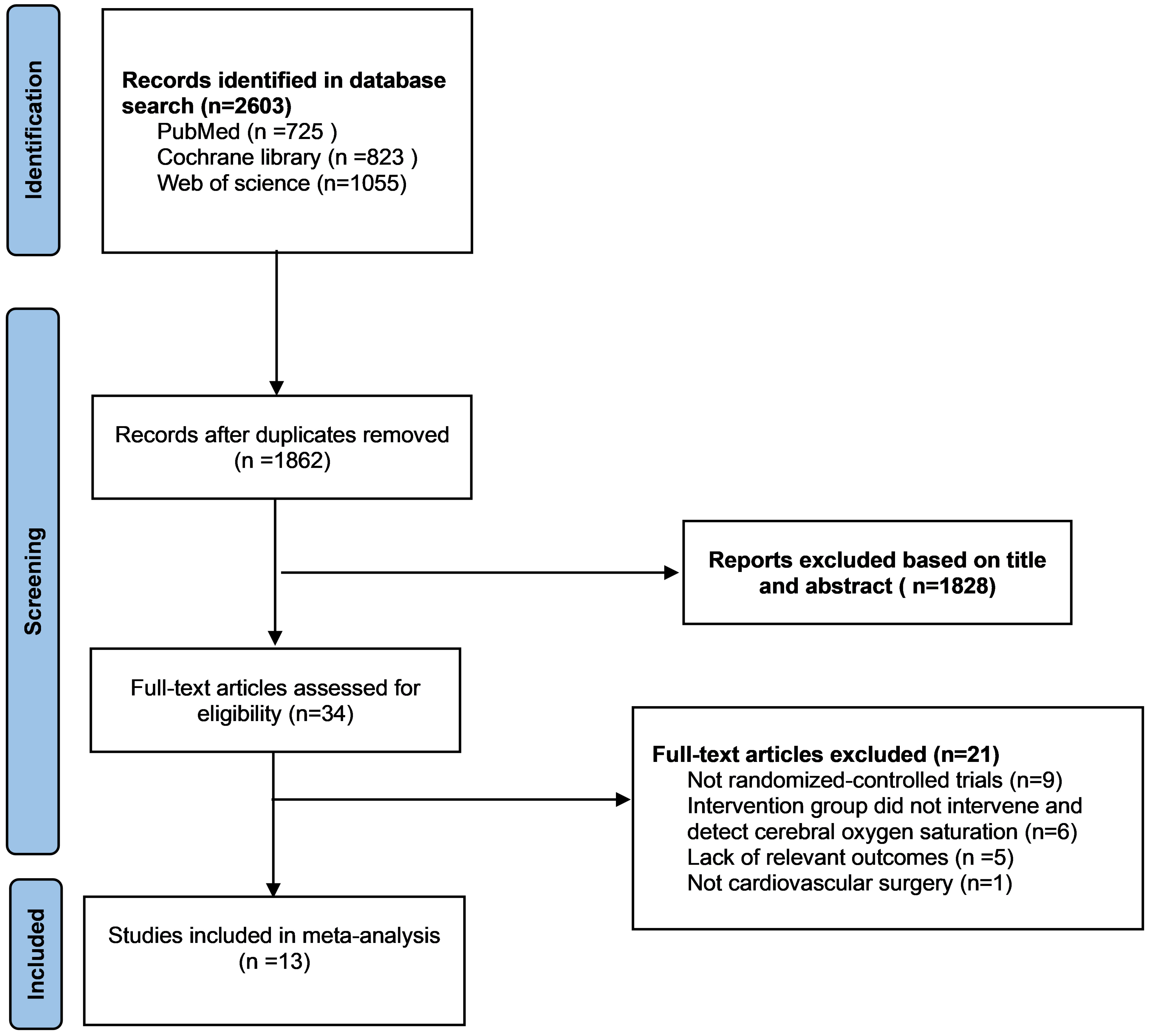
Preferred reporting items for systematic reviews and meta-analyses (PRISMA) flowchart of selection.
The basic characteristics of included studies were summarized in Table 1 (Ref. [5, 11, 14, 17, 18, 19, 20, 21, 22, 23, 25, 26, 27]), these trials were reported from 2007 to 2020. Sample sizes for individual experiments ranged from 10 to 249. Table 2 (Ref. [5, 11, 14, 17, 18, 19, 20, 21, 22, 23, 25, 26, 27]) presents the basic information about the patient population included in the study. A total of 1669 patients were involved, and the overall mean age of the patients included in the study was 64.7 years. All patients underwent cardiovascular-related surgery. An overview of the risk of bias assessment for each included trial can be found in Figs. 2,3. As a whole, three trials were classified as having a low risk of bias [5, 19, 20], seven as having an unclear risk of bias [17, 18, 21, 22, 24, 25, 26], and three as having a high risk of bias [11, 23, 27].
References | Country | Sample size | Age | Surgery | Outcome | ||
Intervention | Control | Intervention | Control | ||||
Colak 2015 [5] | Croatia | 94 | 96 | 61.9 |
63.4 |
CABG | POCD incidence, stroke, RF, infection, arrhythmia, MVD, CPB time |
Deschamps 2013 [17] | Canada | 23 | 25 | 71.1 |
70.2 |
High-risk cardiac surgery | hospital stay, MVD, CPB time |
Deschamps 2016 [18] | Canada | 102 | 99 | 69 |
72 |
Cardiac surgery | POCD incidence, stroke, ICU time, RF, infection, arrhythmia, death, hospital stay, MVD, CPB time |
Kara 2015 [19] | Turkey | 43 | 36 | 59.1 |
61.2 |
CABG | POCD incidence, ICU time, hospital stay, CPB time |
Kunst 2020 [20] | England | 42 | 40 | 71.6 |
72.0 |
CABG | POCD incidence, ICU time, RF, infection, arrhythmia, death, hospital stay, CPB time |
Lei 2017 [21] | America | 123 | 126 | 74.2 |
72.9 |
Cardiac surgery | POCD incidence, stroke, ICU time, RF, infection, arrhythmia, death, hospital stay, MVD |
Mohandas 2013 [11] | India | 50 | 50 | 34.60 |
38.05 |
Open heart surgery | POCD incidence, ICU time, MVD, CPB time |
Molstrom 2017 [22] | Denmark | 5 | 5 | 69 [66–72] | 72 [72–77] | Cardiac surgery | hospital stay, CPB time |
Murkin 2007 [23] | Canada | 100 | 100 | 61.8 |
61.8 |
CABG | Stroke, ICU time, RF, infection, arrhythmia, death, hospital stay, MVD, CPB time |
Uysal 2019 [14] | America | 59 | 66 | 57 |
58 |
Cardiac surgery | POCD incidence, ICU time, RF, death, hospital stay, MVD, CPB time |
Vretzakis 2013 [25] | America | 75 | 75 | 67.3 |
65.9 |
Cardiac surgery | ICU time, RF, arrhythmia, death, hospital stay, MVD, CPB time |
Zhu 2020 [26] | China | 33 | 33 | 61.52 |
60.27 |
CVR | POCD incidence, CPB time |
Zogogiannis 2011 [27] | Greece | 83 | 86 | 69.1 (50–82) | 68.4 (48–81) | Carotid endarterectomy | POCD incidence, stroke, death |
POCD, postoperative cognitive dysfunction; CABG, coronary artery bypass graft;
RF, renal failure; MVD, mechanical ventilation duration; CVR, cardiac valve
replacement; ICU, intensive care unit; CPB, Cardiopulmonary bypass. Data are expressed as mean |
Reference | Age (year) | Male (%) | BMI | Pre-MI (%) | DM (%) | HT (%) | CVA (%) | COPD (%) | CPB duration (min) | Euroscore | Baseline LVEF (%) |
Colak 2015 [5] | 62.7 | 78.0 | NA | 11.6 | 33.7 | 90.5 | NA | NA | 90.0 | 2.3 | 56 |
Deschamps 2013 [17] | 70.6 | 68.8 | NA | NA | NA | NA | NA | NA | 116.6 | NA | 56.3 |
Deschamps 2016 [18] | 71.0 | 72.1 | NA | 4.5 | 29.4 | 79.6 | NA | 9.9 | 135.9 | 5.3 | NA |
Kara 2015 [19] | 60.1 | 78.5 | NA | NA | 30.4 | 74.7 | 12.7 | 15.2 | 78.1 | NA | 54.0 |
Kunst 2020 [20] | 71.8 | 81.7 | 26.7 | NA | 31.7 | 92.7 | 14.6 | 11.0 | 81.1 | 4.4 | NA |
Lei 2017 [21] | 73.5 | 70.7 | 28.1 | 12.4 | 27.7 | 76.7 | 14.5 | 12.9 | 111.0 | NA | NA |
Mohandas 2013 [11] | 36.3 | 58.0 | 20.7 | NA | NA | NA | NA | NA | 88.7 | NA | NA |
Molstrom 2017 [22] | 71.5 | 90.0 | 25.8 | NA | NA | NA | NA | NA | 99.6 | NA | NA |
Murkin 2007 [23] | 61.8 | 87.5 | 29.6 | 5 | 28.5 | NA | 17.5 | 17.5 | 88.2 | NA | NA |
Uysal 2019 [14] | 57.5 | 68.8 | 26.8 | NA | NA | NA | NA | NA | 131.1 | 2.5 | 60.0 |
Vretzakis 2013 [25] | 66.6 | 82.0 | 27.8 | 54.7 | 24 | 80.6 | 21.3 | 21.3 | 91.3 | NA | 47.8 |
Zhu 2020 [26] | 60.9 | NA | NA | NA | NA | NA | NA | NA | 105.3 | NA | NA |
Zogogiannis 2011 [27] | 68.7 | 69.2 | 28.0 | NA | 26.6 | 53.8 | NA | NA | NA | NA | NA |
BMI, body mass index; MI, myocardial infarction; DM, diabetes mellitus; HT, hypertension; CVA, cerebrovascular accident; COPD, chronic obstructive pulmonary disease. |
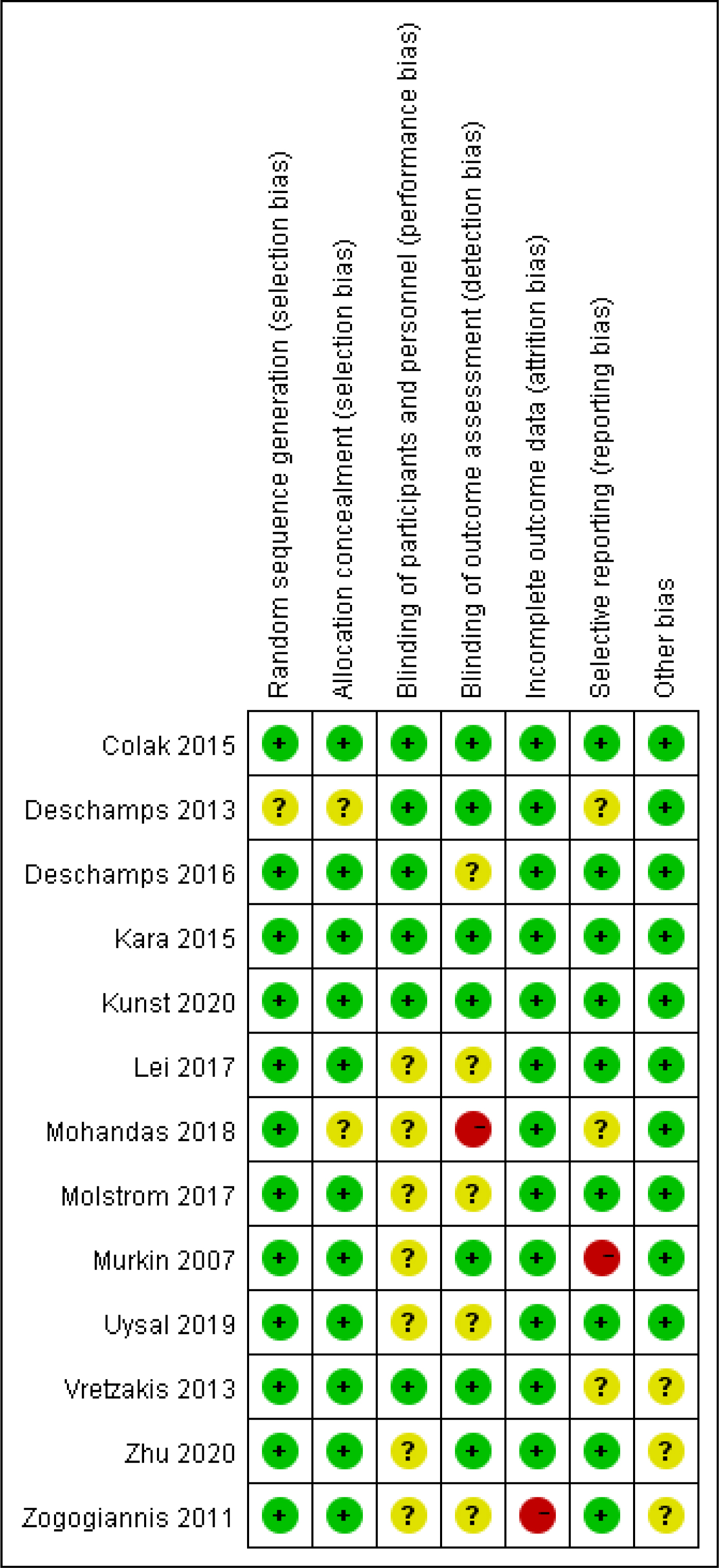
Risk of bias summary: review authors’ judgements about each risk of bias item for each included study.
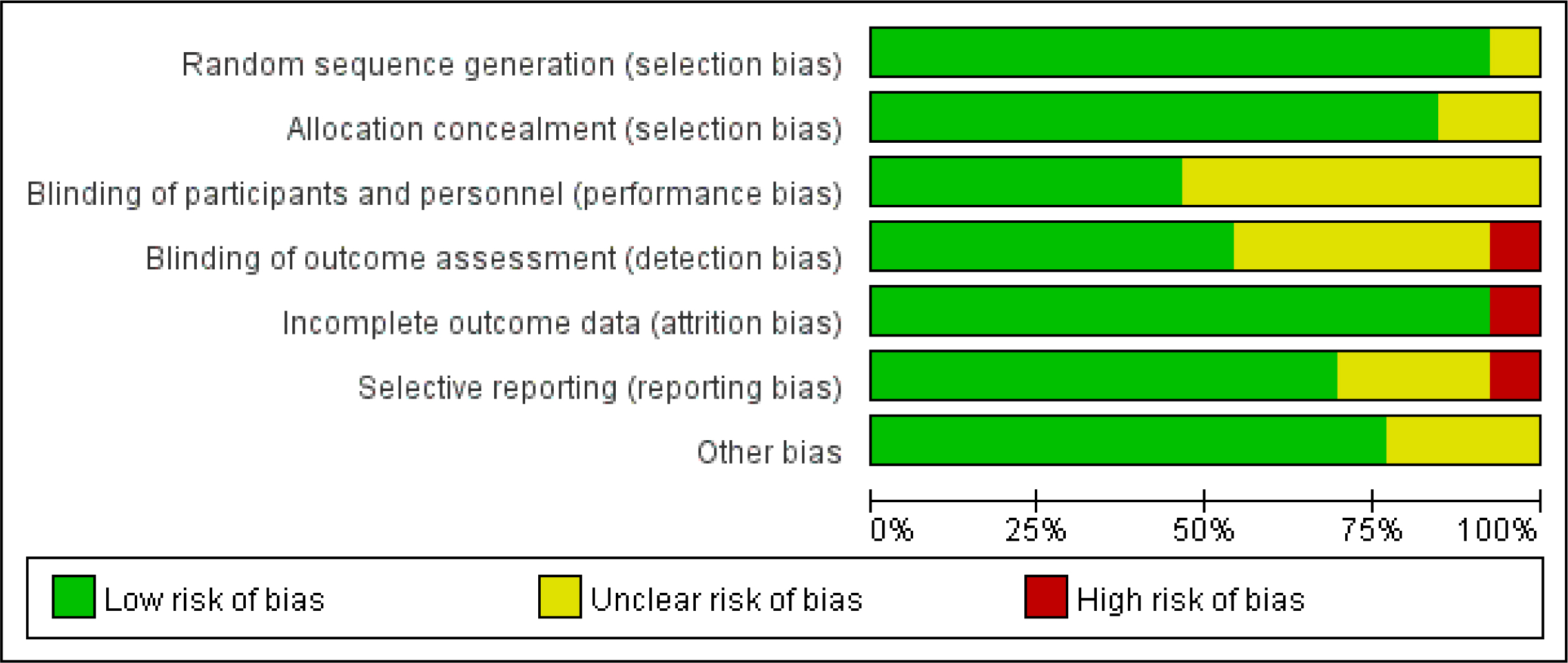
Risk of bias graph: review authors’ judgements about each risk of bias item presented as percentages across all included studies.
The incidence of POCD in a total of 912 patients in 8 RCTs was analyzed. The
risk of POCD in the intervention group was significantly lower than that in the
control group (Fig. 4A; RR, 0.50; 95% CI: 0.30 to 0.85; p = 0.01;
I
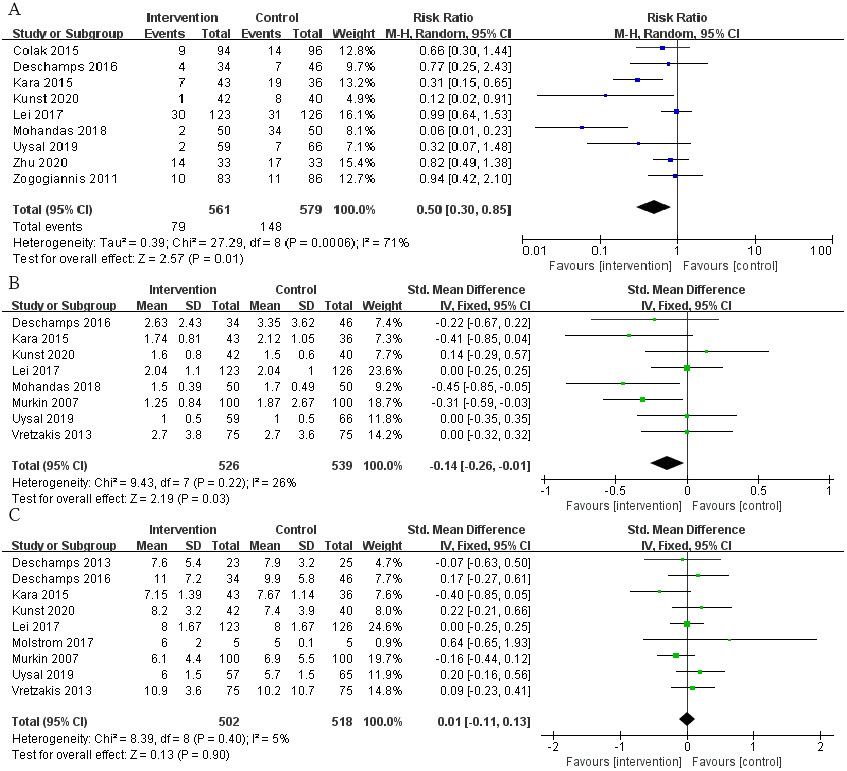
The pooled effect of (A) postoperative cognitive dysfunction (POCD) incidence. (B)Intensive care unit (ICU) time. (C) Hospital stay.
A total of 8 RCTs reported patients’ ICU time, involving a total of 1065
patients. The time of ICU stay in the intervention group was shorter, and the
difference was statistically significant (Fig. 4B; SMD, –0.14 days; 95% CI:
–0.26 to –0.01; p = 0.03; I
We also selected some routine postoperative complications as secondary outcomes
to evaluate the prognosis of patients, but there were no remarkable differences
regarding the incidence of renal failure (Fig. 5A; RR, 1.10; 95% CI: 0.67 to
1.79; p = 0.71; I
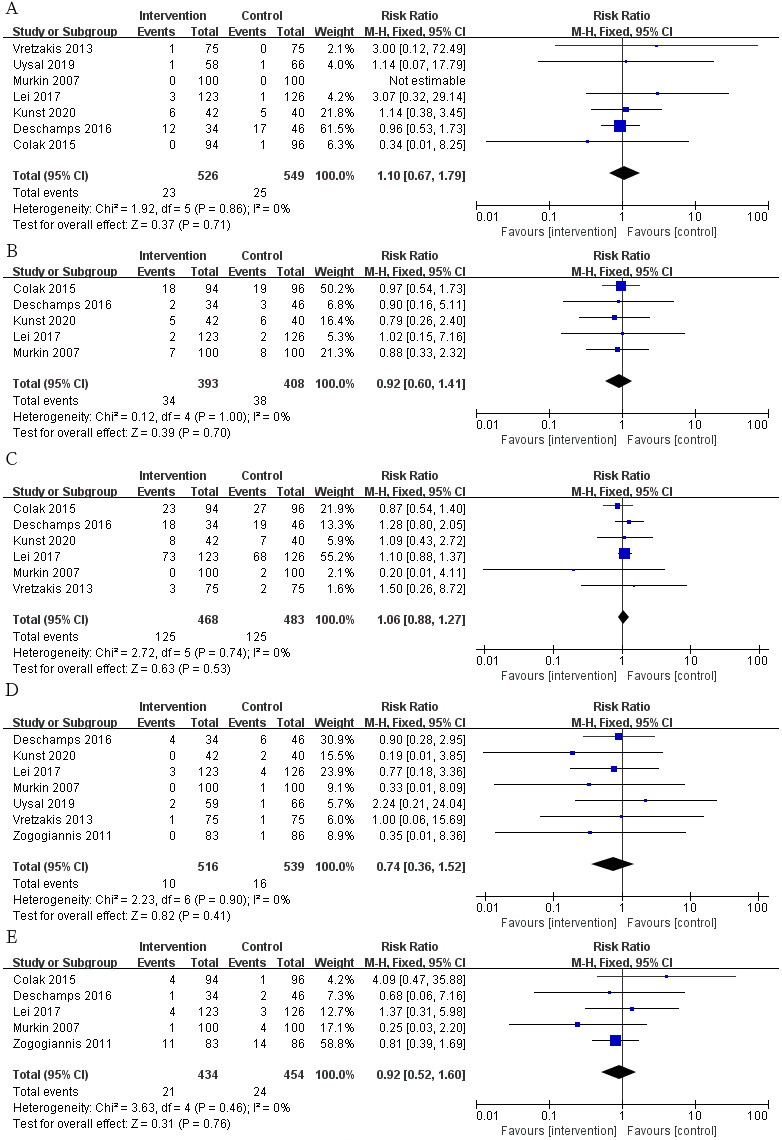
The pooled effect of (A) renal failure. (B) Infection. (C) Arrhythmia. (D) Hospital mortality. (E) Stroke.
Compared with the control group, the mechanical ventilation duration (Fig. 6A;
SMD, –0.03; 95% CI: –0.15 to 0.09; p = 0.63; I
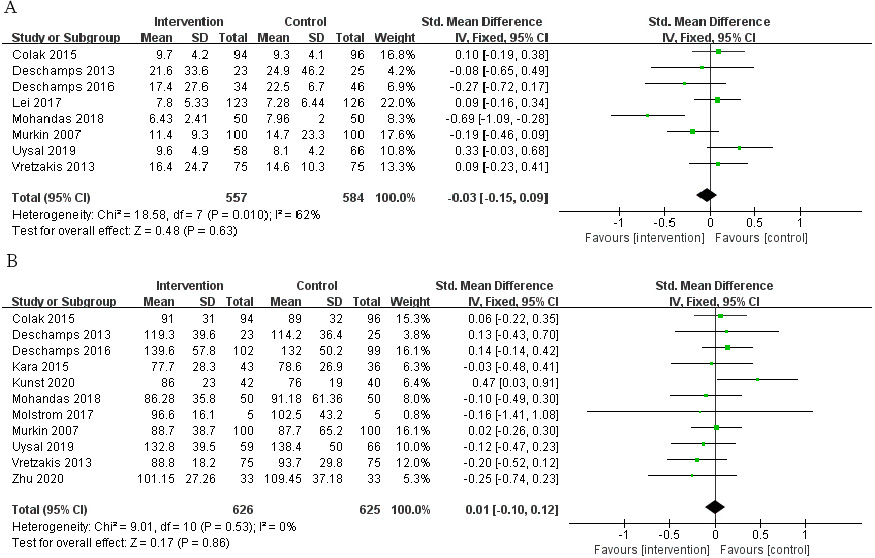
The pooled effect of (A) mechanical ventilation duration. (B) Cardiopulmonary bypass (CPB) time.
We speculated that the year of trial publication year (Fig. 7), the age of the patients (Fig. 8), and the type of surgery (Fig. 9) might be sources of heterogeneity and performed subgroup analyses. Results show lower risk of POCD in people younger than 60 years old.
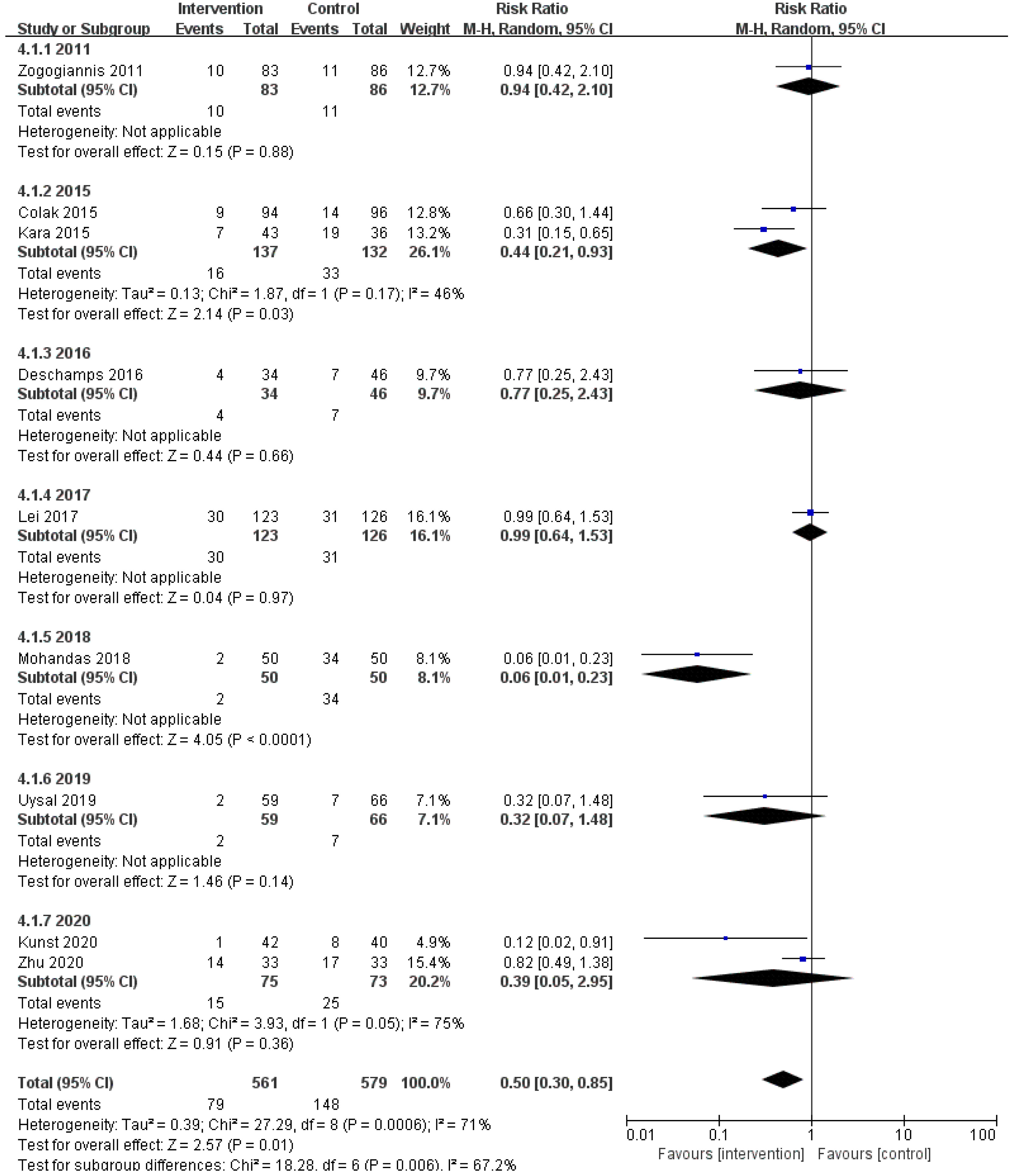
Subgroup analysis of different publication year groups.
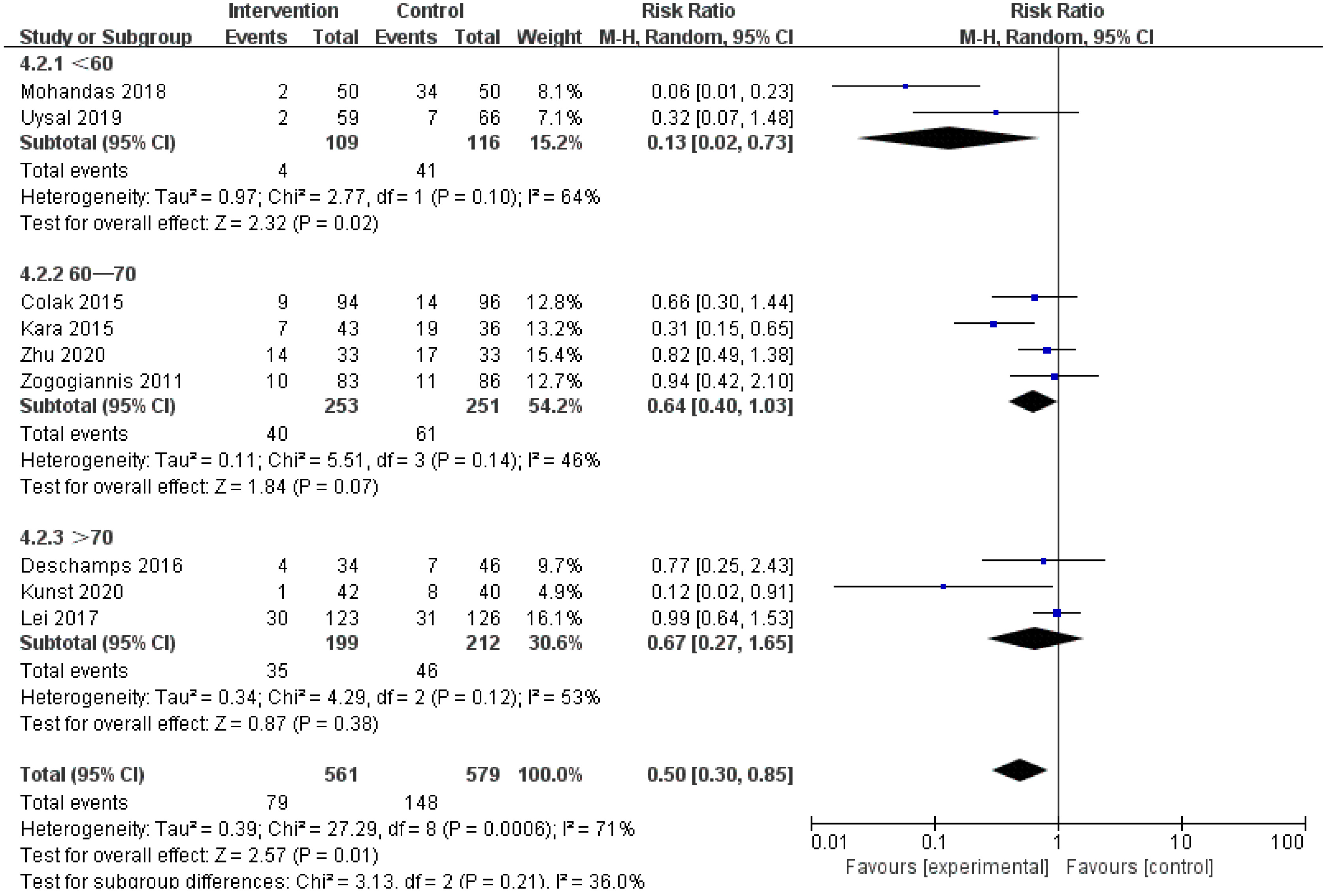
Subgroup analysis of different age groups.
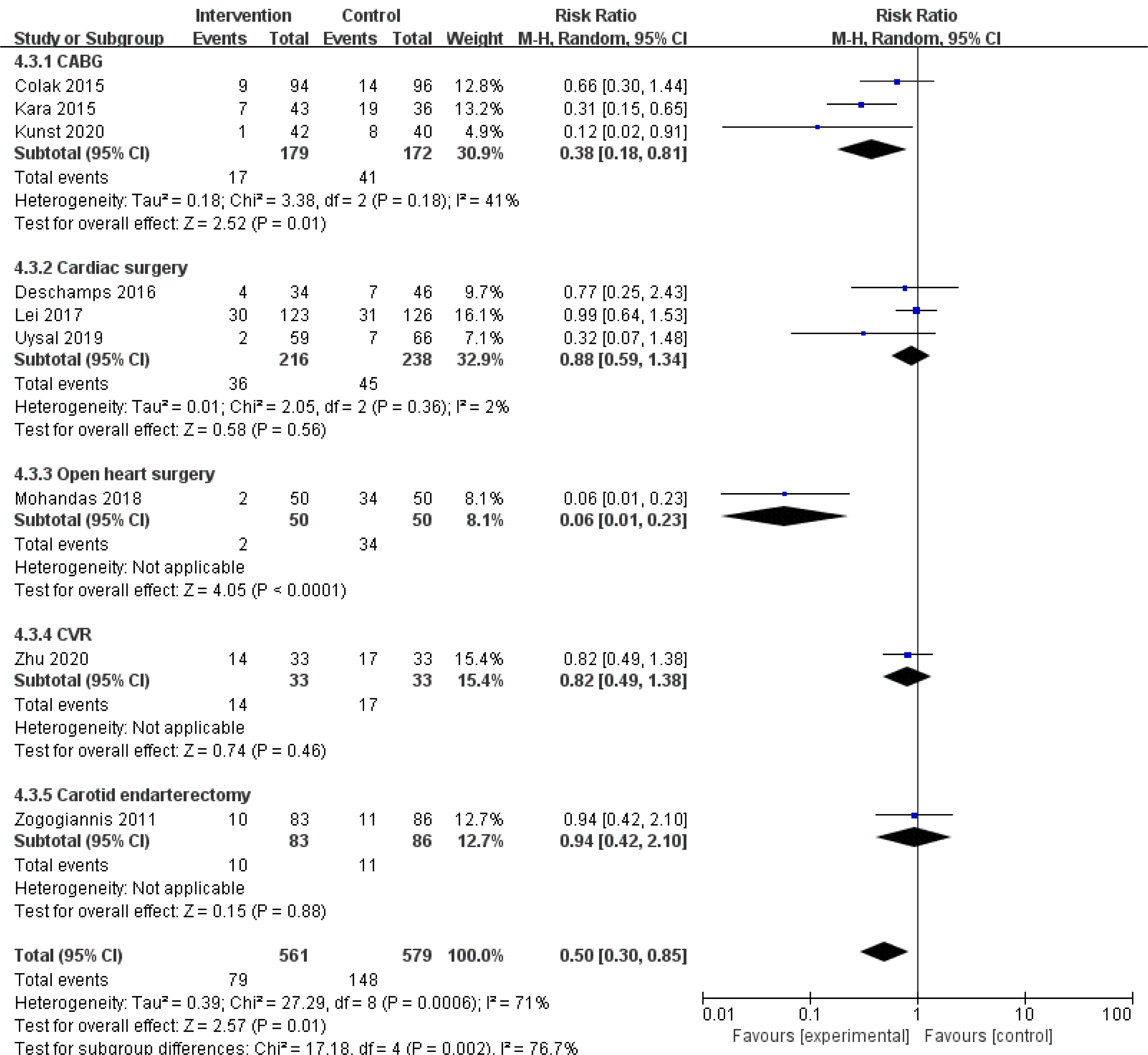
Subgroup analysis of different surgery types groups.
In the random-effect univariate meta-regression analysis of POCD incidence,
variables such as year of publication, age, male, past myocardial infarction,
diabetes, hypertension, cerebrovascular accident, chronic obstructive pulmonary
disease (COPD), European cardiac operative risk evaluation system, and baseline
left ventricular ejection fraction were considered. The main source of
heterogeneity in the incidence of POCD is age (coefficient = 0.06; p =
0.01; adjusted R
The funnel plot of POCD incidence has no obvious asymmetry. Results also showed
that there was no apparent publication bias in POCD incidence (Begg’s p
= 0.08) and ICU time (Begg’s p = 0.54). For other outcomes, due to the
small number of included studies, no begger test or egger test was performed, but
there was no obvious asymmetry in the funnel plot. After excluding the studies of
Lei et al. [21] and Mohandas et al. [11], I
POCD has a higher incidence after cardiovascular surgery, and it is more common in elderly patients. POCD is closely related to the long-term mortality rate after surgery. The symptoms are impairment of memory, orientation and abstract thinking of patients, as well as the ability to engage in social activities after anesthesia [28]. However, the specific mechanism of its occurrence is still unclear. Therefore, our meta-analysis comprehensively and systematically reviewed available randomized controlled trials investigating the relationship between cerebral oxygen saturation and POCD to evaluate the impact of intraoperative cerebral oxygen saturation-guided treatment on selected postoperative outcomes. The major findings of this study were that patients undergoing cardiovascular surgery who had brain oxygen saturation levels measured and maintained at high levels had a significantly lower risk of postoperative POCD than those who did not have brain oxygen saturation measurements and interventions. In addition, both cerebral oxygen testing and intervention had positive, but not statistically significant outcomes in terms of infection, hospital mortality, and stroke. We also found that patients with cardiovascular surgery who underwent intraoperative cerebral oxygen detection and intervention had a shorter ICU stay after surgery, but there was no difference in hospital stay. There was no significant difference in CPB time, mechanical ventilation time and other postoperative complications like renal failure and arrhythmia.
Previous studies have shown that there is a significant correlation between the
severity of the reduction of intraoperative rScO
ICU time and hospital stay can reflect the recovery of patients after surgery,
of which ICU time is more meaningful. Patients with poor prognosis will
inevitably have prolonged ICU time and hospital stay. Therefore, we used ICU time
and hospital stay as secondary outcomes to evaluate whether intraoperative
cerebral oxygen saturation monitoring and intervention are more beneficial to
patient prognosis. Adams et al. [23] conducted a randomized controlled
study of 200 patients undergoing coronary artery bypass grafting, and found that
the lower the basal and mean values of rScO
We initially speculated that the shorter CPB time and mechanical ventilation time could reflect the length of the operation to a certain extent, and based on this, we could evaluate whether the monitoring and intervention of intraoperative cerebral oxygen saturation were beneficial to the smooth progress of the operation. However, our results showed no significant difference between the intervention and control groups. Perhaps there is no association, so we need larger multicenter studies to verify the relationship. We also selected some common postoperative complications to verify whether intraoperative cerebral oxygen saturation monitoring and intervention would reduce the risk of postoperative complications in patients. Studies have shown that changes in cerebral oxygen saturation may be associated with postoperative renal insufficiency due to the relationship between low rSo2 values and impaired systemic tissue perfusion. This is why we chose renal failure as one of the outcomes [23, 33]. Infection is one of the common complications of various surgeries, so we were interested in exploring whether intraoperative changes in cerebral oxygen saturation were associated with the risk of postoperative infection. In addition, arrhythmia and hospital mortality can be used to assess the prognosis of patients. Stroke, as a neurological complication, can be specifically used to evaluate the postoperative neurological condition of patients. Although we found a lower risk of infection, death, and stroke in the intervention group than in the control group, these data were not statistically significant. This may be related to the size of the sample size.
At present, the pathogenesis of POCD is not clear. Studies have found that old age, years of education, preoperative comorbidities, length of anesthesia, types of surgery, preoperative medication, and postoperative infection are all risk factors for POCD [34, 35, 36]. The mechanism of intraoperative cerebral oxygen saturation monitoring and POCD reduction remains unclear, and it may be the result of multiple factors. Cardiovascular surgery is a kind of operation with a relatively long time and large trauma and high incidence of perioperative stroke. Cerebrovascular microembolism caused by plaque removal caused by surgical operation, temporary intraoperative artery blockade, and postoperative wound inflammatory reaction will all lead to arterial pressure deficiency, reduced effective cerebral perfusion, limited nerve cell function, and further lead to brain regulatory dysfunction [37]. We speculate that systemic inflammatory factors will be produced in the human body during the perioperative period, which will destroy the blood-brain barrier, and promote the infiltration of inflammatory factors and macrophages into the brain, resulting in impaired brain function. Preoperative use of dexamethasone has been found to reduce the inflammatory response, thereby reducing the risk of early POCD after cardiac surgery [13, 38]. Maintaining a high level of cerebral oxygen means that normal cerebral perfusion pressure ensures stable blood flow to the brain, thereby ensuring a continuous supply of oxygen and energy to improve cerebral vascular embolism, insufficient cerebral perfusion, tissue hypoxia, and inflammatory reactions. Oxygen metabolism imbalance thereby reducing the incidence of POCD [39]. However, the specific mechanism remains unclear, which is the direction that needs to be studied in the future.
Our study systematically investigated the impact of intraoperative cerebral
oxygen saturation monitoring and intervention on cardiovascular surgery patients
from intraoperative process to prognosis. It provides new directions and guidance
for future research in this field. We also believe that monitoring cerebral blood
perfusion during cardiac surgery is particularly necessary to reduce
postoperative neurological complications, especially for elderly patients, the
use of rScO
Our meta-analysis has several limitations: First, the included studies are quite heterogeneous, and the reason may be that the source of the cases is elderly patients, and their postoperative cognitive function is affected by many factors such as diversification of surgical methods, combination of different underlying diseases, selection of different anesthetics, and so on. Due to the limited number of included literature, it is not yet possible to conduct subgroup analysis to discuss the results of different periods of postoperative POCD. Although the results of this study indicate that there are differences in the impact of intraoperative detection and intervention of cerebral oxygen saturation on patients’ cognitive impairment after surgery, there is currently a lack of large-sample and high-quality randomized controlled studies. The results of this research can provide clues and a reference basis for the design of the next research. Second, the time to evaluate POCD was relatively short (i.e., one week postoperatively), which may restrict the clinical significance of our results. However, it is worth noting that only two trials evaluated this result after three months, and both trials showed a significant reduction in POCD in the intervention group. Third, the sample size of the included randomized controlled trials is small. The lack of a sufficient number of patients to detect a meaningful difference emphasizes the necessity of further massive randomized trials.
In conclusion, we conclude that maintaining intraoperative cerebral oxygen saturation at a high or stable state can significantly reduce the risk of postoperative POCD in patients with cardiovascular surgery, and can shorten the patient’s ICU time, which has a positive effect on the prognosis of the patient.
XS and LW conceived and designed this study. LW and ZL analyzed data, prepared figures, as well as prepared and edited the manuscript. HG and HD performed statistical analyses. LW, YL, and XS wrote and revised manuscript. All authors reviewed the final manuscript.
The authors are accountable for all aspects of the work in ensuring that questions related to the accuracy or integrity of any part of the work are appropriately investigated and resolved. The study was conducted in accordance with the Declaration of Helsinki (as revised in 2013). This study protocol was approved by the Institutional Ethics Committee of Fuwai Hospital (No. 2018-1069).
Not applicable.
This study was supported by Beijing Municipal Science and Technology Commission, China, Major Special Project #Z181100001718197.
The authors declare no conflict of interest.
Publisher’s Note: IMR Press stays neutral with regard to jurisdictional claims in published maps and institutional affiliations.