Cardiovascular disease, and in particular coronary artery disease (CAD), remains an important contributor of morbidity and mortality among patients with chronic kidney disease (CKD). Classic symptomatology of CAD and effectiveness of established therapeutic measures is less frequent in patients with CKD. This suggests unique characteristics of CAD among patients with CKD. Two important features of CAD in CKD include increased calcific density of atherosclerotic plaques and of the vessels themselves (coronary artery calcification -- CAC), as well as a decrease in microcirculatory function -- or coronary microcirculatory dysfunction. A multitude of pathophysiologic pathways have been identified that contribute to CAC in CKD; less is known about the pathophysiology of microcirculatory dysfunction. It is not well established if these two processes are directly related to each other, but the combination results in a greater severity of effect on overall myocardial function and may in part explain the greater preponderance of silent myocardial infarction. Further investigation is needed to better understand these unique aspects of CAD in CKD as well as the role they play in overall CVD in this group, and ultimately therapeutics that may lessen the burden of disease.
For patients living with chronic kidney disease (CKD), the number one leading cause of morbidity and mortality remains cardiovascular disease (CVD), with some studies showing that greater than 50% of patients with end stage renal disease (ESRD) experience death secondary to cardiovascular disease (Go et al., 2004; Sarnak et al., 2019). Even when taking into account the traditional risk factors such as hypertension, diabetes, and hyperlipidemia, the mere presence of CKD worsens the prognosis of those with coronary artery disease (CAD) (Matsushita et al., 2015). Attempts have been made to analyze if risk reductions for traditional CAD risk factors have the same impact in CKD populations. Previous studies have shown that statin use for the reduction of low density lipoproteins does not improve rates of mortality or major adverse cardiovascular events (MACE) in ESRD (Fellstrom et al., 2009; Wanner et al., 2005). When looking at the spectrum of CKD patients, the use of a statin plus ezetimibe did reduce MACE but not vascular mortality, demonstrating that a reduction of vascular disease earlier in the disease process of CKD did have benefit (Baigent et al., 2011).
Beyond the increase in risk for vascular disease, patients with CKD and CAD present differently from CAD-only patients in terms of their clinical presentation and comorbidities. Typical presenting symptoms of CAD are less often seen in CKD patients, and CKD patients more often present with acute coronary syndromes (ACS) as their index of heart disease versus the more common stable ischemic heart disease presentations seen in non-CKD subjects (Sarnak et al., 2019). These peculiarities of CAD in patients with CKD suggest the presence of potentially unique specific pathophysiologic characteristics. Two specific features include extensive vascular calcification including coronary artery calcification (CAC) and coronary microvascular dysfunction. The following will review the available evidence on both CAC and coronary microvascular dysfunction as pathophysiologic mechanisms in this high-risk population of CAD and CKD.
Coronary artery calcification (CAC) is a phenomenon where cardiac vasculature undergoes plaque formation and calcification, thereby becoming less compliant and patent, impairing cardiac myocardial perfusion. Among the general population, CAC is heavily associated with age and gender. Patients greater than 70 years of age have been shown to have CAC in 90% of men and 67% of women (Madhavan et al., 2014). Within coronary arteries, the intimal and medial layers undergo calcification under different rates and likely represent different stages of the atherosclerotic process (McCullough et al., 2008a; McCullough et al., 2008b). CKD is among the disease states that develop both intimal and medial calcification in an accelerated manner.
Though CAC can occur in any stage in CKD, including early stages, it is most prevalent in dialysis and kidney transplant recipients. In a meta-analysis of the available studies examining prevalence and outcomes of CAC in CKD, the overall prevalence of CAC in all patients with CKD was shown to be 60% (Wang et al., 2019). A pooled prevalence of 65% was found amongst hemodialysis patients, with different studies ranging from 24% to as high as 93%. Renal transplantation patients in this meta -analysis were found to have a pooled prevalence of 51%, as compared with a 60% prevalence in peritoneal dialysis patients. The prevalence of medial calcification appears to be similar among patients with CKD as in the general population; however this may be a function of older age, i.e. in younger matched cohorts those on dialysis would have higher rates of medial calcification (Chin et al., 2017; Nakamura et al., 2009; Sanchis et al., 2019). Modern intravascular imaging modalities (Chin et al., 2017) will allow for greater delineation of characteristic calcification patterns in patients with CKD.
The process of CAC develops over the course of a patient's life, and in the absence of CKD tends to be a hallmark of aging. The traditional pathway to CAC is intimal calcification. Here atherosclerosis plaque formation and calcification take place through lipid deposition, inflammation, and ultimately osteogenic differentiation and mineralization. In CKD, there is an acceleration to the formation of intimal plaque due to increased inflammation and subsequent mineralization that can extend into the medial layer, making CKD an accelerator for atherosclerosis with calcification in (Chen and Moe, 2012; McCullough et al., 2008a,b).
Both medial and intimal calcification rely on many signaling factors and involvement of a number of cell types. In CKD, pro-calcification mediators are upregulated, while many inhibitory pathways and mediators are down regulated. For instance, with decreased glomerular filtration rate (GFR) there is an increase in plasma calcium-phosphate complexes with resulting secondary hyperparathyroidism (Chen and Moe, 2012). Fetuin-A is anti-inflammatory protein that regulates extracellular calcium and inhibits calcium and phosphate deposition. Fetuin-A decreases with CKD leading to a lack of anti-inflammatory factors and unregulated mineral deposition. Matrix Gla protein (MGP) is another inhibitor of medial calcification. These vitamin K dependent proteins are shown to be in a predominantly inert decarboxylated state in vitamin K deficiency. In models with knockdown MGP, mice developed complete CAC and are more like to die by 40 days (Roumeliotis et al., 2019). A higher prevalence of inactive MGP has been documented in ESRD (Westenfeld et al., 2012).
The membrane protein Klotho is an enzyme that prevents the conversion of VSMCs into secretory osteoblastic forms when there is high phosphate and calcium in the environment (Vervloet and Cozzolino, 2017). Klotho deficiency occurs in CKD, therefore creating a scenario where not only is mineral homeostasis worsening, but the protein responsible for defending against the development of an extracellular calcium deposition is deficient. The extracellular matrix created by the now osteochondrogenic form of VSMC enhances plaque calcification by attracting macrophages to contribute to plaque formation. The process of vascular calcification requires multiple cell types including macrophages. Instability is introduced into these vulnerable plaques via microcalcifications introduced into the fibrous cap of the plaque (Madhavan et al., 2014). A major driver of these calcification processes is calciprotein particles (CPPs): proteins bound to fetuin-A that are known to be increased in serum with decrease in renal function (Miura et al., 2018). As discussed above, when the homeostasis of phosphate and calcium is interrupted in renal dysfunction, MGP and fetuin-A levels decrease. With this decrease of anti-calcification mediators, the pro-calcification mediator CPP is increased, which leads to the conversion of VSMCs into their osteogenic forms that produce extracellular matrix and ultimately an unstable plaque (Ter Braake et al., 2019). Altogether CKD creates a perfect storm of increased signaling for pro-calcification, inhibition of anti-calcification pathways through inflammation and mineralization, and ultimately both intimal and medical calcification.
The Chronic Renal Insufficiency Cohort Study (CRIC) demonstrated that traditional risk factors in the general population that lead to CVD were also associated with CAC in CKD (obesity, hyperlipidemia, cigarette use, hypertension, and diabetes mellitus). The CRIC study showed that risk factors unique to CKD such as elevated serum calcium and phosphate levels, glomerular filtration rate (estimated with cystatin C levels) were independently associated with the presence of CAC (He et al., 2012).
Using an average follow-up of 5.9 years, the CRIC showed added benefit in the predictive capabilities of CAC in patients with CKD. In the past, the CAC score was found to be a superior predictor of CV morbidity/mortality in patients without CKD, in comparison to other non-invasive techniques such as ankle-brachial index and carotid intima-media thickness, but the CRIC study was able to show that in patients with mild to moderate CKD with a eGFR (20-70), CAC was strongly associated with CVD including MI, heart failure, and all-cause mortality in the non-HD CKD population. CAC was found to be a better predictor and risk stratification tool in patients with CKD than even ACC/AHA atherosclerotic cardiovascular risk factors (total cholesterol, HDL, systolic blood pressure, treatment for hypertension, cigarette smokers, diabetes) for predicting cerebrovascular disease, MI, heart failure, and all-cause mortality (Chen et al., 2017). Even when adjusting for hemoglobin A1c and BMI amongst others risk factors, a CAC score greater than 100 was associated with cardiovascular disease, MI, and heart failure. These studies cumulatively suggest that when it comes to monitoring non- dialysis CKD patients for occult CAD and need for interventions, whether lifestyle modification, pharmacologic or revascularization, CAC might be the superior risk determinant over standard risk calculators. If this conclusion is accurate, the need for accurate and quantifiable measurements in coronary calcium would be significant for these patients and their medical decision making.
Coronary computed tomography (CT) can detect the presence of CAC within thin-cut slices of imaging, with the extent of calcification being given a score with reference to percentiles of calcification based on the patient's age and gender. In addition to this, the density of the plaque is quantified and carries a weighted factor that increases the CAC score using the Agatson method (Bashir et al., 2015). The use of CT guided CAC scoring under the recommendations of the 2019 ACC/AHA primary prevention guidelines and the 2019 ESC guidelines for the diagnosis and management of CAD are as a supplementary risk assessment tool (Arnett et al., 2019; Knuuti et al., 2020). The challenge with the use of coronary CT determined CAC in patients with CKD is the elevated prevalence of high CAC scores at baseline in this population, thus limiting the discrimination for CVD events (Bashir et al., 2015).
On the other hand, the high prevalence might allow for strong negative predictive value. In patients undergoing review for kidney transplant, CACs combined with AHA/ACC risk factors (smoking, dyslipidemia, hypertension, LVH, and previous cardiovascular disease) was used to predict MACE separately and in conjunction, as well as with single photon emission computed tomography (SPECT) and invasive coronary angiography (ICA). The study found that low (less than 3) risk factors along with a CAC Agatson score less than 400 best identified low risk transplant patients, with only 2% of patients in that cohort showing stenosis by ICA (Rasmussen et al., 2018; Winther et al., 2018). Furthermore, CACs was a better predictor for all-cause mortality over ICA likely due to the combined effects of other factors often found in severe CKD patients as previously mentioned (inflammation, diabetes, etc.) in this high risk renal transplant population.
The treatment of patients with elevated CAC and whether reversal is possible or even beneficial is broad and has not produced clear goals for therapy. Small randomized trials have been able to show reduction in CAC through the use of calcium-channel blockers, non-calcium phosphate binders, calcimimetics (e.g. cinacalcet), and supplements such as garlic, B-vitamins, and L-arginine (Madhavan et al., 2014). Pharmacologic data on statins for CAC mitigation in CKD produced mixed results unlike the non-CKD population (Chen et al., 2017; Mitchell et al., 2018). More recently Raggi and colleagues demonstrated efficacy of a novel hydroxyapatite inhibitor (SNF472) in regression of CAC in patients on hemodialysis (Raggi et al., 2020). Further studies will be needed to evaluate long term efficacy on clinically relevant outcomes such as reduction in cardiovascular events and death.
Coronary microcirculation comprises arterioles less than 100 micrometer diameter whose role is to match aerobic consumption with perfusion to the myocardium (Camici and Crea, 2007). Coronary microvascular disease is thought to be a dynamic process, with adaptive changes occurring in response to a multitude of factors such as sheer stress on vessel walls, metabolic and inflammatory processes, and the response of myocardium to decreased oxygenation. Silent myocardial infarction and atypical angina are two presentations of heart disease often seen in CKD patients. Silent MI is a form of clinically relevant cardiac ischemia as seen by EKG that presents without angina or anginal equivalents (diaphoresis, dyspnea, jaw pain, etc.). Though traditionally correlated with diabetes mellitus due to that disease's effects on sensory innervation, studies have shown that patients with advanced renal disease awaiting transplantation have an increased prevalence of silent MI at 10.7% and was associated with an increased risk of cardiovascular mortality (Farag et al., 2017). Atypical angina on the other hand is the presentation of clinically relevant ischemia as seen by ECG and non-specific symptoms such as dyspnea or fatigue. This is a common presentation amongst patients with CKD (Sarnak et al., 2019). Microvascular angina, otherwise known as syndrome X, has been a commonly held hypothesis explaining the phenomenon of typical or atypical angina and presence of ST-segment changes in the absence of obstructive coronary disease or wall motion abnormalities. To explain this, studies have proposed that arteriolar dysfunction leads to scattered areas of focal ischemia throughout the myocardium (Camici and Crea, 2007). Microvascular angina has also been proposed as an under-recognized pathophysiological mechanism for heart failure with preserved ejection fraction (HFpEF), and CKD often co-exists due to their shared macrovascular and microvascular co-morbidities (Pries and Reglin, 2017). All of these observations has led researchers to the hypothesis that microvascular dysfunction could be the physiologic bridge that connects CKD patients to the increased rates of MACE that these patients are prone to experiencing.
Imaging for coronary microcirculatory dysfunction is a rapidly growing field. The most validated imaging modality for the evaluation of microcirculatory disease is positron emission tomography (PET). In this technique, a radiotracer such as N-ammonia or rubidium is used to monitor flow through the myocardium. One specific benefit of the PET is the ability to calculate coronary blood flow before and after administration of vasodilators such as Regadenason or Adenosine to achieve maximal hyperemic conditions. When taking the ratio of the myocardial blood flow (MBF) at rest and maximal hyperemia, we estimate the coronary flow reserve (CFR) (Camici and Crea, 2007). CFR is in this way used as a surrogate of microvascular function since the primary determinants are capillary density and patency. Therefore, in patients with normal coronary arteries as discussed above and as seen in CKD patients still experiencing MACE, CFR gives us insight into a possible etiology. Studies have shown in patients with hemodynamically non-significant coronary artery disease a discrepancy between the CFR and index of microcirculatory resistance (IMR). De Bruyne et al found that having a high IMR, which indicated resistance to arteriolar flow, and low CFR would predispose patients to poor outcomes, indicating that disruptions in microcirculation was clinically significant (De Bruyne et al., 2016).
The pathophysiology and the clinical significance of the deleterious effects of CKD on coronary microvasculature has been gradually elucidated. The first physiologic correlation made was that with the arterial stiffness created by CKD due to the numerous pathways discussed above, a resulting increase in pulse wave velocity, pressure, and flow leads to damage of capillary beds that constitute the microvascular circulation. This damage was found to exist not only in the kidney, brain, and other organs, but in the heart as well (Mitchell, 2008). Later studies were able to correlate the renal insufficiency to decreased MBF. By using PET/CT the research team realized that in patients with normal clinical myocardial perfusion, there was a correlation between renal dysfunction and microvascular dysfunction, as represented by an elevation in baseline (resting) MBF with a resultant minimal increase during stress (Fukushima et al., 2012). On the other hand, Bajaj and colleagues found no differences in resting MBF among patients with CKD, but did find a blunted increase in hyperemic MBF with resultant reduction in CFR estimate (Bajaj et al., 2020). In relation to clinical outcomes, both eGFR and CFR were most strongly associated with clinical cardiovascular outcomes (death, myocardial infarction, or hospitalization for heart failure), but in multivariate analysis including CFR and eGFR, only CFR remained as an important predictor of cardiovascular outcomes. This suggests that microvascular dysfunction, as estimated by CFR, may be along the causal pathway of reduced eGFR and incident cardiovascular events.
Therapeutic options for patients with symptomatic coronary microvascular dysfunction are limited and none have been explicitly tested in the CKD population. Recommended therapies include calcium channel blockers, beta blockers and nitrates (Knuuti et al., 2020; Ong et al., 2015). Novel therapies are under investigation and future trials should include subjects with CKD given the predominance of this type of coronary disease in this population. Given the cardiovascular benefits shown with the use of the newer antidiabetic agents such as the sodium glucose co transporter-2 inhibitors (SGLT2i) as well as the glucagon like peptide-1 receptor agonists (GLP-1Ras) with reduction in mortality risk, HF risk (predominantly with the SGLT2i). and reduction in MACE (predominantly with the GLP-1RAs), there may be additional options available that might expand the scope of therapies for patients with coronary microvascular dysfunction (Lo et al., 2020).
CKD represents a state of heightened cardiovascular risk, which involves the development of progressive ischemic heart disease. Unlike the general population, factors other than atherosclerotic epicardial coronary artery disease play an important role in the CKD population. This is manifest in the frequent presentation of atypical angina or silent myocardial ischemic events in this patient population. Factors that contribute to this include increased calcification of atherosclerotic plaques as well as medial calcification; and an impairment in the coronary microcirculatory function (Central figure). Existing and novel therapies are emerging as potential therapeutic modalities for mitigation of calcification of the coronary arterial system; no such therapies have been established yet for microcirculatory dysfunction. Future investigations and trials should focus on targeting these novel mechanisms of ischemic heart disease in CKD.
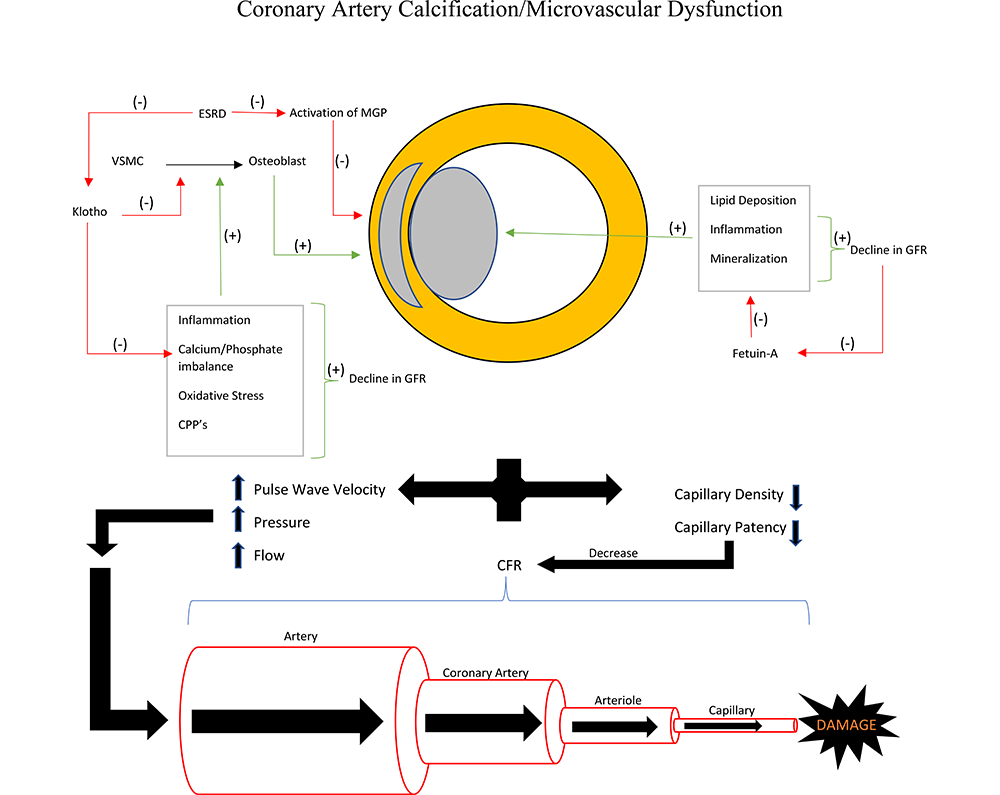
Central representation of coronary artery calcification and microvascular dysfunction in CKD.
Design and initial draft: MF, ROM
Critical Review and revisions: ROM, SB, MSS
Final Approval of Manuscript: MF, MSS, SB, ROM
The views expressed are that of the authors only and do not represent the views of the Federal Government or of the Department of Veterans Affairs.
The authors have no conflict of interest to declare.