Academic Editor: Hack-Lyoung Kim
Subclinical hypothyroidism (SH) is associated with hemodynamic and metabolic
abnormalities that cause endothelial dysfunction and atherosclerotic
cardiovascular diseases. Aortic velocity propagation (AVP), epicardial fat
thickness (EFT), and carotid intima-media thickness (CIMT) may provide additional
information in SH patients.
This study aimed to evaluate thyroid stimulating hormone (TSH), AVP, EFT, and
CIMT in SH patients, and determine the associations among these parameters.
Eighty patients with SH and 43 euthyroid (EU) individuals were enrolled. Blood
samples were collected to measure laboratory parameters. Patients were divided
into two groups based on their TSH values (TSH
Subclinical hypothyroidism (SH) is defined as elevated levels of thyroid-stimulating hormone (TSH) and normal levels of free thyroid hormones. SH affects 4–10% of the population [1]. SH is associated with hemodynamic and metabolic abnormalities, which increase the risk for atherosclerotic cardiovascular diseases [2] and endothelial dysfunction [3, 4]. Decreased thyroid hormones are associated with increased arterial stiffness and systemic vascular resistance [5]. SH patients have lower aortic distensibility and higher aortic stiffness index than euthyroid (EU) individuals [6]. Masaki et al. [7] demonstrated that the cardio-ankle vascular index was increased in SH patients, suggesting that increased arterial stiffness may be associated with cardiovascular diseases. Moreover, TSH levels were inversely correlated with endothelium-dependent dilatation [8]. Aortic velocity propagation (AVP) is a recently described echocardiography-based measure that reflects aortic stiffness. AVP was negatively associated with coronary and carotid atherosclerosis [9].
Epicardial adipose tissue (EAT) is found between the pericardial visceral layer
and myocardium, surrounding the coronary arteries. An increase in EAT is
positively associated with coronary artery disease (CAD) [10]. Cytokines released
from the EAT are involved in causing atherosclerosis [11]. Previous studies have
demonstrated that epicardial fat thickness (EFT) and TSH values are significantly
related to SH. Higher EFT values have been reported in SH patients compared to EU
individuals [12]. A recent study found that EFT was significantly increased in SH
patients with a TSH level
Carotid intima-media thickness (CIMT) is a marker of subclinical atherosclerosis
and was recently found to be associated with CAD [14]. However, the effect of SH
on CIMT values is not clear [15, 16]. A recent meta-analysis based on eight
studies and 3602 patients demonstrated that the CIMT values were higher in SH
patients with TSH
AVP, EFT, and CIMT measurements may provide information on subclinical atherosclerosis in SH patients. We hypothesized that TSH may be related to AVP, EFT, and CIMT in SH patients. This study aimed to investigate the associations between TSH, AVP, EFT, and CIMT in SH patients. To the best of our knowledge, this is the first study to evaluate the association of AVP, EFT, and CIMT measurements with subclinical atherosclerosis in SH patients.
This cross-sectional study included 80 SH patients and 43 controls (matched by
age and sex) who presented to our clinic between May and August 2020. SH was
diagnosed on the basis of TSH levels
Patient evaluation included physical examinations (including anthropometric
measurements), medical history, and basic laboratory tests. Following a fast of
Color M-mode Doppler recordings were obtained using the suprasternal window in supine position. The cursor was placed parallel to the direction of main flow in the descending aorta. The Nyquist limit was 30–50 cm/s, and a recorder sweep rate of 200 mm/s was used during M-mode. AVP was defined as the velocity of flow in the artery, calculated by dividing the distance between the points corresponding to the beginning and end of the propagation slope by the duration between the corresponding time points. At least three measurements were obtained, and their mean was recorded as the AVP (Fig. 1).
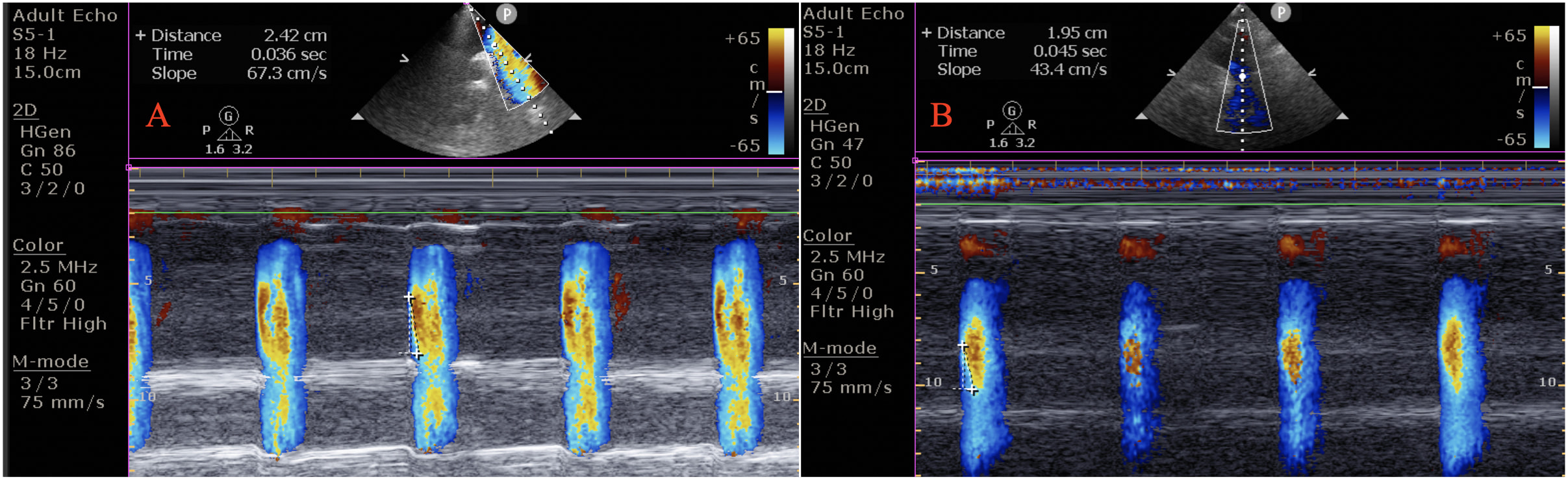
Aortic velocity propagation (AVP) in an euthyroid subjects (AVP = 67.3 cm/s) (A), and in a patient with subclinical hypothyroidism (AVP = 43.4 cm/s) (B). The AVP was calculated by dividing the distance between points corresponding to the beginning and end of the propagation slope by the duration between corresponding time points.
EFT was measured for the right ventricle’s free wall using the parasternal long-axis view with aortic annulus as the anatomic reference. EFT was measured during the end-systolic period. Epicardial fat was identified as the space without an echogenic view between the visceral pericardium and myocardium. EFT was measured at the thickest area, preferably the right supraventricular area (Fig. 2).
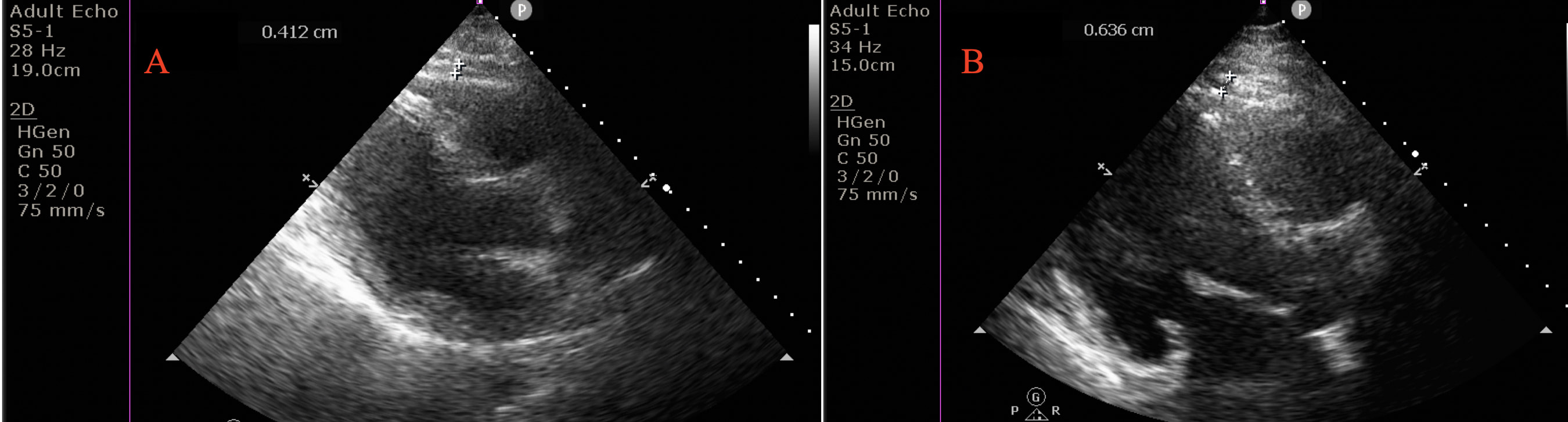
Measurement of epicardial fat thickness (EFT) in an euthyroid subjects (EFT = 0.4 cm) (A), and in a patient with subclinical hypothyroidism (EFT = 0.6 cm) (B). Epicardial fat thickness (EFT) perpendicular to right ventricular free wall. EFT identified as an echo-free space between the myocardium and visceral pericardium from the parasternal long-axis view on two-dimensional echocardiography, was measured perpendicularly in front of the right ventricular free wall at end-diastole.
Both carotid arteries were analyzed at plaque-free sites. CIMT was defined as the interval between the lumen-intima and the media-adventitia borders at the far wall 1–2 cm away from the common carotid artery bifurcation (Fig. 3) [18].
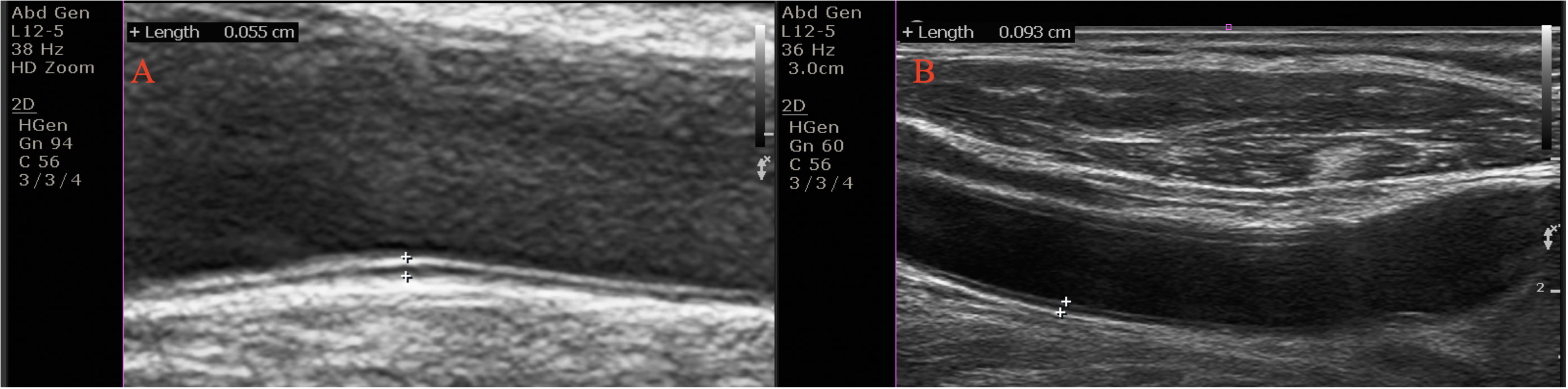
Carotid intima-media thickness (CIMT) in an euthyroid subjects (CIMT = 0.5 mm) (A), and in a patient with subclinical hypothyroidism (CIMT = 0.6 mm) (B). The CIMT was computed on the far wall at 1–2 cm from the common carotid artery’s bifurcation as the interval between the lumen-intima and the media-adventitia borders.
Data were analyzed with SPSS Statistics software (version 25.0 for Windows; IBM
Corp., Armonk, NY, USA). The Kolmogorov-Smirnov test was used to confirm the
normal distribution of continuous variables. Normally distributed variables were
expressed as means
Table 1 summarizes the demographic and laboratory characteristics of the study
population. There were no differences between the groups in terms of age, sex,
body mass index (p
Control (n = 43) | Patients (n = 80) | p value | |
Age (years) | 46.7 |
44.0 |
0.22 |
Body mass index (kg/m |
26.2 |
26.5 |
0.51 |
Male (%) | 27 (63) | 36 (45) | 0.06 |
White blood cell count (10 |
7.9 |
7.2 |
0.10 |
Hemoglobin (g/dL) | 14.5 |
14.8 |
0.12 |
Platelet count (10 |
249.3 |
235.3 |
0.32 |
Creatinine (mg/dL) | 0.7 |
0.7 |
0.89 |
Sodium (mEq/L) | 137.3 |
137.0 |
0.65 |
albumin (g/dL) | 3.5 |
3.8 |
0.11 |
C-reactive protein (mg/L) | 6.9 |
6.5 |
0.16 |
Triglyceride (mg/dL) | 205.2 |
201.9 |
0.42 |
High density lipoprotein (mg/dL) | 42.3 |
44.7 |
0.12 |
Low density lipoprotein (mg/dL) | 119.2 |
118.6 |
0.92 |
Total cholesterol (mg/dL) | 196.7 |
187.0 |
0.15 |
Ejection fraction (%) | 60.8 |
58.9 |
0.06 |
AVP (cm/s) | 62.6 |
43.7 |
|
EFT (mm) | 0.6 |
0.7 |
0.10 |
CIMT (mm) | 0.5 |
0.8 |
|
T4 (µg/dL) | 1.3 |
1.4 |
|
TSH (mIU/L) | 3.1 |
14.2 |
|
AVP, aortic velocity propagation; EFT, Epicardial fat thickness; CIMT, carotid
intima-media thickness; T4, Thyroxine 4; TSH, Thyroid stimulant hormone.
Differences between the two groups were evaluated using Unpaired t-test
for parameters with a normal distribution. p |
SH patients were divided into two groups based on their TSH values and compared
with the control group (Table 2). AVP values were significantly lower in SH
patients with TSH
Control (n = 43) | TSH |
TSH |
p value | |
Age (years) | 46.7 |
46.4 |
42.2 |
0.13 |
Body mass index (kg/m |
26.2 |
26.6 |
26.4 |
0.71 |
White blood cell count (10 |
7.9 |
6.7 |
7.7 |
0.02 |
Hemoglobin (g/dL) | 14.5 |
14.1 |
14.6 |
0.24 |
Platelet count (10 |
249.3 |
244.4 |
228.2 |
0.38 |
Creatinine (mg/dL) | 0.7 |
0.7 |
0.7 |
0.64 |
Sodium (mEq/L) | 137.3 |
137.5 |
136.6 |
0.46 |
albumin (g/dL) | 3.5 |
3.8 |
3.8 |
0.09 |
C-reactive protein (mg/L) | 6.9 |
6.3 |
6.6 |
0.12 |
Triglyceride (mg/dl) | 205.2 |
191.9 |
209.6 |
0.59 |
High density lipoprotein (mg/dL) | 42.3 |
43.6 |
45.6 |
0.18 |
Low density lipoprotein (mg/dL) | 119.2 |
118.9 |
118.4 |
0.99 |
Total cholesterol (mg/dL) | 196.7 |
190.5 |
184.4 |
0.24 |
Ejection fraction (%) | 60.8 |
60.1 |
58.0 |
0.02 |
AVP (cm/s) | 62.6 |
46.7 |
41.4 |
|
EFT (mm) | 0.6 |
0.7 |
0.7 |
0.16 |
CIMT (mm) | 0.5 |
0.8 |
0.7 |
|
T4 (µg/dL) | 1.3 |
1.4 |
1.4 |
0.001 |
TSH (mIU/L) | 3.1 |
7.2 |
19.6 |
|
AVP, aortic velocity propagation; EFT, Epicardial fat thickness; CIMT, carotid
intima-media thickness; T4, Thyroxine 4; TSH, Thyroid stimulant hormone. |
Table 3 summarizes the correlation between TSH and clinical parameters. TSH
levels had a weak positive correlation with EFT (r = 0.188; p = 0.038)
and CIMT (r = 0.236; p = 0.009) values (Fig. 4). However, AVP had a
significant negative correlation with TSH levels (r = –0.424; p
AVP | r | –0.424 |
p | ||
EFT | r | 0.188 |
p | 0.038 | |
CIMT | r | 0.236 |
p | 0.009 | |
TC | r | –0.126 |
p | 0.16 | |
LDL | r | 0.067 |
p | 0.46 | |
TSH, Thyroid stimulant hormone; AVP, aortic velocity propagation; EFT, Epicardial fat thickness; CIMT, carotid intima-media thickness; TC, Total cholesterol; LDL, Low density lipoprotein. |
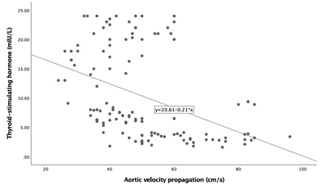
Correlation between Thyroid stimulating hormone and Aortic
velocity propagation (AVP) (r = –0.424, p
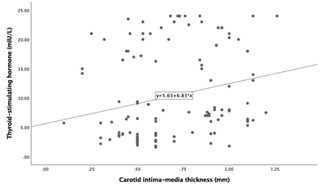
Correlation between Thyroid stimulating hormone and carotid intima-media thickness (r = 0.236, p = 0.009).
There was no significant correlation of TSH levels with TC or LDL levels
(p
B | t | p | 95% CI | |
TSH | –0.298 | –3.702 | –0.946–(–)0.287 | |
CRP | 0.078 | 0.996 | 0.321 | –2.003 |
TC | 0.128 | 1.628 | 0.106 | –0.144 |
TSH, Thyroid stimulant hormone; CRP, C-reactive protein; TC, Total cholesterol.
B, Regression coefficient; t, Degree of freedom; CI, confidence interval.
p |
ICCs for intra- and inter-observer reliability for AVP among 15 randomly selected patients were 0.92 (95% CI = 0.86–0.95) and 0.88 (95% CI = 0.82–0.94), respectively. ICCs for intra- and inter-observer reliability for EFT were 0.92 (95% CI = 0.85–0.94) and 0.90 (95% CI = 0.84–0.96), respectively.
This study investigated the AVP, EFT, and CIMT values in SH patients and EU individuals. AVP was lower in SH patients compared to the control group, and AVP decreased significantly with an increase in TSH levels. SH patients had significantly higher CIMT values compared to EU individuals. In addition, TSH levels had a significant negative correlation with AVP as well as a positive correlation with CIMT and EFT. TSH levels were independently associated with AVP. These findings may explain the pathological mechanisms of cardiovascular diseases in SH patients.
AVP is a parameter for aortic stiffness that can be measured during echocardiography. AVP had a significant correlation with aortic strain and distensibility in CAD patients [19]. Many studies have demonstrated increased vascular stiffness in patients with SH. However, a number of other studies did not find such an association.
Different mechanisms may be responsible for the change in arterial wall properties in SH patients. These mechanisms include endothelial dysfunction, increased angiotensin receptor expression, inflammation, and dyslipidemia [4, 20, 21]. In our study, SH patients had greater aortic stiffness than EU individuals. Moreover, AVP had a significant negative correlation with TSH levels, probably because TSH directly affects the arterial wall. We assumed that high TSH values may predispose SH patients to endothelial dysfunction and increased angiotensin receptor expression, leading to decreased blood velocity in the descending aorta. Previous studies have reported that TSH levels are negatively associated with endothelium-dependent dilatation [22]. Dardano et al. [20] reported that recombinant human TSH reduced vascular nitric oxide levels and induced inflammation leading to endothelium-dependent vasodilation in patients with thyroid disorders. TSH levels affect the aortic wall tension in SH patients. Yurtdaş et al. [6] demonstrated that SH patients had higher aortic stiffness index, lower aortic distensibility, and reduced systolic aortic velocity compared to the control group.
Previous studies reported that SH patients had higher brachial-ankle pulse wave velocity, cardio-ankle vascular index, and flow-mediated dilatation compared to EU individuals [7, 23, 24]. Tian et al. [21] demonstrated that SH was associated with increased carotid arterial stiffness, likely to be due to increased high-sensitivity CRP levels. Arterial wall thickness, arterial stiffness, and endothelial dysfunction in SH patients was associated with increased cardiovascular risk [25]. Itterman et al. [26] reported that hypothyroidism (clinical or subclinical) was associated with atherosclerosis. Additionally, thoracic aortic wall thickness increased with increasing levels of TSH. However, Nagasaki et al. [23] did not find a correlation of brachial-ankle pulse wave velocity with TSH, T3, or T4 levels. Another study found normal arterial stiffness in SH patients [27]. The brachial-ankle pulse wave velocity was decreased in SH patients treated with thyroid hormone replacement [28]. In addition, thyroid hormone replacement reduces atherosclerosis by its direct effect on the blood vessels in SH patients [29]. This study demonstrated that AVP could be used to determine aortic stiffness in SH patients. In the regression analysis, TSH levels were independently associated with AVP in SH patients. We assumed that higher TSH levels caused endothelial dysfunction and increased arterial stiffness. Therefore, AVP decreased significantly with an increase in TSH values. Decreased AVP may indicate atherosclerosis in SH patients, and AVP decreases prior to clinically apparent vascular diseases.
EAT induces paracrine and vasocrine secretion of pro-inflammatory and
pro-atherogenic cytokines into the myocardium that affect the cardiac function
[11]. EAT may also contribute to the pathogenesis and development of CAD due to
the production of several inflammatory adipokines [30]. Besides, the mechanical
effects of EAT may also contribute to the development of atherosclerosis [31]. A
previous study reported that there was increased TSH receptor expression in EAT,
which may affect the cardiac function and pathology [32]. The relationships
between EFT and TSH levels in SH patients are controversial. Korkmaz et
al. [12] demonstrated the EFT values in 61 newly diagnosed SH patients and 24
controls. SH patients had higher EFT values that correlated with the TSH levels.
Additionally, SH patients with TSH
CIMT can be safely measured using ultrasound images, and it may indicate
subclinical atherosclerosis. Carotid plaques are an early surrogate marker of
systemic atherosclerosis and predict major cardiovascular events [35].
Hypothyroidism is associated with accelerated atherosclerosis. A previous study
showed that CIMT was an objective sign of accelerated atherosclerosis in patients
with primary hypothyroidism [36]. Saif et al. [37] demonstrated that
CIMT was higher in overt hypothyroidism and SH patients compared to the controls.
Additionally, endothelial dysfunction was associated with increased CIMT values
in patients with SH or overt hypothyroidism [37]. A recent meta-analysis reported
similar CIMT values in SH patients with TSH
Other studies did not report any significant association between SH and CIMT.
Almeida et al. demonstrated that CIMT values were similar between SH patients
with TSH
There were a few limitations to this study. This was a single-center study based on a relatively small group of patients. The sample size may not be large enough to generalize the results of this study. Women are more prone to thyroid diseases than men. There were more female patients in the SH group than the control group. However, this difference was not statistically significant (p = 0.06). This difference in the sex ratio may explain the absence of sex differences in our study. We did not evaluate the autoimmune antibodies, such as anti-thyroglobulin antibody or anti-TPO-Ab, or their relationship with aortic stiffness. Pulse wave velocity is the gold standard for measuring arterial stiffness. However, we did not measure it in our study. We did not use magnetic resonance imaging or computed tomography to measure EFT. However, the use of echocardiography for the measurement of EFT is less costly and widely accepted. TC levels were similar between the study groups, probably because of the small number of patients and unknown duration of SH. A large randomized clinical trial is needed to validate these findings.
In conclusion, we found that AVP was lower and CIMT was higher in SH patients compared to EU individuals. Different mechanisms may be responsible for the role of TSH in aortic stiffness and subclinical atherosclerosis in SH patients. These mechanisms should be investigated further. The present study suggested that AVP, EFT, and CIMT should be routinely measured in SH patients.
EA, RA—conception and design of the study, provision of study material and patients. EA, ZD, TA—data collection and analysis, provision of study material and patients. EA, ZD—study oversight and critical revision of the article, provision of study material and patients. EA, TA, RA—analysis and interpretation of data, drafting of the article.
All subjects gave their informed consent for inclusion before they participated in the study. The study was conducted in accordance with the Declaration of Helsinki, and the protocol was approved by the Ethics Committee of Van Training and Research Hospital (approval number: 2020/25).
We would like to express our gratitude to all those who helped us during the writing of this manuscript. Thanks to all the peer reviewers for their opinions and suggestions.
This study received no external funding.
The authors declare no conflict of interest.